Abstract
Keywords
1. Background
Industrial development and increasing dependence on energy for different elements of a development strategy requires extraction and transportation of hydrocarbons in sea, as well as land. Therefore, contamination of water bodies and soil due to oil spill is expected, and considering effective ways to minimize the pollution and removal contaminants from the environment is necessary (1, 2). Diesel, as a fuel, is a recalcitrant component that enters the environment by various pathways, and huge contamination would be resulted from different sources (3, 4). Exploring efficient physical, chemical, and biological treatment technologies is necessary for the reduction of environmental pollution due to diesel leakage in water and soil (5-7). Among different technologies, advanced oxidation processes (AOPS) are effective means for the degradation of recalcitrant constituent of diesel as a major environmental contaminant. The main mechanism of AOPS for destruction of pollutants is production of hydroxyl radicals (OH•), which reacts with target molecules rapidly and hardly and degrades them to harmless forms in selected conditions (8-10). Sonication of aqueous solutions is an attractive option that enhances the production rate of OH• radicals (11). The water molecules are broken into OH• radicals and hydrogen in sonication process. Consequently, the produced gas bubbles are destructed and released in water, which in turn results in high pressures and temperatures in solution. In sonication processes, free radicals such OH• and chemicals such as H2O2 participate in degradation of target organic pollutants (12). Recently, electrochemical-based oxidation has been widely studied for the treatment of highly contaminated streams. Immobilization of catalysts on the surface of the electrode is an interesting feature, which removes the need for external addition of catalyst (13). Safety, being environmentally friendly, cost-effectiveness, ease of operation, and high efficiency are advantages of electro-kinetic (EK) processes (14-18). According to literature review, this is the first report demonstrating the combination of EK oxidation and sonication for the treatment of diesel as the target pollutant in saline environment. The combination of sonication and EK oxidation to study the synergetic effects of these processes in the treatment of diesel contaminated solution was the main goal of the current work.
2. Methods
2.1. Chemicals
The analytical grade chemicals in current work, including sulfuric acid, hydroxide, N-hexane, methanol, and isopropanol (C3H8O), were purchased from Sigma-Aldrich.
2.2. Experimental Procedure
In this study, 250 mL of glass beaker was used as the reactor for performing the experiments. Graphite electrodes (150 mm long, 25 mm wide, and 5 mm thick) were placed directly in the solution. A DC power supply (Model: ZX 200) provided the current in the solution through connection to electrodes. The DC power apparatus was able to supply a voltage between 0 - 30 V cm–1. A sonication apparatus (Model: LUC-405) with frequency of 40 KHz was used to provide ultrasonic sound waves through direct insertion of the probe in the solution. H2SO4 (0.5 N) and NaOH (0.5 N) were used to adjust the pH to the desired level. Then, diesel containing wastewater was poured into the reactor at the beginning of each experiment. Effective operational variables including voltage between 0.5 - 3 V cm-1, sonication intensity between 100-300 W, contact time between 30 - 150 min, and pH in the range of 3 - 9 were investigated using one factor at the time of designing the experiment.
2.3. Analytical Methods
After sampling at the determined time intervals, the diesel concentration was measured as total petroleum hydrocarbons (TPH) with a gas chromatography (GC) apparatus using a flame ionization detector (FID) (Model: CHROMPAK CP 9001). The GC apparatus was equipped with a HP-5 capillary column (length: 30 m, inner diameter: 0.32 mm, film thickness: 0.2 mm) (19).
3. Results and Discussion
3.1. Raw Wastewater Characteristics
The composition of raw wastewater is presented in Table 1. As can be seen, the recalcitrant nature of wastewater is proved.
Characteristic of Oily Wastewater
Parameter | Mean | STDEV | Max | Min |
---|---|---|---|---|
COD (mg/L) | 3500 | 180 | 4100 | 3250 |
TPH (mg/L) | 2250 | 300 | 1980 | 2600 |
TDS (mg/L) | 13500 | 410 | 17280 | 11700 |
TSS (mg/L) | 480 | 112 | 640 | 302 |
pH | 7.7 | 0.81 | 8.3 | 7.3 |
3.2. Effect of pH
The solution pH is a key parameter in EK oxidation due to direct role in the rate and kind of oxidizing agent production. According to Figure 1, the highest removal rates of 44% and 40% were observed at pH values of 3 and 5, which was in line with literature that acidic pH better support the oxidation process (20, 21).
Effect of pH variations on TPH removal by sonication enhanced EK oxidation (Voltage = 0.5 V/cm, TPH = 2100 mg/L, reaction time = 60 min).
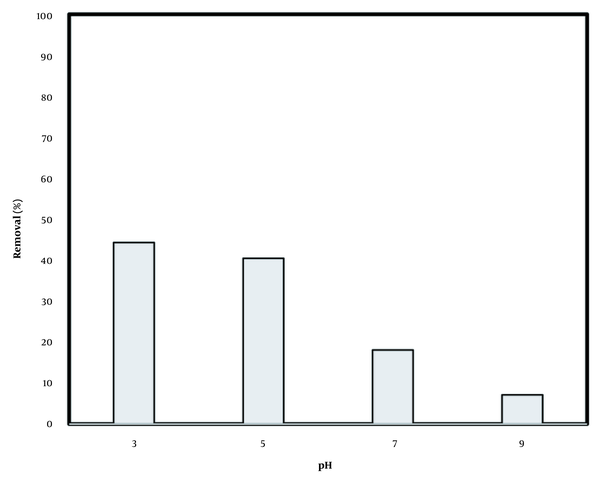
3.3. Effect of Voltage
Oxidation rate is usually enhanced directly along with voltage. The TPH removal varied between 40% and 65% at voltage range of 0.5 to 3 V/cm (Figure 2). There was no significant difference in results of voltage of 2.5 and 3 V/cm. Higher voltage levels provided higher current density in the solution and between the electrodes; therefore, the reactions proceeded more effectively and rapidly (22-24).
Effect of voltage variation on TPH removal Rate by sonication enhanced EK oxidation (pH = 5, TPH = 2000 mg/L, reaction time = 60 min).
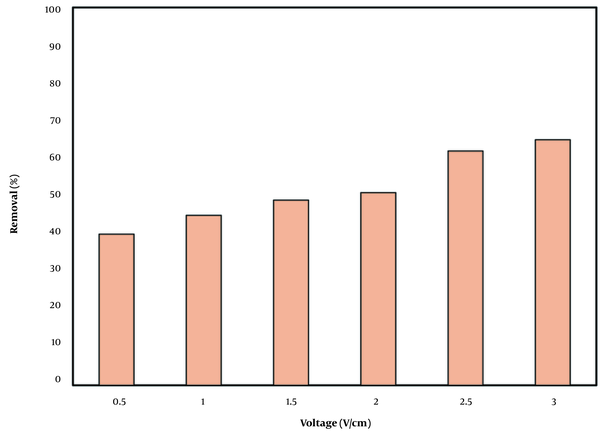
3.4. Effects of US Power
Effects of sonication are presented in Figure 3. Increasing the sonication power to 200 and 300 W, improved the removal rate up to 71% at a contact time of 60 min. Therefore, the sonication level of 200 W was selected due to lower energy consumption. Jiang et al. (25) also reported the direct relation between sonication power and removal.
Effects of variations of sonication intensity on TPH removal rate by sonication enhanced EK oxidation (pH = 5, V = 2.5 v, TPH = 1800 mg/L, reaction time = 60 min).
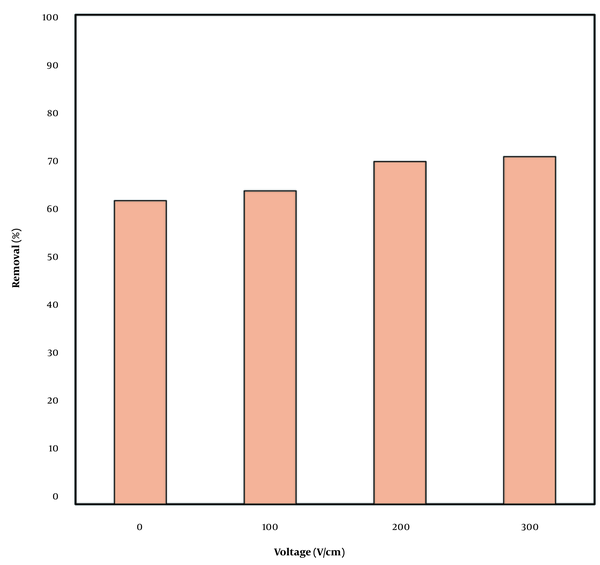
3.5. Effect of Reaction Time
Figure 4 shows the variations of contact time versus the rate of TPH removal. The removal rate of 71% enhanced to 91% at the contact time 150 min, demonstrating the direct relation between time and removal. At a higher contact time, the diesel removal was further increased due to sufficient contact between oxidizing agents and the target pollutant.
Effects of variations of contact time on TPH removal rate by sonication enhanced EK oxidation (pH = 5, V = 2.5 V cm-1, TPH = 2410 mg/L, US = 200 W).
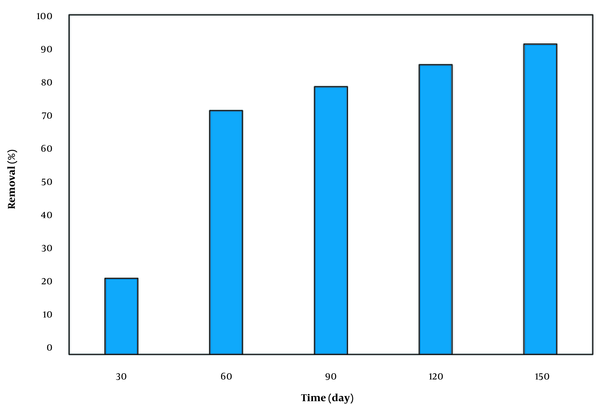
3.6. Kinetic Study
The kinetics study of treatment of diesel contaminated solution was performed at reaction time range of 30 - 150 min and TPH concentrations of 2410 mg/L at selected conditions. In oxidation reactions, pseudo-first-order and pseudo-second-order kinetic models usually are used to predict the rates of reactions in the solution, as given in equations 1 and 2 (7):
Where, C0 is initial TPH concentrations (mg/L), Ct residual TPH concentrations, t reaction time (h), k1 first order rate constant (h-1), and k2 pseudo-second-order rate constant (mole/Lh). Results of kinetic investigation verified pseudo-first-order model as the best fitted equation, as shown in Figure 5. In this regard, the rate constant (k1) of 0.0168 h-1 and the correlation coefficient of 0.94 were calculated for pseudo-first-order model. The obtained data are in accordance with similar studies (21, 22, 26, 27).
The pseudo-first-order model for TPH degradation by sonication enhanced EK oxidation.
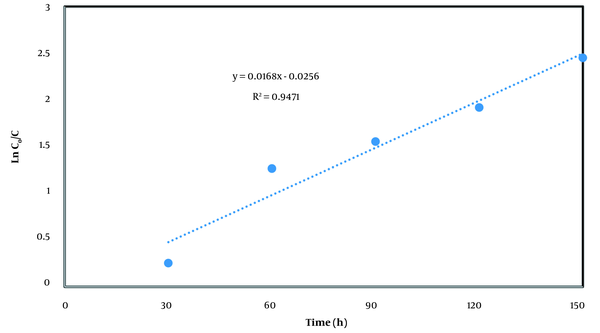
3.7. Alternative Processes
Combination of different oxidation methods to enhance the rate and extent of radical production and maximize the degradation rate is highly recommended by investigators (28, 29). Therefore, separate experiments were conducted to understand the contribution of each process in the integrated system. Results are presented in Figure 6. The results showed that the removal efficiency of EK, sonication, and integration of EK with sonication were 64%, 10%, and 71%, respectively (Figure 6).
The COD removal rate in alternative processes versus sonication enhanced EK oxidation.
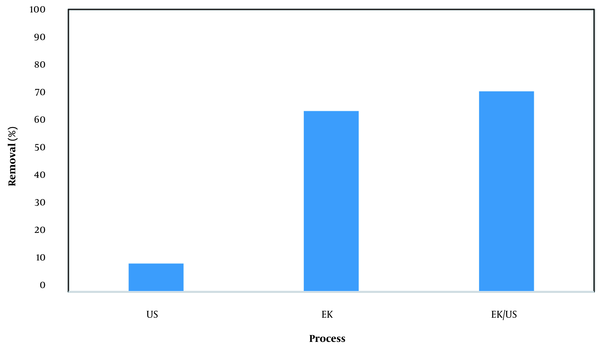
3.8. Conclusions
The treatment of a hydrocarbon contaminated wastewater using the integration of sonication with EK oxidation was investigated. The most TPH removal of 91% was obtained in selected levels of operational variables. According to obtained data, it can be concluded that the integration of sonication with EK oxidation can be considered as a suitable technology for diesel removal from wastewater. The kinetic data followed the pseudo-first-order kinetic model.
3.9. Limitations
Controlling the constant voltage and stability of electrodes which were destroyed during the oxidations were the main limitations of this study.
Acknowledgements
References
-
1.
Asfaram A, Fathi MR. [Removal of direct red 12B dye from aqueous solutions by wheat straw: Isotherms, kinetics and thermodynamic studies]. J Color Sci Technol. 2013;7(3):223-35. Persian.
-
2.
Kholghi S, Badii K, Ahmadi SH. [Bio-sorption isotherm and kinetic study of acid red 14 from aqueous solution by using Azolla A. filiculodes]. J Color Sci Technol. 2013;6:337-46. Persian.
-
3.
Pagga U, Brown D. The degradation of dyestuffs: Part II Behaviour of dyestuffs in aerobic biodegradation tests. Chemosphere. 1986;15(4):479-91. https://doi.org/10.1016/0045-6535(86)90542-4.
-
4.
Ehrampoosh M, Moussavi GH, Ghaneian M, Rahimi S, Ahmadian M. [Removal of methylene blue dye from textile simulated sample using tubular reactor and TiO2/UV-C photocatalytic process]. J Environ Health Sci Eng. 2011;8(1):34-40. Persian.
-
5.
Akbari A, Remigy JC, Aptel P. Treatment of textile dye effluent using a polyamide-based nanofiltration membrane. Chem Eng Process. 2002;41(7):601-9. https://doi.org/10.1016/s0255-2701(01)00181-7.
-
6.
Babu BR, Parande AK, Raghu S, Kumar TP. Cotton textile processing: Waste generation and effluent treatment. J Cotton Sci. 2007;11(3):141-53. [PubMed ID: IND43960251].
-
7.
Bazrafshan E, Alipour MR, Mahvi AH. Textile wastewater treatment by application of combined chemical coagulation, electrocoagulation, and adsorption processes. Desalination Water Treat. 2015;57(20):9203-15. https://doi.org/10.1080/19443994.2015.1027960.
-
8.
Kiani R, Mirzaei F, Ghanbari F, Feizi R, Mehdipour F. Real textile wastewater treatment by a sulfate radicals-advanced oxidation process: Peroxydisulfate decomposition using copper oxide (CuO) supported onto activated carbon. J Water Process Eng. 2020;38. https://doi.org/10.1016/j.jwpe.2020.101623.
-
9.
Bejankiwar R, Lalman JA, Seth R, Biswas N. Electrochemical degradation of 1,2- dichloroethane (DCA) in a synthetic groundwater medium using stainless-steel electrodes. Water Res. 2005;39(19):4715-24. [PubMed ID: 16289674]. https://doi.org/10.1016/j.watres.2005.09.012.
-
10.
Munoz M, de Pedro ZM, Casas JA, Rodriguez JJ. Triclosan breakdown by fenton-like oxidation. Chem Eng J. 2012;198-199:275-81. https://doi.org/10.1016/j.cej.2012.05.097.
-
11.
Sadrnourmohamadi M, Poormohammadi A, Almasi H, Asgari G, Ahmadzadeh A, Seid-Mohammadi A. Removal of 2, 4-dichlorophenol from aqueous solution using ultrasonic/H2O2. Desalination Water Treat. 2017;75:189-94. https://doi.org/10.5004/dwt.2017.20766.
-
12.
Feizi R, Ahmad M, Jorfi S, Ghanbari F. Sunset yellow degradation by ultrasound/peroxymonosulfate/CuFe2O4: Influential factors and degradation processes. Korean J Chem Eng. 2019;36(6):886-93. https://doi.org/10.1007/s11814-019-0268-0.
-
13.
Saez V, Esclapez Vicente MD, Frias-Ferrer AJ, Bonete P, Gonzalez-Garcia J. Electrochemical degradation of perchloroethylene in aqueous media: an approach to different strategies. Water Res. 2009;43(8):2169-78. [PubMed ID: 19303130]. https://doi.org/10.1016/j.watres.2009.02.019.
-
14.
Zhang AY, Long LL, Liu C, Li WW, Yu HQ. Electrochemical degradation of refractory pollutants using TiO2 single crystals exposed by high-energy {001} facets. Water Res. 2014;66:273-82. [PubMed ID: 25222331]. https://doi.org/10.1016/j.watres.2014.08.030.
-
15.
Alvarez-Guerra M, Quintanilla S, Irabien A. Conversion of carbon dioxide into formate using a continuous electrochemical reduction process in a lead cathode. Chem Eng J. 2012;207-208:278-84. https://doi.org/10.1016/j.cej.2012.06.099.
-
16.
Dai Q, Zhou J, Meng X, Feng D, Wu C, Chen J. Electrochemical oxidation of cinnamic acid with Mo modified PbO 2 electrode: Electrode characterization, kinetics and degradation pathway. Chem Eng J. 2016;289:239-46. https://doi.org/10.1016/j.cej.2015.12.054.
-
17.
Zhao C, Wang J. Electrochemical reduction of CO 2 to formate in aqueous solution using electro-deposited Sn catalysts. Chem Eng J. 2016;293:161-70. https://doi.org/10.1016/j.cej.2016.02.084.
-
18.
Lin H, Niu J, Ding S, Zhang L. Electrochemical degradation of perfluorooctanoic acid (PFOA) by Ti/SnO2-Sb, Ti/SnO2-Sb/PbO2 and Ti/SnO2-Sb/MnO2 anodes. Water Res. 2012;46(7):2281-9. [PubMed ID: 22381981]. https://doi.org/10.1016/j.watres.2012.01.053.
-
19.
Comninellis C, Chen G. Electrochemistry for the environment. Springer; 2010.
-
20.
Rahmani AR, Rezaeivahidian H, Almasi M, Shabanlo A, Almasi H. A comparative study on the removal of phenol from aqueous solutions by electro–fenton and electro–persulfate processes using iron electrodes. Res Chem Intermed. 2015;42(2):1441-50. https://doi.org/10.1007/s11164-015-2095-1.
-
21.
Ahmadi S, Igwegbe CA, Rahdar S. The application of thermally activated persulfate for degradation of Acid Blue 92 in aqueous solution. Int J Ind Chem. 2019;10(3):249-60. https://doi.org/10.1007/s40090-019-0188-1.
-
22.
Abtahi M, Jorfi S, Mehrabi N, Saeedi R, Darvishi Cheshmeh Soltani R, Barzegar G. A novel combination of surfactant addition and persulfate-assisted electrokinetic oxidation for remediation of pyrene-contaminated soil. Chem Biochem Eng Q. 2018;32(1):55-69. https://doi.org/10.15255/cabeq.2017.1204.
-
23.
Najafpoor AA, Davoudi M, Rahmanpour Salmani E. Decolorization of synthetic textile wastewater using electrochemical cell divided by cellulosic separator. J Environ Health Sci Eng. 2017;15:11. [PubMed ID: 28560044]. [PubMed Central ID: PMC5445381]. https://doi.org/10.1186/s40201-017-0273-3.
-
24.
Ghodbane H, Hamdaoui O. Degradation of acid blue 25 in aqueous media using 1700kHz ultrasonic irradiation: ultrasound/Fe(II) and ultrasound/H(2)O(2) combinations. Ultrason Sonochem. 2009;16(5):593-8. [PubMed ID: 19109046]. https://doi.org/10.1016/j.ultsonch.2008.11.006.
-
25.
Jiang Y, Pétrier C, David Waite T. Kinetics and mechanisms of ultrasonic degradation of volatile chlorinated aromatics in aqueous solutions. Ultrasonics Sonochem. 2002;9(6):317-23. https://doi.org/10.1016/s1350-4177(02)00085-8.
-
26.
Weng C, Tao H. Highly efficient persulfate oxidation process activated with Fe0 aggregate for decolorization of reactive azo dye Remazol Golden Yellow. Arab J Chem. 2018;11(8):1292-300. https://doi.org/10.1016/j.arabjc.2015.05.012.
-
27.
Shahamat YD, Farzadkia M, Nasseri S, Mahvi AH, Gholami M, Esrafili A. Magnetic heterogeneous catalytic ozonation: A new removal method for phenol in industrial wastewater. J Environ Health Sci Eng. 2014;12(1):50. [PubMed ID: 24572145]. [PubMed Central ID: PMC3974053]. https://doi.org/10.1186/2052-336X-12-50.
-
28.
Pang YL, Abdullah AZ, Bhatia S. Review on sonochemical methods in the presence of catalysts and chemical additives for treatment of organic pollutants in wastewater. Desalination. 2011;277(1-3):1-14. https://doi.org/10.1016/j.desal.2011.04.049.
-
29.
Li Y, Hsieh WP, Mahmudov R, Wei X, Huang CP. Combined ultrasound and fenton (US-Fenton) process for the treatment of ammunition wastewater. J Hazard Mater. 2013;244-245:403-11. [PubMed ID: 23274940]. https://doi.org/10.1016/j.jhazmat.2012.11.022.