Abstract
Background:
Beta-lactam resistance in gram-negative bacteria, especially Escherichia coli, is a main clinical problem. It is often caused by the co-production of β-lactamases, particularly extended-spectrum β-lactamases (ESBLs) and AmpC enzymes. It may lead to a problem for diagnosis via recommended phenotypic tests by the Clinical and Laboratory Standards Institute (CLSI). Conversely, β-lactamase genes have several subfamilies; therefore, using designed primers with high ability could be valuable to detect all of them.Objectives:
This investigation focused on evaluating the prevalence of extended-spectrum β-lactamases using disk diffusion method and confirmatory test (Combined Disk), as well as PCR for complete detection of these genes (SHV- and AmpC (CITM, FOX)- type β-lactamase genes).Materials and Methods:
500 clinical samples were collected from different hospitals of Tehran, Iran and 200 E. coli isolates were detected by standard biochemical tests such as IMVIC. These specimens were isolated from urine, stool, blood, and wound. Subsequently, the isolates were screened by disk diffusion method and combined disk assay for β-lactamase production. Resistant isolates were evaluated by PCR for molecular assessing.Results:
In disk agar diffusion test, 128(64%) E. coli isolates resistant to ceftazidime (55.5%) and cefotaxime (64%) were selected and followed by combined disk (ceftazidime, cefotaxime, and clavulanic acid) assay. In combined disk assay, among 128 screened isolates, 115 (89.8%) and 13 (10.2%) isolates were detected as ESBLs and AmpC producers, respectively. PCR performed on all 128 selected isolates via phenotypic tests and the results showed that among 115 and 13 isolates, 7 (6.1%) and 13(100%) cases contained blaSHV and blaCITM, respectively. Fox gene was not detected in any sample.Conclusions:
Our findings indicate that the prevalence of ESBLs in Tehran is rising. According to CLSI, isolates showing negative confirmatory test are potentially considered as producers of AmpC (the result of CITM PCR was 100% positive). On the other hand, co-production of ESBLs and AmpC may lead to ESBLs false negative. Thus, development of diagnosis methods for complete detection of β-lactamase enzymes is important for resistance control and the treatment with high achievement.Keywords
HV-Type Extended Spectrum Beta-Lactamases AmpC Beta-Lactamases
1. Background
Infection with resistant organisms is a major public health issue. Evolution of resistance to beta lactam antibiotics in gram negative pathogens, especially in Escherichia coli, frequently results from the production of β-lactamase enzymes with ability to hydrolyze β-lactam ring (1, 2).Today, Emerging newer β-lactamase enzymes, including extended-spectrum β-lactamases (ESBLs) and AmpC β-lactamases are associated with misuse of β-lactam antibiotics. On the other hand, E. coli strains possess several virulence factors leading to emergence of a multidrug-resistance (MDR) phenotype in this organism. This resistance to broad antimicrobial agents has increased the therapy failure, significantly (3, 4).
The SHV-type of ESBLs is encoded by chromosomal genes in isolates of Klebsiella and extended via transport to mobile elements such as plasmids among microbial societies, especially Enterobacteriaceae family (5).The detection of ESBLs is believed to be highly significant as they are the most important reasons for therapy failure (6). One recognition criteria for ESBLs is an initial screening of CLSI-recommended cephalosporin's indicators, especially ceftazidime and cefotaxime, and subsequently, performing confirmatory tests with clavulanic acid (as an inhibitor of ESBLs). In this test, if the zone of inhibition (ZOI) in the presence of clavulanic acid is greater than the ZOI in its absence, the organisms are diagnosed as ESBLs producers (7, 8). But in the past decade, the appearance of AmpC β-lactamases in pathogens led to a problem for ESBLs diagnosis via disk diffusion method because this enzyme was resistant to β-lactamase inhibitors such as clavulanic acid and it isn’t inhibited by clavulanic acid. Co-production of ESBLs and AmpC β-lactamases in some pathogens, principally E. coli, is increasing and provides a broader spectrum of resistance (9, 10). As a consequence, using molecular methods for better interpretation of phenotypic tests is essential. Alternatively, β-lactamase super families such as SHV and AmpC (CITM, FOX) genes have several subfamilies; accordingly, in this survey employing designed primers with high ability to detect these genes was preferred (7, 11).
2. Objectives
This study was aimed to assess the prevalence of ESBLs and also to detect SHV- and AmpC (CITM, FOX)-type β-lactamase genes by using designed primers through PCR assay.
3. Materials and Methods
3.1. Bacterial Strains
Over 500 clinical samples including urine, stool, blood, and wound were collected from Tehran hospitals from which 200 E. coli isolates were detected by IMVIC standard biochemical tests including indole, methyl red, Voges-Proskauer, and citrate. Afterward, all strains of isolated E. coli were stored in skim milk at 70°C for further usage in the tests.
3.2. Screening and Phenotypic Identification of ESBLs and AmpC
Disk diffusion method on mueller-hinton agar was used for initial screening of E. coli isolates producing ESBLs. The following antibiotics were used: cefotaxime (30 µg), ceftazidime (30 µg), gentamicin (10 µg), amoxicillin (30 µg), imipenem (10 µg), nalidixic acid (30 µg), streptomycin (10 µg), co-trimoxazole (1.25 µg), ciprofloxacin (5 µg), and chloramphenicol (30 µg) (Mast Diagnostics Ltd., UK). After incubation for 24h at 37°C, the zone of inhibition around each disk was measured and compared with global standards of CLSI; the results were reported as resistant, intermediate, and sensitive (12).
3.3. Polymerase Chain Reaction and Sequencing of the β-Lactamase Genes
Genomic DNA of positive screens for ESBLs and AmpC was extracted by extraction kit (Bioneer, Seoul, Korea) according to the manufacturer’s instructions, followed by PCR assay for amplification of blaSHV, blaCITM, and blaFOX using designed primers for each cluster ( Table 1 ). Multiplex PCR for blaSHV and blaCITM was also carried out with the same conditions. Subsequently, the amplicons were evaluated by electrophoresis in 0.8% agarose gel. In this study, K. pneumoniae ATCC 7881 was taken as positive control for blaSHV expression. The band of PCR product which was equivalent to expected amplification size in each cluster was extracted from gel by kit (Fermentas, Germany) according to manufacturer’s instructions and sent to Macrogen Research, Seoul, Korea for analyzing via sequencing.
Primers Used for Amplification
Primer | Sequence (5`to 3` as Synthesized) | Expected Amplicon Size (bp) | |
---|---|---|---|
SHV | SHVF | GCCTGTGTATTATCTCCCTGTTAGC | 764 |
SHVR | CAGATAAATCACCACAATGCGC | ||
LAT-1 to LAT-4, CMY-2 to CMY-7, CMY-21,-22,-24,-28, -32,-33,-43,-44, BIL-1 | CITMF | CAGCCTCTTTCTCCACATTTGC | 575 |
CITMR | CAGTTTTAATGGTTGCAGGACG | ||
FOX-1 to FOX-5b | FOXMF | TGAGCTTCCAGGCCAATCC | 167 |
FOXMR | GATGGGATAGTTGCGATTGGC |
3.4. Design of Primers
Submitted sequences related to SHV, CITM, and FOX genes of E. coli in GenBank were 50, 24, and 5, respectively. The sequences of each cluster were aligned by MEGA 4 multiple-alignment program until analogous locals were identified. These locals were used for designing primers by Generunner software. Then, the designed primers were tested in silico for homology with submitted sequences by using BLAST. Then, three sets of designed primers were evaluated by PCR and sequenced in wet lab.
4. Results
In our study, 200 clinical isolates of E. coli were collected within 6 months of which 125 (62.5%) isolates were from urine and urinary catheter, 48 (24%) isolates from stool, 18 (9%) isolates from blood, and 5 (2.5 %) isolates from wound. Resistant pattern of 200 E. coli isolates to 10 antimicrobial agents are shown in Table 2 . The majority of isolates showed a high degree of resistance to oxyimino cephalosporins but appeared to be susceptible to imipenem. Up to 70% of the isolates exhibited a multidrug-resistance (MDR) phenotype.
Pattern of Resistance to 10 Antimicrobial agents Among 200 E. coli Isolates.
Resistance, No. (%) | Intermediate, No. (%) | Sensitive, No. (%) | |
---|---|---|---|
Imipenem | 1 (0/5) | 0 | 199 (99.5) |
Ciprofloxacin | 109 (54.5) | 16 (8) | 75 (37.5) |
Gentamicin | 78 (39) | 4 (2) | 118 (59) |
Chloramphenicol | 61 (30.5) | 36 (18) | 103 (51.5) |
Streptomycin | 143 (71.5) | 29 (14.5) | 28 (14) |
Cefotaxime | 128 (64) | 7 (3.5) | 65 (32.5) |
Ceftazidime | 111 (55.5) | 11 (5.5) | 78 (39) |
Co-trimoxazole | 161 (80.5) | 3 (1.5) | 36 (18) |
Nalidixic acid | 148 (74) | 13 (6.5) | 39 (19.5) |
Amoxicillin | 189 (94.5) | 3 (1.5) | 8 (4) |
4.1. Phenotypic Identification of ESBLs and AmpC
In disk diffusion method, 128 (64%) E. coli isolates resistant to ceftazidime and cefotaxime were selected and followed by combined disk assay for possibly positive ESBLs. In combined disk assay, among 128 screened isolates, 115 (89.8%) and 13 (10.2%) isolates were detected as ESBLs and AmpC producers, respectively ( Figure 1 ). ESBL-expressing strains of E. coli were seen more commonly in urinary samples (80%).
Screening and Phenotypic Identification of ESBLs and AmpC.
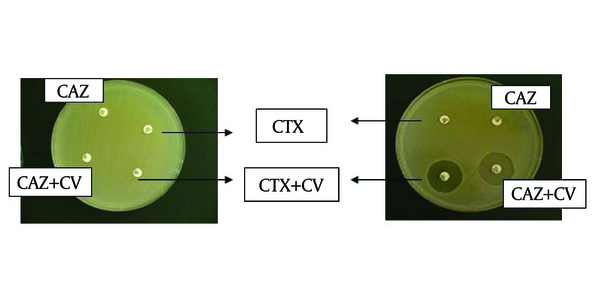
4.2. PCR
PCR was performed on all 128 resistant isolates and the results among these isolates showed that 7 (5.5%) and 13 (10.2%) isolates had blaSHV and blaCITM , respectively. Fox gene was not detected in any sample. The result of multiplex PCR (blaSHV and blaCITM) in relation to one strain was positive which led to ESBLs false negative ( Figure 2 ). SHV and CITM sequence analyses also showed high similarity to the submitted sequences related to these genes (blaSHV and blaCITM) in GenBank.
PCR Amplification of blaSHV and blaCITM.
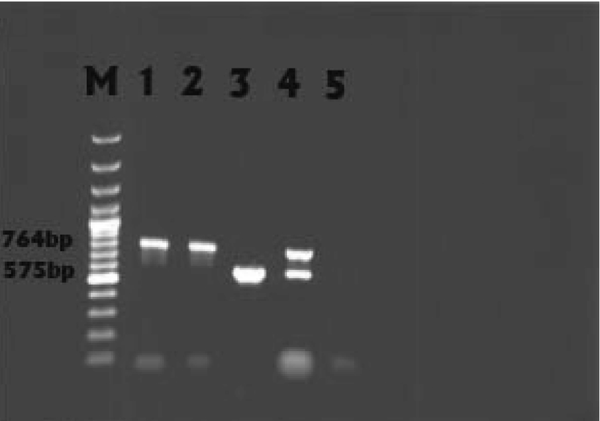
5. Discussion
The prevalence of β-lactamase producers is different from one year to another, and even from one nation to another(15). For example, among 7054 E. coli samples collected between 1994 and 1996 in Barcelona (Hospital de la Santa Creu i Sant Pau), the prevalence of ESBL-producing strains was 0.14% (16). In 2001, this prevalence increased to 2.1% (17). Susceptibility data in other studies have shown that ESBLs prevalence in Iran was more than other countries which was attributed to irregular consumption of β-lactam antibiotics, especially expanded cephalosporins. Therefore, the resistant rate of E. coli to cephalosporin`s indicators in this study (32.11%), was higher than that reported in the study of Nakhaei et al. (1, 15).
In our study, among 128 screened E. coli isolates in disk diffusion method, only 115 isolates were selected for ESBLs producers by using CLSI-recommended confirmatory method (combined disk assay). Today, the significant challenge for diagnostic laboratories is detection of extended-spectrum β-lactamases in the presence of AmpC β-lactamase enzymes because there is no guideline (CLSI) for identification of AmpC which leads to a diagnostic problem for ESBLs via phenotypic methods and occurrence of ESBLs false negative (18).
The misidentification of ESBLs by diagnostic laboratories could result in wrong therapy via unsuitable prescription of cephalosporins leading to failure in treatment and even causing death (19). However, according to the recommendation of the Clinical and Laboratory Standards Institute (CLSI), isolates showing negative confirmatory test are potentially producer of AmpC and should be evaluated by molecular methods (13, 20). Thus, PCR was performed on all 128 resistant isolates. This survey improved recommendation of CLSI because among 13 positive screens for AmpC in confirmatory test, the result of CITM PCR was 100% positive. On the other hand, the multiplex PCR was performed on all AmpC producers to prove mentioned recommendation.
The result of multiplex PCR (blaSHV and blaCITM) in relation to one strain was positive (Figure 2) which led to ESBLs false negative. These data indicate that ESBLs production could be covered by AmpC enzymes. So, using only confirmatory tests based on inhibitory effect of clavulanic acid is not sufficient to detect phenotypic ESBLs, and employing molecular assays is essential. The blaSHV gene was only detected in 5.5% of the isolates tested. Other ESBL genes such as TEM, OXA, and CTX-M may be responsible for ESBL phenotype (21). The rate of blaSHV in this study was very similar to that reported in the Swedish hospital (6%-2001 to 2006) (22), was nearly similar to that in Seoul National University Hospital in Korea (8.7% - 1995 to 1999) (23), and a little higher than that reported in Thailand (3.8% - 2005) (24); it was lower than that reported in university hospital in Salamanca, Spain (15.6%-2001 to 2004) (25) and in Turkish hospitals( 28.6%-2007) (26).
Extended-spectrum β-lactamases (ESBLs) and AmpC β-lactamases are first described in 1983 (Germany) and 1988 (India), respectively. Subsequently, a variety of these enzymes have been extended worldwide and lots of pathogens, especially Klebsiella spp. and E. coli , which were associated with failure in treatment and increasing the mortality were found (3, 27). In many clinical institutes as well as in lots of investigations, there is no attention toward the effects of AmpC β-lactamases in phenotypic tests (28). Based on data of this study, we suggest that laboratories are required to improve their reorganization methods for complete detection of AmpC and ESBLs co-producer organisms.
Acknowledgements
References
-
1.
Bakhtiari R, Fallah Mehrabadi J, Molla Agamirzaei H, Sabbaghi A, Soltan Dallal MM. 2011;2(8).
-
2.
Rayamajhi N, Kang SG, Lee DY, Kang ML, Lee SI, Park KY, et al. Characterization of TEM-, SHV- and AmpC-type beta-lactamases from cephalosporin-resistant Enterobacteriaceae isolated from swine. Int J Food Microbiol. 2008;124(2):183-7. [PubMed ID: 18455821]. https://doi.org/10.1016/j.ijfoodmicro.2008.03.009.
-
3.
Ahmed MO, Clegg PD, Williams NJ, Baptiste KE, Bennett M. Antimicrobial resistance in equine faecal Escherichia coli isolates from North West England. Ann Clin Microbiol Antimicrob. 2010;9(12). [PubMed ID: 20374640]. https://doi.org/10.1186/1476-0711-9-12.
-
4.
Bradford PA. Extended-spectrum beta-lactamases in the 21st century: characterization, epidemiology, and detection of this important resistance threat. Clin Microbiol Rev. 2001;14(4):933-51. [PubMed ID: 11585791].
-
5.
Paterson DL, Bonomo RA. Extended-spectrum beta-lactamases: a clinical update. Clin Microbiol Rev. 2005;18(4):657-86. [PubMed ID: 16223952]. https://doi.org/10.1128/CMR.18.4.657-686.2005.
-
6.
Netzel TC, Jindani I, Hanson N, Turner BM, Smith L, Rand KH. The AmpC inhibitor, Syn2190, can be used to reveal extended-spectrum beta-lactamases in Escherichia coli. Diagn Microbiol Infect Dis. 2007;58(3):345-8. [PubMed ID: 17379469]. https://doi.org/10.1016/j.diagmicrobio.2007.01.017.
-
7.
Pitout JD, Laupland KB. Extended-spectrum beta-lactamase-producing Enterobacteriaceae: an emerging public-health concern. Lancet Infect Dis. 2008;8(3):159-66. https://doi.org/10.1016/S1473-3099(08)70041-0.
-
8.
Sturenburg E, Mack D. Extended-spectrum beta-lactamases: implications for the clinical microbiology laboratory, therapy, and infection control. J Infect. 2003;47(4):273-95. https://doi.org/10.1016/S0163-4453(03)00096-3.
-
9.
Deshpande LM, Jones RN, Fritsche TR, Sader HS. Occurrence of plasmidic AmpC type beta-lactamase-mediated resistance in Escherichia coli: report from the SENTRY Antimicrobial Surveillance Program (North America, 2004). Int J Antimicrob Agents. 2006;28(6):578-81. [PubMed ID: 17112706]. https://doi.org/10.1016/j.ijantimicag.2006.07.025.
-
10.
Goossens H, Grabein B. Prevalence and antimicrobial susceptibility data for extended-spectrum beta-lactamase- and AmpC-producing Enterobacteriaceae from the MYSTIC Program in Europe and the United States (1997-2004). Diagn Microbiol Infect Dis. 2005;53(4):257-64. [PubMed ID: 16360549]. https://doi.org/10.1016/j.diagmicrobio.2005.10.001.
-
11.
Jacoby GA, Medeiros AA. More extended-spectrum beta-lactamases. Antimicrob Agents Chemother. 1991;35(9):1697-704. https://doi.org/10.1128/AAC.35.9.1697.
-
12.
Performance standards for antimicrobial susceptibility testingClinical and Laboratory Standards Institute. Performance standards for antimicrobial susceptibility testing 17th informational supplement. M100-S17. 2007.
-
13.
Song W, Bae K, Lee Y-N, Lee C-H, Lee SH, Jeong SH. Detection of extended-spectrum β -lactamases by using Boronic acid as an AmpC β -lactamase inhibitor in clinical isolates of Klebsiella spp. and Escherichia coli. J Clin Microbiol. 2007;45(4):1180-4. [PubMed ID: 17301276]. https://doi.org/10.1128/JCM.02322-06.
-
14.
Coudron PE, Moland ES, Thomson KS. Occurrence and detection of AmpC beta-lactamases among Escherichia coli, Klebsiella pneumoniae, and Proteus mirabilis isolates at a veterans medical center. J Clin Microbiol. 2000;38(5):1791-6. [PubMed ID: 10790101].
-
15.
Al-Jasser AM. Extended-spectrum beta-lactamases (ESBLs): a global problem. Kuwait Med J. 2006;38(3):171-85.
-
16.
Sabate M, Miro E, Navarro F, Verges C, Aliaga R, Mirelis B, et al. Beta-lactamases involved in resistance to broad-spectrum cephalosporins in Escherichia coli and Klebsiella spp. clinical isolates collected between 1994 and 1996, in Barcelona (Spain). J Antimicrob Chemother. 2002;49(6):989-97. [PubMed ID: 12039891]. https://doi.org/10.1093/jac/dkf057.
-
17.
Kenny MJ, Birtles RJ, Day MJ, Shaw SE. Community transmission of extended-spectrum β-lactamase. Emerg Infect Dis. 2003;9(8):1024-5. [PubMed ID: 12971376]. https://doi.org/10.3201/eid0908.030094.
-
18.
Hanson ND. AmpC beta-lactamases: what do we need to know for the future? J Antimicrob Chemother. 2003;52(1):2-4. [PubMed ID: 12775673]. https://doi.org/10.1093/jac/dkg284.
-
19.
Paterson DL, Ko WC, Von GA, Casellas JM, Mulazimoglu L, Klugman KP. Outcome of cephalosporin treatment for serious infections due to apparently susceptible organisms producing extended-spectrum β -lactamases: Implications for the clinical microbiology laboratory. J Clin Microbiol. 2001;39(6):2206-12. [PubMed ID: 11376058]. https://doi.org/10.1128/JCM.39.6.2206-2212.2001.
-
20.
Walther-Rasmussen J, Hoiby N. Plasmid-borne AmpC beta-lactamases. Can J Microbiol. 2002;48(6):479-93. https://doi.org/10.1139/w02-039.
-
21.
Zhang Z, Guo X, Zhang Q. Prevalence characterization of extended‐spectrum β‐lactamases among Escherichia coli isolates collected in Zhengzhou. J Clin Lab analys. 2009;23(6):404-7. [PubMed ID: 19927355]. https://doi.org/10.1002/jcla.20351.
-
22.
Fang H, Ataker F, Hedin G, Dornbusch K. Molecular epidemiology of extended-spectrum beta-lactamases among Escherichia coli isolates collected in a Swedish hospital and its associated health care facilities from 2001 to 2006. J Clin Microbiol. 2008;46(2):707-12. [PubMed ID: 18094139]. https://doi.org/10.1128/JCM.01943-07.
-
23.
Kim J, Lee HJ. Rapid discriminatory detection of genes coding for SHV beta-lactamases by ligase chain reaction. Antimicrob Agents Chemother. 2000;44(7):18-604. https://doi.org/10.1128/AAC.44.7.1860-1864.2000.
-
24.
Kiratisin P, Apisarnthanarak A, Laesripa C, Saifon P. Molecular characterization and epidemiology of extended-spectrum- β -lactamase-producing Escherichia coli and Klebsiella pneumoniae isolates causing Health Care-Associated infection in Thailand, where the CTX-M family is endemic. Antimicrob Agents Chemother. 2008;52(8):2818-24. [PubMed ID: 18505851]. https://doi.org/10.1128/AAC.00171-08.
-
25.
Romero ED, Padilla TP, Hernandez AH, Grande RP, Vazquez MF, Garcia IG. Prevalence of clinical isolates of Escherichia coli and Klebsiella spp. producing multiple extended-spectrum beta-lactamases. Diagn Microbiol Infect Dis. [PubMed ID: 17913435]. https://doi.org/10.1016/j.diagmicrobio.2007.06.007.
-
26.
Hosoglu S, Gundes S, Kolayli F, Karadenizli A, Demirdag K, Gunaydin M. Extended-spectrum beta-lactamases in ceftazidime-resistant Escherichia coli and Klebsiella pneumoniae isolates in Turkish hospitals. Indian J Med Microbiol. 2007;25(4):346-50. [PubMed ID: 18087082]. https://doi.org/10.4103/0255-0857.37336.
-
27.
Thomson KS. Controversies about extended-spectrum and AmpC beta-lactamases. Emerg Infect Dis. 2001;7(2):333-6. [PubMed ID: 11294735]. https://doi.org/10.3201/eid0702.010238.
-
28.
Jeong HS, Bae IK, Shin JH, Jung HJ, Kim SH, Lee JY, et al. Prevalence of plasmid-mediated quinolone resistance and its association with extended-spectrum beta-lactamase and AmpC beta-lactamase in Enterobacteriaceae. Korean J Lab Med. 2011;31(4):257-64. [PubMed ID: 2201667]. https://doi.org/10.3343/kjlm.2011.31.4.257.