Abstract
Background:
The Pseudomonas aeruginosa porin OprD is a substrate-specific porin that facilitates the diffusion of basic amino acids, small peptides, and carbapenems into the cell. OprD-mediated resistance occurs as a result of decreased transcriptional expression of oprD and/or loss of function mutations that disrupt protein activity.Objectives:
In this study, we examined the level of oprD expression in P. aeruginosa clinical isolates to determine the contribution of OprD porins in carbapenem resistance.Materials and Methods:
Included strains were divided into two groups, comprised of multidrug-resistant (MDR) and isolated carbapenem-resistant (ICR) strains. The transcription product level of oprD was identified using real-time polymerase chain reaction (qPCR).Results:
Of the 18 clinical isolates, a decrease in the oprD level was found to be significant in 13 isolates. Nine of eighteen isolates with a significant decrease were determined in the first group and comprised MDR isolates that showed a statistically significant difference compared with the ICR group (P = 0.001). In the ICR group, oprD levels were found to be significantly low in 4 isolates. Six different patterns were determined by comparing band profiles in AP-PCR.Conclusions:
Although the data support the idea that the basic mechanism of imipenem resistance could be via the loss of oprD, they do not fully explain the role of oprD and indicate that other mechanisms may play an important role. Additionally, the significant decrease in the oprD levels in MDR strains suggests that oprD also plays a role in the emergence of both carbapenem and non-carbapenem resistance.Keywords
Pseudomonas aeruginosa OprD Porin Protein qPCR AP-PCR oprD Expression
1. Background
The outer membrane of Gram-negative bacteria constitutes a semipermeable barrier that slows the penetration of antibiotics. The permeability of this barrier is known to vary greatly among species, with the outer membrane of Pseudomonas aeruginosa only 8% as permeable as that of Escherichia coli (1). This decreased permeability severely restricts the uptake of nutrients and other important compounds into the cell, which consequently must be imported into cells using a collection of water-filled protein channels called porins.
The P. aeruginosa family of porin proteins, defined based on their apparent sequence homology within the P. aeruginosa genome, plays an important physiological role in the transport of substances required for metabolism. However, these proteins also exhibit an affinity for certain hydrophilic antibiotics, such as β-lactams, aminoglycosides, tetracyclins, and some fluoroquinolones, allowing these compounds to transverse the otherwise insoluble outer bacterial membrane (2, 3). Deletion of one or more porin proteins has been shown to reduce the susceptibility of P. aeruginosa to certain antibacterial agents (4).
The P. aeruginosa porin OprD is a substrate-specific porin that facilitates the diffusion of basic amino acids, small peptides, and carbapenems into the cell (5). OprD-mediated resistance occurs as a result of decreased transcriptional expression of oprD and/or loss of function mutations that disrupt protein activity. Specific mechanisms resulting in decreased transcriptional expression of oprD include (i) disruption of the oprD promoter, (ii) premature termination of oprD transcription, (iii) co-regulation with trace metal resistance mechanisms, (iv) salicylate-mediated reduction, and (v) decreased transcriptional expression via co-regulation with the multidrug efflux pump encoded by mexEF-oprN (6).
2. Objectives
In this study, we examined the level of oprD expression in P. aeruginosa clinical isolates to determine the contribution of OprD porins in carbapenem resistance. Clinical isolates were further examined using additional molecular methods to determine the degree of variability among isolates.
3. Materials and Methods
3.1. Bacterial Isolates and Antibiotic Susceptibility Testing
Pseudomonas aeruginosa isolates were obtained from clinical samples sent to our laboratory routinely. Species identification was performed using conventional methods. Antibiotic susceptibility testing was carried out using the Kirby-Bauer disc diffusion method (7). Isolates were divided into two groups according to their resistance status: multiple-drug resistant (MDR) and isolated carbapenem resistant (ICR).
3.2. Minimum Inhibitory Concentration (MIC) Testing
Minimum inhibitory concentrations (MIC) of ceftazidime (CAZ), gentamicin (CN), piperacillin-tazobactam (TZP), ciprofloxacin (CIP), imipenem (IMP), and meropenem (MEM) were determined using the Vitek 2 system (bioMerieux, France). The Densi-Check 2 system (bioMérieux, France) was used to calibrate the turbidity of samples against the 0.5 McFarland standard. Susceptibility tests were performed on the Vitek 2 system using AST-N174 cards, according to the manufacturer’s instructions. MIC values of ≥ 32 µg/mL for ceftazidime, ≥ 16 µg/mL for gentamicin, ≥ 128 µg/mL for piperacillin-tazobactam, ≥ 4 µg/mL for ciprofloxacin, ≥ 16 µg/mL for imipenem, and ≥ 16 µg/mL for meropenem were defined as resistance (7).
3.3. Quantitative Real-Time PCR
Transcript levels of oprD were analyzed by real-time polymerase chain reaction (qPCR) using a LightCycler instrument (Roche Diagnostics, Germany). Total RNA was extracted using the High Pure RNA Isolation Kit (Roche Diagnostics, Germany) and converted into cDNA for qPCR using the Transcriptor High-fidelity cDNA Synthesis Kit (Roche Diagnostics, Germany). The quality and purity of the RNA obtained was evaluated spectrophotometrically (Maestrogen Nanodrop, USA). As a result of evaluation, required volume was calculated for 100 ng cDNA. Quantitative PCR was performed in capillary glass using the LightCycler FastStart DNA Master SYBR Green I Kit (Roche Diagnostics, Germany) with primers specific for oprD, and rpsL (Table 1).
PCR Primers Used in This Study
Control cDNA was obtained from P. aeruginosa strain PAO1. Amplification of triplicate cDNA samples from each isolate was performed under the following conditions: initial denaturation for 10 minutes at 95ºC, followed by 45 cycles of denaturation at 95ºC for 20 seconds, annealing at 68ºC for 10 seconds, and elongation at 72ºC for 15 seconds. A final melting curve analysis was performed using a single read at 90ºC.
3.4. Arbitrarily Primed PCR (AP-PCR)
To evaluate the similarities among strains, arbitrarily primed PCR (AP-PCR) was performed using an M13 primer of sequence 5’-GAGGGTGGCGGTTCT-3’. PCR was carried out under the following conditions: 2 cycles of 94ºC for 5 minutes, 40ºC for 5 minutes, and 72ºC for 5 minutes, followed by 40 cycles of 94ºC for 1 minute, 40ºC for 1 minute, and 72ºC for 2 minutes. Amplification products were identified by agarose gel electrophoresis, and similarities among isolates were evaluated by comparison of the band profiles.
3.5. Evaluation of Gene Expression
Transcription data were analyzed using the LightCycler Relative Quantification software. Relative expression values (R) were determined using the ‘ΔΔCt’ method; the gene encoding ribosomal protein RpsL was used as a control (10). P. aeruginosa strain PAO1 was used as a standard for normalization of relative mRNA levels. Reduced oprD expression was defined as transcription levels ≤ 70% of those of the PAO1 isolate (11).
Primer dimers and other artifacts were evaluated by melting curve analysis. To confirm that specific amplification had occurred, the melting curves of each amplicon were assessed and compared with Tm values obtained using PAO1 DNA as the template.
3.6. Statistical Analyses
All statistical analyses were carried out using SPSS statistical software (version 17.0). Comparisons among groups were performed using a one-way ANOVA test.
4. Results
4.1. Antibiotic Susceptibility Testing
Clinical isolates were divided into two groups based on their drug susceptibility profiles. MDR isolates were defined as those exhibiting resistance to ceftazidime (MIC ≥ 32), piperacillin (MIC ≥ 128), imipenem (MIC ≥ 16), and gentamicin (MIC ≥ 16) (12). All ICR isolates were resistant to imipenem, and an additional three isolates (33%) also exhibited resistance to meropenem. Antibiotic sensitivities and MIC data for each group are summarized in Table 2.
Antimicrobial Susceptibilities and Gene Transcription Levels of P. aeruginosa Isolates
Isolate | Groupa | AP-PCR Categoryb | MIC, mg/L Levels | Relative Gene Expressionc (oprD) | |||||
---|---|---|---|---|---|---|---|---|---|
CAZ | TZP | CN | IPM | MEM | CIP | ||||
1 | 1 | 1 | ≥ 64 | ≥ 128 | ≥ 16 | ≥ 16 | 8 | ≥ 4 | 0.165 |
2 | 1 | 1 | ≥ 64 | ≥ 128 | ≥ 16 | ≥ 16 | ≥ 16 | ≥ 4 | 0.285 |
3 | 1 | 1 | ≥ 64 | ≥ 128 | ≥ 16 | ≥ 16 | ≥ 16 | ≥ 4 | 0 |
4 | 1 | 2 | ≥ 64 | ≥ 128 | ≥ 16 | ≥ 16 | ≥ 16 | ≥ 4 | 0 |
5 | 1 | 2 | ≥ 64 | ≥ 128 | ≥ 16 | ≥ 16 | ≥ 16 | ≥ 4 | 0.005 |
6 | 1 | 2 | ≥ 64 | ≥ 128 | ≥ 16 | ≥ 16 | ≥ 16 | ≥ 4 | 0 |
7 | 1 | 3 | ≥ 64 | ≥ 128 | ≥ 16 | ≥ 16 | ≥ 16 | ≥ 4 | 0.002 |
8 | 1 | 3 | ≥ 64 | ≥ 128 | ≥ 16 | ≥ 16 | ≥ 16 | ≥ 4 | 0 |
9 | 1 | 3 | ≥ 64 | ≥ 128 | ≥ 16 | ≥ 16 | ≥ 16 | ≥ 4 | 0.001 |
10 | 2 | 4 | 2 | 8 | ≤ 1 | ≥ 16 | 4 | ≤ 0.25 | 0.029 |
11 | 2 | 4 | 2 | 8 | ≤ 1 | ≥ 16 | 8 | ≤ 0.25 | 0.034 |
12 | 2 | 4 | 4 | 32 | ≤ 1 | ≥ 16 | ≥ 16 | ≤ 0.25 | 0.04 |
13 | 2 | 5 | 4 | 8 | ≤ 1 | ≥ 16 | 4 | ≤ 0.25 | 0.509 |
14 | 2 | 5 | 4 | 16 | ≤ 1 | ≥ 16 | ≥ 16 | ≤ 0.25 | 0.28 |
15 | 2 | 5 | 2 | 8 | ≤ 1 | ≥ 16 | 4 | ≤ 0.25 | 2 |
16 | 2 | 6 | 2 | ≤ 4 | ≤ 1 | ≥ 16 | ≤ 0.25 | ≤ 0.25 | 0.736 |
17 | 2 | 6 | 4 | ≤ 4 | ≤ 1 | ≥ 16 | 4 | ≤ 0.25 | 14.256 |
18 | 2 | 6 | 4 | 64 | 2 | ≥ 16 | ≥ 16 | ≤ 0.25 | 0.875 |
4.2. Gene Expression
Relative mRNA expression levels of oprD were determined by qPCR. Decreased oprD expression was observed in 16 of 18 P. aeruginosa clinical isolates, with significant decreases detected in 13 isolates (72%). Within the MDR group, oprD expression was significantly decreased in all 9 isolates. oprD levels were decreased in 7 ICR isolates, with significant decreases found in 4 of these isolates. Detailed expression data for each group are shown in Table 2. Due to the consistently low expression seen in the MDR group, differences in mRNA expression between the groups were statistically significant (P = 0.001).
4.3. AP-PCR Analysis
The genetic similarity among isolates was determined using AP-PCR. Among the 18 clinical isolates tested, six distinct banding patterns were identified (Figure 1). Classifications of P. aeruginosa isolates based upon AP-PCR analysis are shown in Table 2.
Agarose Gel Electrophoresis of Amplification Products Following AP-PCR
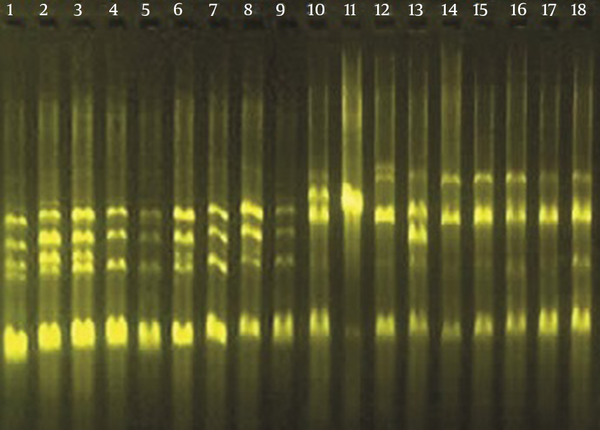
5. Discussion
Pseudomonas aeruginosa represents a phenomenon of bacterial resistance, and most of the known antimicrobial resistance mechanisms are displayed in this species, and multiple resistance mechanisms may be expressed simultaneously within the same isolate (4). Due to the increase in multi-drug resistant P. aeruginosa infections, a greater emphasis has been placed on identifying genetic characteristics underlying bacterial resistance and the clinical implications of these mutations.
Outer membrane protein OprD is considered the preferred portal of entry for carbapenems and similar drugs such as imipenem and meropenem also enter the cell via OprD (3, 13, 14). Any loss of OprD expression from the outer membrane significantly decreases the susceptibility of P. aeruginosa to carbapenems and has been shown to play a major role in the acquired resistance to imipenem and, to a lesser extent, meropenem (15). One study showed that OprD expression was decreased in the vast majority of 29 multi-drug resistant P. aeruginosa isolates (97%) and played a significant role in their carbapenem resistance (16).
Expression of oprD was decreased significantly in 13 of the 18 imipenem-resistant P. aeruginosa clinical isolates examined in this study, including all 9 MDR isolates. The frequency of decreased oprD expression in MDR isolates was significantly higher than that of ICR isolates (P = 0.001), suggesting that oprD also plays an important role in the emergence of both carbapenem and non-carbapenem resistance.
The impact of OprD-mediated resistance on carbapenems can be quantified relative to its effect on the antibacterial potency of carbapenems (6). In a study evaluating isogenic wild-type and OprD-deficient mutant pairs, the loss of OprD decreased the susceptibility of P. aeruginosa to meropenem 4- to 32-fold, compared with 4- to 16-fold for imipenem and 8- to 32-fold for doripenem (14). Zeng et al. (17) investigated relative gene expression in 29 carbapenem-resistant and ceftazidime- and cefepime-sensitive P. aeruginosa clinical isolates and found that the loss of oprD was directly related to carbapenem resistance. In another study, Fournier et al. (18) detected loss of oprD, as a result of mutations or gene disruptions, in 94 of 109 (86.2%) imipenem-resistant P. aeruginosa isolates.
In this study, oprD mRNA levels were decreased in 7 of 9 ICR isolates evaluated, even though significance was observed in only 4 of these isolates, in contrast with prior studies. Although our data are consistent with the basic mechanism of imipenem resistance mediated by diminished OprD protein levels in the outer membrane, the poor correlation between oprD mRNA expression and carbapenem resistance suggests involvement of additional resistance mechanisms in these isolates.
Impermeability was long thought to be the driver of intrinsic resistance in P. aeruginosa; however, resistance has since been found to involve a more complex interplay between impermeability and multi-drug efflux pumps (19). The interaction of efflux pumps with meropenem differs from that with imipenem. While it is believed that both meropenem and imipenem are able to enter the cell via the OprD pathway, only meropenem is a substrate of the MexAB-OprM efflux pump (19). Furthermore this mechanism plays a role in the emergence of resistance to fluoroquinolones and other β-lactams, increasing the likelihood of cross-resistance (20). However, despite this additional mechanism, meropenem resistance is less likely to be acquired than imipenem resistance (77 vs. 68% sensitivities for meropenem and imipenem, respectively), as it requires both the loss of oprD expression and upregulation of MexAB-OprM (6, 21). In our study, all 18 P. aeruginosa clinical isolates were resistant to imipenem, compared with only 12 isolates exhibiting meropenem resistance, consistent with previously published reports.
The relationship between OprD deficiency and imipenem resistance has been well established; however, cases of discordant OprD expression and carbapenem susceptibility, due to genetic versatility and multiple resistance mechanisms displayed in this pathogen, have been reported (6). El Amin et al. (15) identified four imipenem-susceptible isolates with significant reductions in oprD mRNA levels caused by severe oprD mutations that resulted in frame shifts or premature termination. Furthermore, they reported that the oprD mRNA levels did not always correlate with imipenem resistance, and differences in imipenem susceptibility could not be explained by oprD mutations or efflux pump genes (15). In our study, increased oprD levels were detected in 2 of the 18 imipenem-resistant P. aeruginosa clinical isolates analyzed. Also we could not find any relation between genotype and resistance pattern in AP-PCR study. Further studies will be necessary to understand the mechanisms underlying this apparent discordance between oprD levels and imipenem resistance.
While OprD porin proteins play an important role in carbapenem resistance in P. aeruginosa, this resistance cannot be explained by OprD levels alone, and other important interactions may influence carbapenem susceptibility. Characterization of carbapenem resistance mechanisms could provide additional therapeutic targets or allow for alternative strategies to enhance the efficacy of carbapenems.
References
-
1.
Hancock RE, Brinkman FS. Function of pseudomonas porins in uptake and efflux. Annu Rev Microbiol. 2002;56:17-38. [PubMed ID: 12142471]. https://doi.org/10.1146/annurev.micro.56.012302.160310.
-
2.
Nikaido H. Outer membrane barrier as a mechanism of antimicrobial resistance. Antimicrob Agents Chemother. 1989;33(11):1831-6. [PubMed ID: 2692513].
-
3.
Yoshimura F, Nikaido H. Diffusion of beta-lactam antibiotics through the porin channels of Escherichia coli K-12. Antimicrob Agents Chemother. 1985;27(1):84-92. [PubMed ID: 2580479].
-
4.
Strateva T, Yordanov D. Pseudomonas aeruginosa - a phenomenon of bacterial resistance. J Med Microbiol. 2009;58(Pt 9):1133-48. [PubMed ID: 19528173]. https://doi.org/10.1099/jmm.0.009142-0.
-
5.
Trias J, Nikaido H. Outer membrane protein D2 catalyzes facilitated diffusion of carbapenems and penems through the outer membrane of Pseudomonas aeruginosa. Antimicrob Agents Chemother. 1990;34(1):52-7. [PubMed ID: 2109575].
-
6.
Lister PD, Wolter DJ, Hanson ND. Antibacterial-resistant Pseudomonas aeruginosa: clinical impact and complex regulation of chromosomally encoded resistance mechanisms. Clin Microbiol Rev. 2009;22(4):582-610. [PubMed ID: 19822890]. https://doi.org/10.1128/CMR.00040-09.
-
7.
Performance Standards for Antimicrobial Susceptibility Testing; Twenty-First Informational Supplement. Wayne; Clinical and Laboratory Standards Institute. 2011.
-
8.
Savli H, Karadenizli A, Kolayli F, Gundes S, Ozbek U, Vahaboglu H. Expression stability of six housekeeping genes: A proposal for resistance gene quantification studies of Pseudomonas aeruginosa by real-time quantitative RT-PCR. J Med Microbiol. 2003;52(Pt 5):403-8. [PubMed ID: 12721316]. https://doi.org/10.1099/jmm.0.05132-0.
-
9.
Oh H, Stenhoff J, Jalal S, Wretlind B. Role of efflux pumps and mutations in genes for topoisomerases II and IV in fluoroquinolone-resistant Pseudomonas aeruginosa strains. Microb Drug Resist. 2003;9(4):323-8. [PubMed ID: 15000738]. https://doi.org/10.1089/107662903322762743.
-
10.
Pfaffl MW, Tichopad A, Prgomet C, Neuvians TP. Determination of stable housekeeping genes, differentially regulated target genes and sample integrity: BestKeeper--Excel-based tool using pair-wise correlations. Biotechnol Lett. 2004;26(6):509-15. [PubMed ID: 15127793].
-
11.
Xavier DE, Picao RC, Girardello R, Fehlberg LC, Gales AC. Efflux pumps expression and its association with porin down-regulation and beta-lactamase production among Pseudomonas aeruginosa causing bloodstream infections in Brazil. BMC Microbiol. 2010;10:217. [PubMed ID: 20704733]. https://doi.org/10.1186/1471-2180-10-217.
-
12.
Gales AC, Jones RN, Turnidge J, Rennie R, Ramphal R. Characterization of Pseudomonas aeruginosa isolates: occurrence rates, antimicrobial susceptibility patterns, and molecular typing in the global SENTRY Antimicrobial Surveillance Program, 1997-1999. Clin Infect Dis. 2001;32 Suppl 2:S146-55. [PubMed ID: 11320454]. https://doi.org/10.1086/320186.
-
13.
Mushtaq S, Ge Y, Livermore DM. Doripenem versus Pseudomonas aeruginosa in vitro: activity against characterized isolates, mutants, and transconjugants and resistance selection potential. Antimicrob Agents Chemother. 2004;48(8):3086-92. [PubMed ID: 15273124]. https://doi.org/10.1128/AAC.48.8.3086-3092.2004.
-
14.
Sakyo S, Tomita H, Tanimoto K, Fujimoto S, Ike Y. Potency of carbapenems for the prevention of carbapenem-resistant mutants of Pseudomonas aeruginosa: the high potency of a new carbapenem doripenem. J Antibiot (Tokyo). 2006;59(4):220-8. [PubMed ID: 16830889]. https://doi.org/10.1038/ja.2006.31.
-
15.
El Amin N, Giske CG, Jalal S, Keijser B, Kronvall G, Wretlind B. Carbapenem resistance mechanisms in Pseudomonas aeruginosa: alterations of porin OprD and efflux proteins do not fully explain resistance patterns observed in clinical isolates. APMIS. 2005;113(3):187-96. [PubMed ID: 15799762]. https://doi.org/10.1111/j.1600-0463.2005.apm1130306.x.
-
16.
Vatcheva-Dobrevska R, Mulet X, Ivanov I, Zamorano L, Dobreva E, Velinov T, et al. Molecular epidemiology and multidrug resistance mechanisms of Pseudomonas aeruginosa isolates from Bulgarian hospitals. Microb Drug Resist. 2013;19(5):355-61. [PubMed ID: 23600605]. https://doi.org/10.1089/mdr.2013.0004.
-
17.
Zeng ZR, Wang WP, Huang M, Shi LN, Wang Y, Shao HF. Mechanisms of carbapenem resistance in cephalosporin-susceptible Pseudomonas aeruginosa in China. Diagn Microbiol Infect Dis. 2014;78(3):268-70. [PubMed ID: 24359931]. https://doi.org/10.1016/j.diagmicrobio.2013.11.014.
-
18.
Fournier D, Richardot C, Muller E, Robert-Nicoud M, Llanes C, Plesiat P, et al. Complexity of resistance mechanisms to imipenem in intensive care unit strains of Pseudomonas aeruginosa. J Antimicrob Chemother. 2013;68(8):1772-80. [PubMed ID: 23587654]. https://doi.org/10.1093/jac/dkt098.
-
19.
Livermore DM. Of Pseudomonas, porins, pumps and carbapenems. J Antimicrob Chemother. 2001;47(3):247-50. [PubMed ID: 11222556].
-
20.
Brown SD, Traczewski MM. Comparative in vitro antimicrobial activity of a new carbapenem, doripenem: tentative disc diffusion criteria and quality control. J Antimicrob Chemother. 2005;55(6):944-9. [PubMed ID: 15872043]. https://doi.org/10.1093/jac/dki134.
-
21.
Cabot G, Ocampo-Sosa AA, Tubau F, Macia MD, Rodriguez C, Moya B, et al. Overexpression of AmpC and efflux pumps in Pseudomonas aeruginosa isolates from bloodstream infections: prevalence and impact on resistance in a Spanish multicenter study. Antimicrob Agents Chemother. 2011;55(5):1906-11. [PubMed ID: 21357294]. https://doi.org/10.1128/AAC.01645-10.