Abstract
Background:
Liposuction nowadays is the most popular way of weight loss and body contouring. It is a mechanical procedure to reduce fat through the back-and-forth movement of a heavy metal called cannula. The thick cannula, essential for efficient fat removal and subsequent suctioning, causes some undesirable effects such as bruise, scars, heavy blood loss, skin laxity and long-run recovery. While, the new method of liposuction using a 100-300 µm fiber laser inserted into a thin cannula of 1 mm diameter causes less distress and bleeding. Simultaneous interaction between laser radiation and tissue causes faster skin tightening and coagulation of small blood vessels.Objectives:
The study aimed at evaluating the effects of these highly important parameters of lipolysis operations.Materials and Methods:
In this study, penetration depth and tissue heating following laser irradiation with a 980 nm diode laser were investigated. This laser is different from 1064 nm Nd:YAG in terms of absorption and scattering coefficient. Hence, dissimilar results for beam penetration and tissue heating were expected. Monte Carlo method was used to simulate radiation (photons) propagation in tissues. Using such simulation can be useful in evaluating penetration depth, absorption, scattering and reflection of the photon within the tissue and across the tissue borders. Temperature rise was simulated using Comsol Multiphysics software.Results:
The simulation results showed that the penetration depth and temperature rise of 980 nm wavelengths were different from those of 1064 nm wavelength. It appeared that 1064 nm wavelength penetrated to deeper layers of tissue compared with 980 nm. Moreover, temperature rise during 1064 nm irradiation led to temperature increase in allowable ranges. The findings proved the reason why 1064 nm wavelength are commonly used in laser lipolysis in comparison with 980 nm wavelength.Conclusions:
The simulation indicated that temperature rise in 980 nm wavelength was 70.802 ℃, which was higher than that of 1064 nm wavelength. Therefore, 980 nm laser can leave unwanted negative effects on tissues including hyperthermia.Keywords
1. Background
Using lasers as complementary tools in liposuction is a novel method. It first introduced in 1994 which is now among the most popular cosmetic surgeries. Common problems with traditional methods - some even with side effects - have made it necessary to choose safer procedures and it appears that laser lipolysis can meet these increasingly important demands. Lipolysis is a 100-300 µm fiber laser inserted into a thin cannula of 1 mm diameter, which not only create smaller incisions but also is less traumatic. In comparison with old methods, in the new procedures the coagulation of small blood vessels and skin retraction result from the interaction of laser radiation with tissue, causing less bleeding and faster skin tightening (1, 2). Using lasers as auxiliary devices in lipolysis has limited the use of traditional liposuction methods. In this new method, lasers are utilized to produce the desired damages and effects on the tissue. Interaction of laser with tissue cells can have many effects, most of which improve the downsides of the conventional methods. Safety and efficacy of conventional liposuctions have also been enhanced for the new ones by using thinner cannulas, which not only make smaller incisions but also increase the coagulation process of small blood vessels through interaction between laser and tissues. Laser lipolysis also results in selective tissue heating leading to have less thermal damages to target tissues. Such interaction also promotes hemostasis, injury healing and postoperative recovery (2).
Laser lipolysis has a wavelength-dependent mechanism. First, laser radiation is absorbed by fat cells and receptive chromophores, the absorbed radiation then transforms into heat and produces the desired thermal effects. Heat acts on fat cells and extracellular matrix to compensate both reversible and irreversible damages, which also facilitate the process of liposuction by having fewer traumas as well as less bleeding. The absorption of radiation in tissue depends strongly on absorption coefficient. The coefficient for 980 nm diode laser is 100 µm and nearly different from that of 1064 nm Nd:YAG laser. It is expected that 980 nm diode laser produces distinct thermal effects comparing with 1064 nm Nd:YAG laser (2, 3).
Laser lipolysis is a new technique which is still under development. It indemnifies the conventional liposuction methods concerning tissue damaging and hemostasis effects. Notably, the efficacy of laser lipolysis depends on the interaction between laser and tissue. In addition, understanding its mechanism has a vital role in choosing suitable laser wavelengths and energy doses. In laser lipolysis, one can evaluate the thermal effects and damages on tissue as well as the required energy dose for achieving desirable outcome by numerical simulation of laser-tissue interaction and that is one of many advantages of this method over the traditional liposuction. It is worth mentioning that simulation of laser-tissue interaction provides enormous research opportunities for the researches in this field.
2. Objectives
Laser lipolysis has a purely wavelength-dependent nature. Penetration depth of the radiation into tissue is directly proportional to radiation wavelength. Tissue heating also depends on radiation power and the duration laser radiation used. These are the aims the study seek to examine; the effects of these highly important parameters on lipolysis operations.
4. Results
In Figure 1, the absorption versus penetration depth for the two wavelengths is presented. The findings of our simulation confirmed the expected differences between the penetration depth of the two wavelengths as the result of having different absorption and scattering coefficients. The outcomes showed that penetration depths for 980 nm diode lasers and 1064 nm Nd:YAG lasers were 2.65 and 3.1 mm, respectively. The results implied that absorption and scattering coefficient were the key factors in laser lipolysis, since they determined the penetration depth of laser radiations inside the tissue (1-7). Deeper penetration in 1064 nm Nd: YAG offered the preference of this wavelength to 980 nm, as the former propagated deeper in the fat tissues. Absorption coefficient of 1064 nm wavelength was shorter than that of 980 nm, consequently direct heating volume in 1064 nm was larger. Furthermore, skin retraction and tissue tightening with 1064 nm wavelength were obtained more efficiently in superficial treatment (1) due to the penetration of radiation to dermal layers and initiation of collagen gel retraction (CGR). The latter is one of the most important features of laser lipolysis causing skin retraction which leads to the decrease in skin laxity following fat removal operations.
Fluence is another major factor in laser-tissue interaction. It is defined as energy conveyed per unit area and the dimension is Joule/m2. Fluence can affect laser lipolysis in different ways. Given that, the effects of increasing and decreasing fluence on penetration depth and radiation absorption in the tissue were studied. First, powers of the lasers were increased to 10, 20 and 30 watt for both wavelengths, then achieved results of the simulation were compared. The outcomes again revealed that 1064 nm wavelength could penetrate to deeper layers of fat tissue. Figure 2 and Figure 3 depicted the absorption versus the penetration depth for 1064 nm and 980 nm, respectively. It appeared that the deepest penetration for both wavelengths occurred in power of 30 watt which was equal to 3.2 mm for 1064 nm and 2.8 mm for 980 nm. Accordingly, increasing laser fluence for having deeper penetration was obtained more efficiently in 1064 nm wavelength.
This time, the effect of decreasing laser fluence on radiation penetration was scrutinized. To this end, radius of the laser beams was increased up to a fixed power of 10 watt, which caused fluence reduction. The beam radius was increased from the initial value of 200 µm to 282.84 and 346.41 µm for both wavelengths. We apply the influence of increasing of beam radius as increasing of the FWHM (full width at half maximum). In Figure 4 and Figure 5, the absorption versus the penetration depth for 1064 and 980 nm wavelengths are illustrated, respectively. The results indicated slight difference between the two wavelengths. Maximum penetration depth for 1064 nm wavelength occurred in 200 µm beam radius which was equal to 3 mm. Similarly, the deepest penetration for 980 nm happened in 200 µm; equal to 2.5 mm due to the influence of absorption coefficient of fat tissues. As the radius of laser beam increased, radiation spread over wider areas in tissue and was immediately absorbed by tissue cells, led to reduction in penetration depth for both 1064 and 980 nm wavelengths.
As mentioned above, 980 nm wavelength of diode laser and 1064 nm of Nd:YAG laser had almost different absorption and penetration depths, hence these wavelengths showed different impacts on laser-tissue interaction. Results of the simulation verified the hypotheses; the wavelengths had different behaviors towards fat tissues. This is why nowadays 1064 nm wavelength is widely used in laser lipolysis instead of 980 nm diode lasers (1-3). Having had more penetration depth in tissue, 1064 nm wavelength had larger directly-heated volume. Its absorption coefficient was smaller than 980 nm, as the result laser beam perforated deeper to the tissue and heated larger . As the laser beam for 980 nm wavelength had greater absorption; the radiation therefore was accumulated in smaller volume, caused significant increase in the temperature for low volume tissues and resulted in unwanted damages to tissue such as bruise and hyperthermia.
In this stage, simulation of the tissue heating and temperature rise of the fat tissues following the irradiation of both 980 nm and 1064 nm wavelengths was analyzed. The results of the tissue heating for 1064 and 980 nm wavelengths are presented in Figure 6 and Figure 7, respectively. The issue was heated up to 339.687 k for irradiation of 1064 nm Nd:YAG laser. The simulation of the tissue heating for the 980 nm diode laser showed that tissue temperature rose to 343.952 k. To better comprehend the results of tissue heating, the following figures of cannula insertion to the tissue surface were designed. In Figure 8, the temperature rise is seen for the location of laser radiation of 1064 nm Nd:YAG in the tissue; 1 cm below the tissue surface. It clearly shows the temperature rises up to 66℃ in the site of laser radiation. This temperature was sufficient for achieving the desired reversible and irreversible effects of fat tissues. The results of this simulation indicated different temperature rise for the two wavelengths. Our findings were in agreement with the literature as well as experimental studies (1, 2). These studies confirmed that 1064 nm wavelength was suitable for efficient laser lipolysis and had less unwanted effects on tissue. Lukac affirmed that radiation of 1064 nm could produce the authorized temperatures needed for lipolysis (3). Most researches agreed that the temperature range of 50-65℃ was the clinical endpoint for the applicability of laser lipolysis. In other words, the temperature range were essential to obtain the desired tissue damages. Besides, they were also sufficient to trigger the favorable thermal effects which could have the desired reversible and irreversible damages. Temperatures below 50-65℃ were not enough to create the desired damages and temperatures higher than that would result in unfavorable effects such as bruise and hyperthermia (2, 3). The findings of the simulation proved the preference of 1064 nm over the 980 nm wavelength in many ways. The penetration depth of 1064 nm was deeper and it was also absorbed in larger volume of the fat tissues. This wavelength also resulted in better skin tightening and fat reduction. In contrast, as 980 nm had greater absorption coefficient, it caused less penetration and higher temperature rise in shallow parts of the tissue, possibly caused side effects such as necrosis, bruise and hyperthermia.
Penetration Depth for Wavelengths Under Study
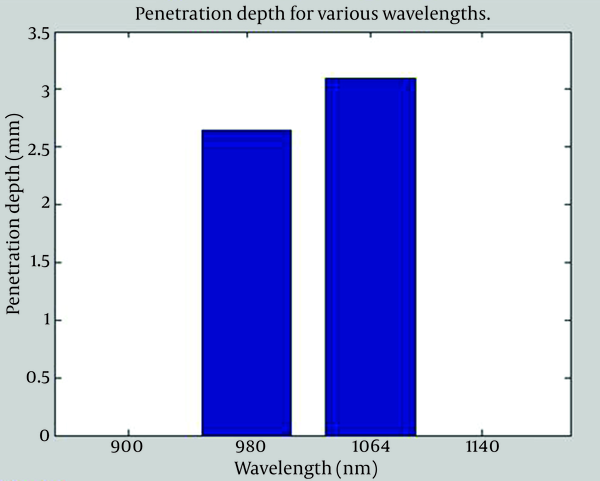
Absorption Versus Penetration Depth for Various Powers of 1064 nm Wavelength and Fixed Beam Radius of 200 µm
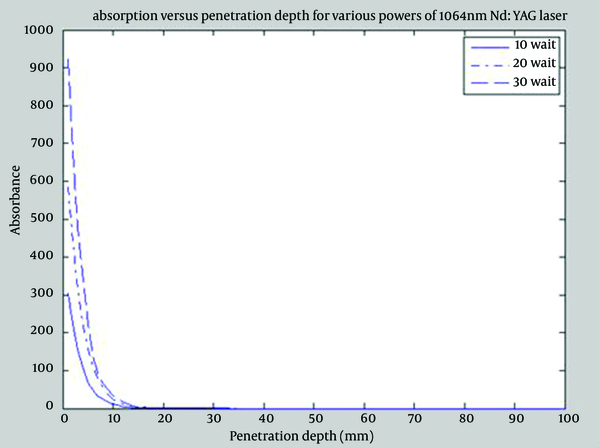
Absorption Versus Penetration Depth for Various Powers of 980 nm Wavelength and Fixed Beam Radius of 200 µm
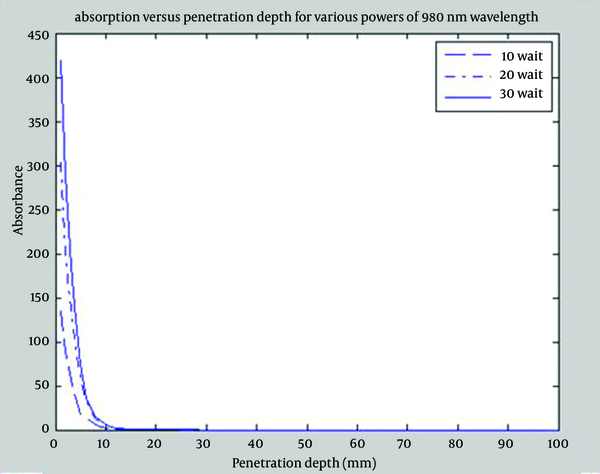
Absorption Versus Penetration Depth for Various Radiuses of 1064 nm at Fixd Power of 10 Watt
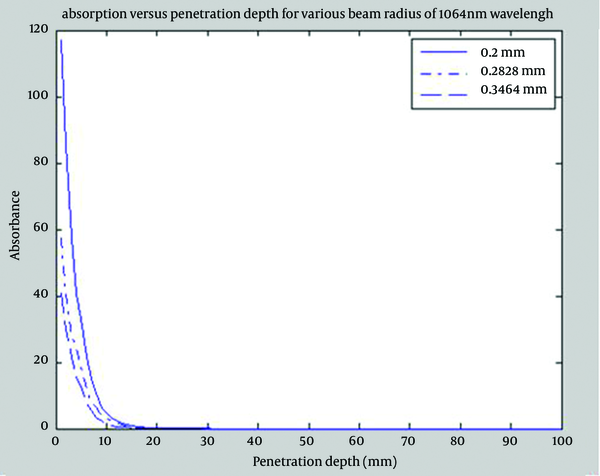
Absorption Versus Penetration Depth for Various Beam Radiuses of 980 nm at Fixed Power of 10 Watt
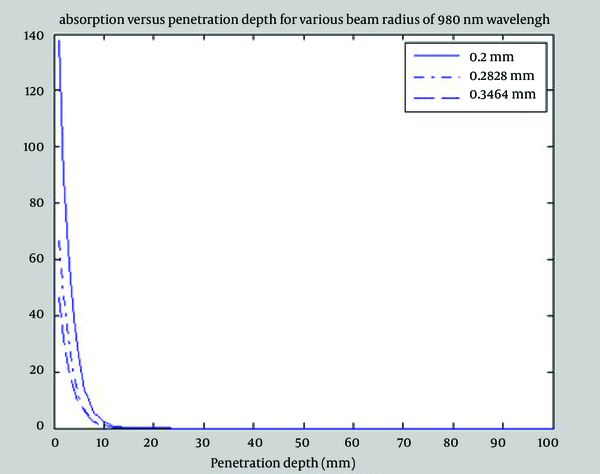
Temperature Rise Following the Irradiation of 1064 nm Wavelength at Power of 15 Watt
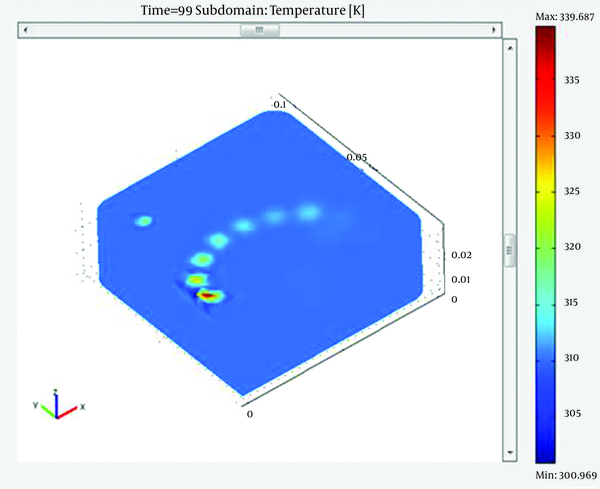
Temperature Rise Following the Irradiation of 980 nm Wavelength at Power of 15 Watt
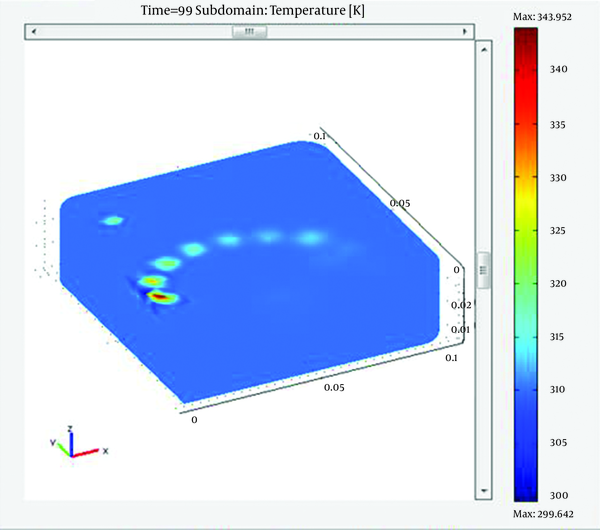
Temperature Rise of Fat Tissue After Radiation of 1064 nm Wavelength at the Depth of 1 cm Below the Tissue Surface
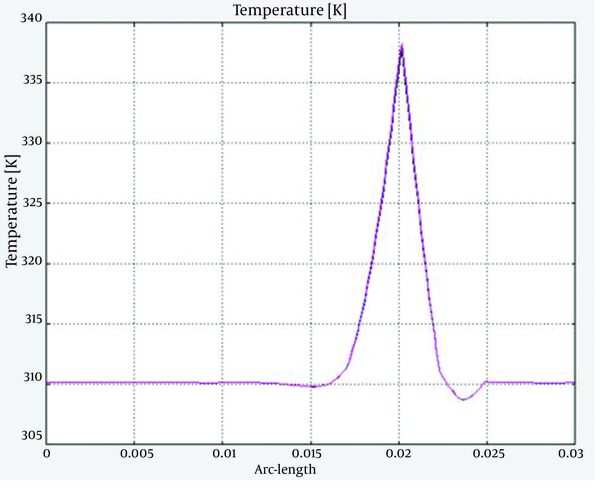
5. Discussion
As an innovative technique to remove excess body fat, liposuction is now among the most popular cosmetic surgeries. Yet, this method suffers some adverse effects, some of which are critically problematic. With the increasing demand to lose weight, it is vital to improve liposuction and discuss the existing deficiencies. Laser lipolysis is a response to this existing demand as it produces the desirable thermal effects and causes less tissue damages with simultaneous collagen retraction and vessel coagulation, resulted in quicker skin tightening and less bleeding (1-3). The findings of our simulations indicated some differences between the performance of 1064 nm and 980 nm wavelengths as they had nearly diverse absorption and scattering coefficients for fat tissues. Monte Carlo simulation results showed that these wavelengths did not have similar penetration depth in the fat tissue as proposed earlier, because the mean free path of the photons in the tissue was inversely proportional with absorption coefficient. Moreover, as 1064 nm wavelength had smaller absorption coefficient than 980 nm, it penetrated deeper into the fat tissues and could be more effectively used in laser lipolysis. Smaller absorption coefficient made the radiation propagate deeper in the tissue, consequently the laser energy accumulated in larger volume and lipolysis were performed more efficiently. Besides, penetration of radiation into the dermal layer in superficial treatment with 1064 nm resulted in collagen retraction, made skin tightening achievable using 1064 nm Nd:YAG laser. These remarks verified the priority of 1064nm wavelength over the 980 nm and are the reasons why nowadays 1064 nm Nd:YAG lasers are widely used in laser lipolysis. Results of the tissue heating simulation using the Comsol Multiphysics showed different temperature rise following the radiation with the wavelengths of 980 nm and 1064 nm (1). The results of our simulation offered that 1064 nm wavelength be could be successfully employed in laser lipolysis. The findings of our simulation showed that tissue heated up to 339.687 k or 66.537℃, which was sufficient to initiate the desired tissue damages. Experience has shown that the thermal damages on fat tissue occur in approximate temperature range of 50-65℃ (1, 3). Most researchers unanimously believe that this temperature range is clinical endpoints for laser lipolysis. Higher temperatures would cause negative effects such as bruise and necrosis. Beside, lower temperatures do not effectively cause destruction of adipocytes (lipolysis) (1-3). Our simulation indicated that temperature rise in 980 nm wavelength was 70.802℃ which was higher than the temperature range mentioned before. Accordingly, this laser gave rise to unwanted side effects on tissue including hyperthermia. All in all, 1064 nm wavelength of Nd:YAG laser are widely used in laser lipolysis and as our findings also suggested low powers of 980 nm diode laser can be used as alternative, because with lower power one can control the temperature rise and avoid the hyperthermia (1).
Acknowledgements
References
-
1.
Mordon SR, Wassmer B, Reynaud JP, Zemmouri J. Mathematical modeling of laser lipolysis. Biomed Eng Online. 2008;7:10. [PubMed ID: 18312643]. https://doi.org/10.1186/1475-925X-7-10.
-
2.
Badin AZ, Gondek LB, Garcia MJ, Valle LC, Flizikowski FB, de Noronha L. Analysis of laser lipolysis effects on human tissue samples obtained from liposuction. Aesthetic Plast Surg. 2005;29(4):281-6. [PubMed ID: 16075355]. https://doi.org/10.1007/s00266-004-0102-9.
-
3.
Lukac M, Vizintin Z, Zabkar J, Pirnat S/. QCW pulsed Nd: YAG 1064 nm laser lipolysis. J Laser Health Acad. 2009;4(1):24-34.
-
4.
Niemz MH. Laser-tissue interactions: fundamentals and applications. Germany: Springer; 2007.
-
5.
Wang L, Jacques SL, Zheng L. MCML--Monte Carlo modeling of light transport in multi-layered tissues. Comput Methods Programs Biomed. 1995;47(2):131-46. [PubMed ID: 7587160].
-
6.
Guo Z, Aber J, Garetz BA, Kumar S. Monte Carlo simulation and experiments of pulsed radiative transfer. J Quant Spectrosc Radiat Transf. 2002;73(2):159-68. https://doi.org/10.1016/S0022-4073(01)00203-5.
-
7.
Prahl SA, Keijzer M, Jacques SL, Welch AJ. A Monte Carlo model of light propagation in tissue. Dosim Laser Radiat Med Biol. 1989;5:102-11.