Abstract
Background:
The development of dental caries around orthodontic brackets is a common complication of orthodontic treatment.Objectives:
This study aimed to compare the antibacterial efficacy of stainless steel (SS) brackets coated with copper oxide nanoparticles (CuO-NPs), titanium dioxide nanoparticles (TiO2-NPs), and hydroxyapatite silver nanoparticles (HA-SNPs) against Streptococcus mutans.Methods:
In this in vitro, experimental study, 20 orthodontic brackets were assigned to four groups (n = 5, each group). One group served as the no-intervention control group, and the brackets in the remaining three groups underwent dip coating with CuO-NPs, TiO2-NPs, and HA-SNPs < 100 nm. The number of S. mutans colonies was counted after 24 h, one week, and one and three months. Data were analyzed using the Shapiro Wilk test, repeated measures ANOVA, and Bonferroni multiple comparisons test.Results:
All three experimental groups exhibited similar antibacterial properties after 90 days of trial (P > 0.05). Moreover, CuO-NPs had a greater inhibitory effect than TiO2-NPs on S. mutans after 24 h (P = 0.007). However, the inhibitory effect of HA-SNPs was not significantly different from that of TiO2-NPs (P = 0.259) or CuO-NPs (P = 0.224).Conclusions:
Considering the similar antibacterial properties of all three coatings in the long term, all three types of nanoparticles can be used to coat orthodontic brackets to prevent caries. However, due to the high cost and difficult preparation of HA-SNPs and the slightly higher efficacy of CuO-NPs in the short-term, the latter may be preferred for this purpose.Keywords
1. Background
Dental caries are a concern common in orthodontic treatments (1-4). Such caries describe an infectious condition depending on several factors, such as the microbial flora of the oral cavity, the accumulation of fermentable carbohydrates on tooth surfaces, and adequate time for microbial activities (5). The placement of orthodontic appliances in the oral cavity increases food impaction (6); hence, bacterial species in the oral environment have a greater, and longer access to fermentable carbohydrates in foods, , thereby promoting their proliferation and activity (3, 4, 6, 7). Streptococcus mutans is a Gram-positive facultative anaerobe in the oral environment and the leading cause of dental caries (2, 7). It ferments the sucrose and produces lactic acid, damaging the enamel (2, 8).
Several strategies can be adopted to prevent caries during orthodontic treatment such as correct tooth brushing, and using fluoride gel and mouthwash, the effectiveness of which depends on patients’ cooperation and their oral hygiene quality (8, 9). Despite observing oral hygiene and using some adjuncts such as fluoride gel, evidence has documented a higher rate of caries in patients under orthodontic treatment compared to non-orthodontic controls (3, 4, 6, 7).
Recently, organic materials containing metals (namely silver, copper, titanium, and zinc) have been used as antibacterial agents. Copper and silver have long been used as antimicrobial agents (2, 4). In this regard, the release and antibacterial activity of copper particles in different shapes and sizes have been addressed in previous studies. The release rate of copper oxide (CuO) and copper nanoparticles is higher than the release rate of copper in mass form. Moreover, the nanoparticulate form has higher antimicrobial activity.
On the other hand, the CuO nanoparticles (CuO-NPs) damage DNA, further adding to its antibacterial activity. Moreover, the release rate of CuO-NPs is higher than that of copper nanoparticles (10). Silver nanoparticles (SNPs) can affect the cells’ respiratory cycle; the respiratory cycle in different microbial species decreases by an increase in the concentration of SNPs (11). SNPs eliminate microorganisms by different mechanisms (12). Titanium dioxide (TiO2) also has different applications due to its antimicrobial properties (13). Since the early 20th century, the following two different roles of TiO2 are confirmed: oxidation-reduction, and the surface modification of materials by conferring hydrophilicity to them. TiO2 can damage the microbial cell membrane by producing free radicals (14).
Hydroxyapatite (HA) is a crystalline calcium phosphate compound with numerous applications in medicine and dentistry since it has properties similar to those of the mineral part of the hard tissue in the human body (e.g., biocompatibility and low solubility in wet environments) (15-17). Antibacterial activity is another favorable property of HA. Evidence shows that HA can limit the proliferation of S. mutans and other oral pathogens (6).
2. Objectives
Nanoparticles have the lowest rate of toxicity in the environment; hence, they can be used to eliminate pathogenic microorganisms (18, 19). Accordingly, coating orthodontic brackets with nanoparticles may prevent dental caries. Several studies have evaluated the antimicrobial activity of various nanoparticles coated on orthodontic wires and brackets; however, no study has compared them at different time intervals. Moreover, the effect of combining HA-SNPs against S. mutans has not been studied yet (20-22). Accordingly, this study aimed to compare the effects of coating orthodontic brackets with CuO-NPs, TiO2-NPs, and HA-SNPs on S. mutans.
3. Methods
After receiving the code of ethics from the Ethics Committee of the Semnan University of Medical Sciences, the implementation of the project initiated. Twenty 022 slot stainless steel (SS) orthodontic brackets of Roth system (American Ortho, USA) were assigned to four groups (n = 5) using a table of random numbers. Orthodontic brackets in the three experimental groups were coated with CuO-NPs, TiO2-NPs, and HA-SNPs. The five brackets in the control group underwent no intervention.
To coat brackets with antibacterial nanoparticles, the size of nanoparticles was first measured using a scanning electron microscope (DSM-960A; Zeiss, Germany) (Figure 1). Then CuO-NP, TiO2-NP (< 100 nm; synthesized by the laboratory of the Faculty of Physics of the Tehran University), and HA-SNP (synthesized by the Tehran Atomic Energy Organization) coatings were applied on the surface of SS brackets using the dip-coating technique. Atomic force microscopy (AFM; ENTEGRA AFMNT – MDT; NT-MDT, Russia) was used to measure the thickness of nanoparticle coatings. To determine the antibacterial activity of nanoparticle coatings, S. mutans was first obtained from the microbial bank of the Microbiology Laboratory of the Shahid Beheshti University of Medical Sciences and then cultured. In the next step, microbial suspension with 0.5 McFarland standard concentration was prepared. Each bracket was placed into a test tube containing S. mutans suspension with 0.5 McFarland standard concentration for 24 h, one week, and one and three months. Afterward, the brackets were transferred to another test tube containing sterile Mueller Hinton broth for two hours. After two hours, 0.001 cc of each tube’s contents was cultured on Mueller Hinton agar culture medium. The culture medium was then incubated at 37°C, and the number of colonies was counted after 24 h, one week, one month, and three months. Each colony dot was also counted once. Our approach was to set the Petri dish on a grid background and count the colonies in each grid cell, moving in a methodical pattern through all cells. We marked counted colonies on the back of the Petri dish to reduce the likelihood of mistakes. After counting the colonies on each plate, the mean frequency of colonies in each group of brackets was calculated by adding the colony counts of relevant plates divided by 5 (number of brackets in each group). Data were analyzed with SPSS software version 23.0 using the Shapiro-Wilk test, repeated measures ANOVA, and Bonferroni test for multiple comparisons at P = 0.05.
Size measurement of nanoparticles by scanning electron microscope
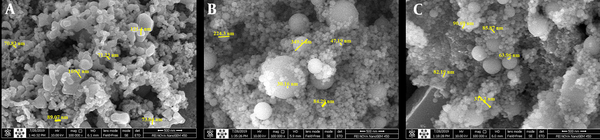
4. Results
4.1. Streptococcus mutans Colony Count
Repeated measures ANOVA revealed the significant interaction effect of type of bracket coating and measurement time on S. mutans colony count [P = 0.026, F (2.5 and 15.3):4.3]. Table 1 shows the mean and standard deviation of S. mutans colonies at each time point for different groups. The Bonferroni test showed a significant difference between different groups at different time points. At all time-points, the colony count in the control group was not possible due to the large frequency of S. mutans colonies, which was significantly greater than the frequency of all studied nanoparticles (P > 0.05).
Quantity of Streptococcus mutans Colonies in Experimental Groups Across Four-Time Points a
Coatings | No. | Time Points | |||
---|---|---|---|---|---|
Day 1 | Day 7 | Day 30 | Day 90 | ||
TiO2 | 5 | (47.6 × 1000) ± 8.6 | (472 × 1000) ± 19.3 | (554 × 1000) ± 47.8 | (858 × 1000) ± 115.8 |
CuO | 5 | (10.4 × 1000) ± 6.2 | (628.4 × 1000) ± 64.3 | (788 × 1000) ± 54.2 | (1014 × 1000) ± 91.1 |
HA-SNP | 5 | (29.4 × 1000) ± 5.5 | (515 × 1000) ± 45.6 | (548 × 1000) ± 33.7 | (1500 × 1000) ± 266.0 |
4.1.1. 24 h
Comparing S. mutans colony count in the three experimental groups at 24 h showed that the mean colony count was significantly lower in the CuO-NP group than the TiO2-NP group (P = 0.007). However, no significant difference was noticed between TiO2-NP and HA-SNP groups regarding the mean colony count (P = 0.259). Furthermore, the difference between CuO-NP and HA-SNP groups was not statistically significant (P = 0.224; Table 2).
Ferquency Changes of Streptococcus mutans Colonies Between the Experimental Groups at Different Times
Coating Groups | Time Points | |||||||
---|---|---|---|---|---|---|---|---|
Day 1 | Day 7 | Day 30 | Day 90 | |||||
Mean ± SD | P-Value | Mean ± SD | P-Value | Mean ± SD | P-Value | Mean ± SD | P-Value | |
TiO2-NPs | ||||||||
CuO-NPs | 37.2 ± 9.3 | 0.007 | -156.0 ± 6.63 | 0.108 | -234.0 ± 81.8 | 0.011 | -156.0 ± 177.9 | 1.00 |
HA-SNPs | 18.2 ± 8.6 | 0.259 | -43.0 ± 7.45 | 1.00 | 6.0 ± 72.4 | 1.00 | -642.0 ± 185.5 | 0.071 |
HA-SNPs | ||||||||
CuO-NPs | 19.0 ± 8.7 | 0.224 | -113.4 ± 0.83 | 0.338 | -240.0 ± 60.5 | 0.009 | 486.0 ± 311.6 | 0.222 |
4.1.2. Seven Days
Comparison of S. mutans colony count in the three experimental groups after one week showed no significant difference between the groups (P > 0.05, Table 2).
4.1.3. One Month
The mean colony count was significantly lower in the TiO2-NP group than in the CuO-NP group (P = 0.011). Moreover, the mean colony count was significantly lower in the HA-SNP group than in the CuO-NP group (P = 0.009). However, the mean colony count difference between the TiO2-NP and HA-SNP groups was not significant (P = 1.00, Table 2).
4.1.4. Three Months
The mean colony count was not significantly different among the three groups (P > 0.05). However, the mean colony count in the three experimental groups was significantly different from the control group (P < 0.001). Accordingly, all three coatings preserved their antibacterial activity after three months (P > 0.05, Table 2 and Figure 2).
Colonies grown on agar culture medium: A, Control group; B, TiO2; C, CuO; D, Silver hydroxyapatite.
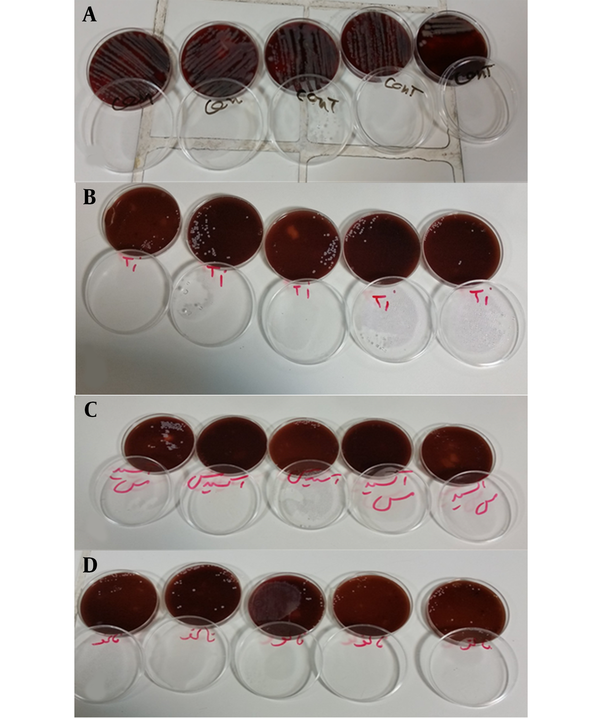
4.2. Within-Group Comparisons
4.2.1. TiO2-NPs
The mean colony count in the TiO2-NP group was significantly higher on day 7 vs. day 1 (P < 0.001); however, the mean colony counts were not significantly different on day 30 vs. day 7 (P = 1.00) and day 90 vs. day 30 (P = 0.596, I).I).
4.2.2. CuO-NPs
The mean colony count in the CuO-NP group significantly increased on day 7 vs. day 1 (P < 0.001). However, the mean colony count were not significantly different on day 30 vs. day 7 (P = 0.239) or day 90 vs. day 30 (P = 1.00, I).I).
4.2.3. HA-SNPs
The mean colony count in the HA-SNP group was significantly higher on day 7 vs. day 1 (P < 0.001). However, the mean colony counts were not significantly different on day 30 and day 1 (P = 1.00). The mean colony count significantly increased on day 90 compared to day 30 (P = 0.001, Table 1).
5. Discussion
This study assessed the antibacterial activity of CuO-NP, TiO2-NP, and HA-SNP coatings of SS orthodontic brackets against S. mutans at four-time points. Previous studies have assessed the effect of different sizes of one type of nanoparticle and different nanoparticles at different time points against different microbial species (2, 4, 23-26). The present study assessed the antibacterial effects of nano-coatings on S. mutans after 24 h, one week, and one and three months. The results revealed a significantly lower frequency of S. mutans colonies in each experimental group compared to the control group, indicating the optimal antibacterial activity of all three nano-coatings against S. mutans. The comparative results of counts after 24 h indicated that CuO-NPs had maximum antibacterial activity against S. mutans, and TiO2-NPs had minimum antibacterial activity in the short-term (24 h).
The results obtained on day 30 indicated the increasing effects of TiO2-NP and HA-SNPs on S. mutans, which reached the maximum value on day 30. The results on day 90 showed similar antibacterial activities of the three nanoparticles in the long term; however, the antibacterial activity of TiO2-NP and HA-SNPs against S. mutans decreased after day 30.
Ramazanzadeh et al. (2015) evaluated the antibacterial effects of CuO and ZnO nanoparticles on S. mutans (27). Similarly, their results confirmed the antibacterial activity of CuO. They also reported the superior antibacterial activity of CuO compared to ZnO, which may be due to different molecular sizes of copper and zinc. In the present study, CuO-NPs showed higher antibacterial activity than HA-SNPs and TiO2-NPs after 24 hours. Metin-Gürsoy et al. (25) also confirmed the antimicrobial activity for the nano-silver coating of orthodontic brackets against S. mutans, which was in agreement with our results. They demonstrated that orthodontic brackets coated with nano-silver inhibited S. mutans and decreased smooth-surface caries during 30 days.
Ruparelia et al. (2008) evaluated the antimicrobial effects of SNPs and copper nanoparticles against different bacterial species. They concluded that different microbial species were sensitive to nanoparticles at different levels, and that SNPs had antibacterial activity against a larger frequency of microbial species than copper nanoparticles (26). This finding can be due to the smaller size of silver molecules than copper molecules, and, consequently larger surface/volume ratio of silver compared to copper. However, the antimicrobial efficacy of CuO-NPs and HA-SNPs was the same after 24 hours, one week, and three months in the present study, which may be due to the chemical interactions of these nanoparticles with oxygen and HA. The antimicrobial activity of CuO-NPs is higher than that of copper nanoparticles, and HA-silver molecules are larger than nanosilver particles. Rai et al. (2009) (28) studied the antimicrobial properties of SNPs against antibiotic-resistant microorganisms and confirmed their optimal effectiveness for this purpose. Ciobanu et al. (2013) evaluated the antimicrobial activity of silver-doped HA nanoparticles against Gram-positive, Gram-negative bacteria, and fungi using qualitative tests. They reported that silver-doped HA nanoparticles acted efficiently against different microbial targets in different forms (17). This finding was probably due to the differences in the structure of microbial cell walls. Espinosa-Cristóbal et al. (2018) evaluated the antimicrobial and anti-adhesive properties of the SNP coatings of orthodontic brackets in two sizes against S. mutans (2). Similar to the present study, they showed that all types of SNPs inhibited the adhesion of S. mutans. However, smaller particles caused greater inhibition than larger particles, which can be due to the larger surface/volume ratio of smaller nanoparticles, resulting in their superior efficacy compared to larger particles. Salehi et al. (2018) assessed the antimicrobial activity of brackets coated with TiO2 by nitrogen in the long term and demonstrated the optimal effectiveness of brackets coated with TiO2 in inhibiting S. mutans and preventing enamel decalcification during 90 days (4). In the present study, the mean number of colonies in the TiO2-NP group was significantly higher on day 7 that on day 1; however, the difference between day 7 and day 30, or day 30 and day 90 was not statistically significant. This could be explained by the accelerated release of the Ti nanoparticles into the environment, affecting the accumulation of S. mutants.
Coating brackets with nanoparticles also affects antimicrobial activity. Nanoparticle coating of surfaces refers to creating a nanoscale layer on the surface by using different techniques such as dip-coating. The coating thickness in the dip-coating technique can be as thin as a monolayer of molecules. The dip-coating technique was used to coat brackets in the present study. Meyer-Kobbe et al. (2019) assessed the effects of intraoral biofilm reduction on brackets using three different methods of galvanic technique, physical vapor deposition, and plasma immersion ion implantation and deposition for SNP coating (29). They evaluated the effect of different coating methods of SNPs on their bactericidal property and biofilm formation and reported that only one coating method increased the bactericidal property of SNPs, which can be due to the change in the behavioral properties of SNPs during coating. In the present study, HA-SNPs applied by dip-coating decreased S. mutans count at different time points. This might be caused by the bactericidal or bacteriostatic effect of HA-SNPs. Ferrando-Magraner et al. (2020), in a systematic review, studied the antibacterial properties of nanoparticles in dental restorative materials (30). They concluded that adding nanoparticles to any dental restorative material increases its antibacterial properties. The present study also confirmed the antimicrobial activity of all three tested nanoparticle types. In their study, the antibacterial property of TiO2-NPs was higher than that of other nanoparticle types. In contrast, the present study revealed that all three nanoparticle coatings had a similar antibacterial performance in the long term, which can be due to in vitro design and the use of coated brackets. Carrouel et al. (2020) evaluated the antimicrobial, anti-inflammatory, and remineralizing properties of nanoparticles in dental materials (31). Nanoparticles in dental products such as toothpaste and mouthwashes confer antimicrobial, anti-inflammatory, and remineralizing properties. In line with the present study, Carrouel et al. confirmed the antimicrobial activity of nanoparticles such as TiO2, which might be due to the fast release of nanoparticles into the environment. Kotta et al. (2020) compared the antimicrobial characteristic of lingual retainers bonded with conventional composite and nanoparticle-containing composite (32). In agreement with the present study, they confirmed that the TiO2 composite group showed statistically more significant antibacterial activity without compromising the bond strength.
Difficulty in determining the size of nanoparticles under a scanning electron microscope, difficult coating of brackets, and difficult measurement of the thickness of nanoparticle coatings were among the limitations of this study.
CuO-NPs, TiO2-NPs, and HA-SNPs showed similar antibacterial activity against S. mutans under in vitro conditions in the long term. However, in the short-term (24 h), CuO-NP had a greater inhibitory effect on S. mutans than TiO2-NP and HA-SNPs. Accordingly, it can be used for coating appliances only at specific times and for a short period. Future studies are recommended to assess the antibacterial properties of HA-SNPs, CuO-NPs, and TiO2-NPs incorporated in composite resins used for bracket bonding under clinical conditions and applied as a coating on different bracket types.
5.1. Conclusions
Considering the relatively similar antibacterial properties of all three coatings in the long term, all three types of nanoparticles can be used for coating orthodontic brackets to reduce caries in patients undergoing orthodontic treatment.
Acknowledgements
References
-
1.
Brook PH, Shaw WC. The development of an index of orthodontic treatment priority. Eur J Orthod. 1989;11(3):309-20. [PubMed ID: 2792220]. https://doi.org/10.1093/oxfordjournals.ejo.a035999.
-
2.
Espinosa-Cristóbal LF, López-Ruiz N, Cabada-Tarín D, Reyes-López SY, Zaragoza-Contreras A, Constandse-Cortéz D, et al. Antiadherence and Antimicrobial Properties of Silver Nanoparticles against Streptococcus mutans on Brackets and Wires Used for Orthodontic Treatments. J Nanomater. 2018;2018:1-11. https://doi.org/10.1155/2018/9248527.
-
3.
Zhang R, Zhang W, Bai X, Song X, Wang C, Gao X, et al. Report: Discussion on the development of nano Ag/TiO2 coating bracket and its antibacterial property and biocompatibility in orthodontic treatment. Pak J Pharm Sci. 2015;28(2 Suppl):807-10. [PubMed ID: 25796158].
-
4.
Salehi P, Babanouri N, Roein-Peikar M, Zare F. Long-term antimicrobial assessment of orthodontic brackets coated with nitrogen-doped titanium dioxide against Streptococcus mutans. Prog Orthod. 2018;19(1):35. [PubMed ID: 30221309]. [PubMed Central ID: PMC6139290]. https://doi.org/10.1186/s40510-018-0236-y.
-
5.
Heymann HO, Swift EJ, Ritter AV. Tabari K, translator. [Art and Science of operative dentisry]. 2nd ed. Tehran, Iran: Royan Pazhoh; 2013. p. 51-98. Persian.
-
6.
Mizrahi E. Enamel demineralization following orthodontic treatment. Am J Orthod. 1982;82(1):62-7. [PubMed ID: 6984291]. https://doi.org/10.1016/0002-9416(82)90548-6.
-
7.
Mattingly JA, Sauer GJ, Yancey JM, Arnold RR. Enhancement of Streptococcus mutans colonization by direct bonded orthodontic appliances. J Dent Res. 1983;62(12):1209-11. [PubMed ID: 6361082]. https://doi.org/10.1177/00220345830620120601.
-
8.
Garcia-Godoy F, Hicks MJ. Maintaining the integrity of the enamel surface: The role of dental biofilm, saliva and preventive agents in enamel demineralization and remineralization. J Am Dent Assoc. 2008;139 Suppl:25S-34S. [PubMed ID: 18460677]. https://doi.org/10.14219/jada.archive.2008.0352.
-
9.
Simark-Mattsson C, Emilson CG, Hakansson EG, Jacobsson C, Roos K, Holm S. Lactobacillus-mediated interference of mutans streptococci in caries-free vs. caries-active subjects. Eur J Oral Sci. 2007;115(4):308-14. [PubMed ID: 17697171]. https://doi.org/10.1111/j.1600-0722.2007.00458.x.
-
10.
Midander K, Cronholm P, Karlsson HL, Elihn K, Moller L, Leygraf C, et al. Surface characteristics, copper release, and toxicity of nano- and micrometer-sized copper and copper(II) oxide particles: A cross-disciplinary study. Small. 2009;5(3):389-99. [PubMed ID: 19148889]. https://doi.org/10.1002/smll.200801220.
-
11.
Zhang HX, Siegert U, Liu R, Cai WB. Facile fabrication of ultrafine copper nanoparticles in organic solvent. Nanoscale Res Lett. 2009;4(7):705-8. [PubMed ID: 20596465]. [PubMed Central ID: PMC2893818]. https://doi.org/10.1007/s11671-009-9301-2.
-
12.
Yin IX, Zhang J, Zhao IS, Mei ML, Li Q, Chu CH. The Antibacterial Mechanism of Silver Nanoparticles and Its Application in Dentistry. Int J Nanomedicine. 2020;15:2555-62. [PubMed ID: 32368040]. [PubMed Central ID: PMC7174845]. https://doi.org/10.2147/IJN.S246764.
-
13.
Visai L, De Nardo L, Punta C, Melone L, Cigada A, Imbriani M, et al. Titanium oxide antibacterial surfaces in biomedical devices. Int J Artif Organs. 2011;34(9):929-46. [PubMed ID: 22094576]. https://doi.org/10.5301/ijao.5000050.
-
14.
Hashimoto K, Irie H, Fujishima A. TiO2Photocatalysis: A Historical Overview and Future Prospects. Jpn J Appl Phys. 2005;44(12):8269-85. https://doi.org/10.1143/jjap.44.8269.
-
15.
Mocanu A, Furtos G, Rapuntean S, Horovitz O, Flore C, Garbo C, et al. Synthesis; characterization and antimicrobial effects of composites based on multi-substituted hydroxyapatite and silver nanoparticles. Appl Surf Sci. 2014;298:225-35. https://doi.org/10.1016/j.apsusc.2014.01.166.
-
16.
Ferraz MP, Monteiro FJ, Manuel CM. Hydroxyapatite nanoparticles: A review of preparation methodologies. J Appl Biomater Biomech. 2004;2(2):74-80. [PubMed ID: 20803440].
-
17.
Ciobanu CS, Iconaru SL, Chifiriuc MC, Costescu A, Le Coustumer P, Predoi D. Synthesis and antimicrobial activity of silver-doped hydroxyapatite nanoparticles. Biomed Res Int. 2013;2013:916218. [PubMed ID: 23509801]. [PubMed Central ID: PMC3591194]. https://doi.org/10.1155/2013/916218.
-
18.
Shrivastava S, Bera T, Roy A, Singh G, Ramachandrarao P, Dash D. Characterization of enhanced antibacterial effects of novel silver nanoparticles. Nanotechnology. 2007;18(22):225103. https://doi.org/10.1088/0957-4484/18/22/225103.
-
19.
Yu B, Leung KM, Guo Q, Lau WM, Yang J. Synthesis of Ag-TiO2 composite nano thin film for antimicrobial application. Nanotechnology. 2011;22(11):115603. [PubMed ID: 21387845]. https://doi.org/10.1088/0957-4484/22/11/115603.
-
20.
Bacela J, Labowska MB, Detyna J, Ziety A, Michalak I. Functional Coatings for Orthodontic Archwires-A Review. Materials (Basel). 2020;13(15). [PubMed ID: 32707959]. [PubMed Central ID: PMC7435379]. https://doi.org/10.3390/ma13153257.
-
21.
Metin-Gursoy G, Taner L, Akca G. Nanosilver coated orthodontic brackets: in vivo antibacterial properties and ion release. Eur J Orthod. 2017;39(1):9-16. [PubMed ID: 26787659]. https://doi.org/10.1093/ejo/cjv097.
-
22.
Cao B, Wang Y, Li N, Liu B, Zhang Y. Preparation of an orthodontic bracket coated with an nitrogen-doped TiO(2-x)N(y) thin film and examination of its antimicrobial performance. Dent Mater J. 2013;32(2):311-6. [PubMed ID: 23538768]. https://doi.org/10.4012/dmj.2012-155.
-
23.
Grosso D. How to exploit the full potential of the dip-coating process to better control film formation. J Mater Chem. 2011;21(43):17033. https://doi.org/10.1039/c1jm12837j.
-
24.
Borges J, Mano JF. Molecular interactions driving the layer-by-layer assembly of multilayers. Chem Rev. 2014;114(18):8883-942. [PubMed ID: 25138984]. https://doi.org/10.1021/cr400531v.
-
25.
Metin-Gursoy G, Taner L, Baris E. Biocompatibility of nanosilver-coated orthodontic brackets: An in vivo study. Prog Orthod. 2016;17(1):39. [PubMed ID: 27800593]. [PubMed Central ID: PMC5136532]. https://doi.org/10.1186/s40510-016-0152-y.
-
26.
Ruparelia JP, Chatterjee AK, Duttagupta SP, Mukherji S. Strain specificity in antimicrobial activity of silver and copper nanoparticles. Acta Biomater. 2008;4(3):707-16. [PubMed ID: 18248860]. https://doi.org/10.1016/j.actbio.2007.11.006.
-
27.
Ramazanzadeh B, Jahanbin A, Yaghoubi M, Shahtahmassbi N, Ghazvini K, Shakeri M, et al. Comparison of Antibacterial Effects of ZnO and CuO Nanoparticles Coated Brackets against Streptococcus Mutans. J Dent (Shiraz). 2015;16(3):200-5. [PubMed ID: 26331150]. [PubMed Central ID: PMC4554313].
-
28.
Rai M, Yadav A, Gade A. Silver nanoparticles as a new generation of antimicrobials. Biotechnol Adv. 2009;27(1):76-83. [PubMed ID: 18854209]. https://doi.org/10.1016/j.biotechadv.2008.09.002.
-
29.
Meyer-Kobbe V, Doll K, Stiesch M, Schwestka-Polly R, Demling A. Comparison of intraoral biofilm reduction on silver-coated and silver ion-implanted stainless steel bracket material : Biofilm reduction on silver ion-implanted bracket material. J Orofac Orthop. 2019;80(1):32-43. [PubMed ID: 30535568]. [PubMed Central ID: PMC6334737]. https://doi.org/10.1007/s00056-018-00165-3.
-
30.
Ferrando-Magraner E, Bellot-Arcis C, Paredes-Gallardo V, Almerich-Silla JM, Garcia-Sanz V, Fernandez-Alonso M, et al. Antibacterial Properties of Nanoparticles in Dental Restorative Materials. A Systematic Review and Meta-Analysis. Medicina (Kaunas). 2020;56(2). [PubMed ID: 32013103]. [PubMed Central ID: PMC7073742]. https://doi.org/10.3390/medicina56020055.
-
31.
Carrouel F, Viennot S, Ottolenghi L, Gaillard C, Bourgeois D. Nanoparticles as Anti-Microbial, Anti-Inflammatory, and Remineralizing Agents in Oral Care Cosmetics: A Review of the Current Situation. Nanomaterials (Basel). 2020;10(1). [PubMed ID: 31941021]. [PubMed Central ID: PMC7022934]. https://doi.org/10.3390/nano10010140.
-
32.
Kotta M, Gorantla S, Muddada V, Lenka RR, Karri T, Kumar S, et al. Antibacterial activity and debonding force of different lingual retainers bonded with conventional composite and nanoparticle containing composite: An in vitro study. J World Fed Orthod. 2020;9(2):80-5. [PubMed ID: 32672659]. https://doi.org/10.1016/j.ejwf.2020.03.001.