Abstract
Background:
Statins are widely used to control the level of plasma cholesterol. There is growing evidence that statins reduce the prevalence of Alzheimer disease (AD) and dementia.Objectives:
The present study evaluated the effect of lovastatin treatment on neuronal responses of pyramidal cells of the CA1 region of the hippocampus in a rat model of AD.Methods:
Rats randomly separated into 4 groups (n = 10): the lesion group of nucleus basalis magnocellularis (NBM) that received destruction by an electrical method (0.5 mA, 3 seconds) and lovastatin groups [lesion + lovastatin (10, 20, and 30 mg/kg)]. An in vivo single-unit recording method was used in rats anesthetized with urethane. After 15 minutes of baseline recording from pyramidal neurons in the CA1 region of the hippocampus, intraperitoneal injections of lovastatin and dimethyl sulfoxide (DMSO) were administered, and neuronal response to administration of lovastatin continued to be recorded for 105 minutes.Results:
Acute injection of 30-mg/kg lovastatin excited 11 out of 17 neurons and inhibited 2 neurons. On the other hand, intraperitoneal injection of lovastatin in the highest dose resulted in increased spontaneous activity in the CA1 region of the hippocampus.Conclusions:
The acute use of lovastatin increases the neuronal frequency in the pyramidal neurons of the CA1 region of the hippocampus.Keywords
Alzheimer Disease Lovastatin Extracellular Single-Unit Recording Rat
1. Background
Memory is the ability to remember past events by conscious or unconscious, as well as the mechanism for encoding, storing, and recalling information (1). Memory plays a decisive role in human performance, and without it, it is impossible to perform the simplest task (2). Over the past decades, the subject of memory and cognitive impairment has attracted many researchers. Pharmacological studies on memory are conducted in the hope that behavioral findings, along with the mechanism of action of drugs, will examine and clarify the basis of neurobiological memory and learning (3). Alzheimer disease (AD), the most common cause of forgetting, is a progressive neurodegenerative disease associated with loss of neurons in various brain areas and memory impairment (4). Neuropathologically, the brain regions associated with cognition, learning, and memory (especially the hippocampus and neocortex) are more affected by AD (5). One of the neuropathological characteristics of AD is the destruction of large cholinergic neurons of the nucleus basalis of Meynert and balanced to nucleus basalis magnocellularis (NBM) of rodents, which is responsible for many of the cognitive deficiencies and memory of the disease (6). About 90% of NBM cholinergic nuclei have their fibers emitted into all cortical regions and amygdala nuclei (7). In AD, 50% to 88% of these cholinergic neurons disappear (8, 9). Studies have shown that cholinergic brain markers (including the release of acetylcholine and choline acetyltransferase activity) reduce and learning and memory are damaged in NBM-lesion rats (10). Statins, the competitive inhibitors of 3-hydroxy-3-methylglutaryl-COA (HMG-COA) reductase, are commonly used to treat hypercholesterolemia. Evolving evidence suggests that this group of drugs has beneficial effects on neurological disorders (such as AD), traumatic brain injury, and stroke (11). In vivo experiments have shown that statin therapy increases neurogenicity and synaptogenesis after brain damage without altering serum cholesterol levels. These findings suggest that statins promote neuronal protection by releasing neurotrophic factors (12). The treatment with statins leads to an increase in soluble amyloid precursor protein (s-APPα) production and a decrease in beta-amyloid production (13). Simvastatin and atorvastatin lead to neurogenesis and inhibit neuronal death, and lovastatin results in decreased inflammation (14). In addition to lovastatin, simvastatin, and atorvastatin repair the cognitive impairments caused by traumatic brain injury (15).
There is a fairly large gap between the properties of the neuron membrane and the physiological and behavioral events. To this end, we must be able to understand the activity of neurons. Extra single-unit recording techniques provided precious data about the characteristics of central nervous system structures. In neuroscience, the single-unit recording provides a method for measuring electrophysiological responses to individual neurons using a microelectrode system (16). Most research on AD has examined memory defects, and spontaneous activity of neurons in the brain has been poorly studied. Therefore, in the present research, the effect of lovastatin on the spontaneous activity of pyramidal neurons in the CA1 region of the hippocampus was studied in a rat model of AD.
2. Objectives
This study aimed to evaluate the effect of lovastatin on the electrical activity of the CA1 region in a rat model of AD.
3. Methods
Forty adult male Wistar rats weighing approximately 200 ± 20 g were purchased from Ahvaz Jondishapur University of Medical Sciences. The animals were kept in a room at a constant temperature of 24 ± 2°C and a 12:12 hour light-dark cycle and provided with water and food ad libitum. The charter and ethical guidelines were followed in conducting this research (EE/97.24.3.17933/scu.ac.ir). Lovastatin (Sigma-Aldridge, USA), dissolved in 5% dimethyl sulfoxide (DMSO) as a solvent, was studied in this research.
3.1. Alzheimer Model Induction
Rats were anesthetized with ketamine (78 mg/kg intraperitoneal; Alphasan, Holland) and xylazine (3 mg/kg intraperitoneal; Alphasan, Holland). Afterward, the NBM of the rat was ruined by surgical procedures (AP = -1.3 mm, ML = ± 2.8 mm, DV = -7.6 mm) (7). The NBM was destroyed with an electrical current for 3 seconds at 0.5 mA (17). A histology confirmation of NBM destruction was prepared (Figure 1). Animals were randomly divided into 4 groups as follows: (1) the lesion group in which the NBM of animals was destroyed bilaterally with electrical lesion + DMSO 5%; (2) the lesion group + 10-mg/kg lovastatin (L10); (3) the lesion group + 20-mg/kg lovastatin (L20); (4) and the lesion group + 30-mg/kg lovastatin (L30).
A, Schematic position of the nucleus basalis magnocellularis region in the Paxinus atlas; B, Sample confirmation of the location of damage to the nucleus basalis magnocellularis.
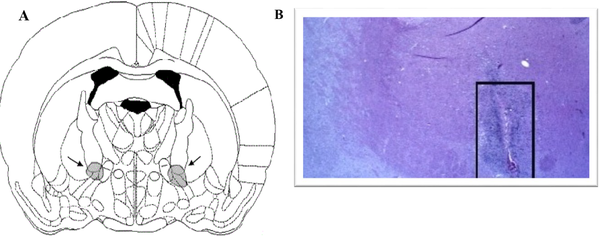
3.2. Animal Preparation and Stereotactic Surgery
After the destruction of NBM and the recovery period, animals were prepared for electrophysiological tests. They were anesthetized by administration of 1.5 g/kg intraperitoneal urethane (Sigma-Aldrich, Germany), which was performed every 1 hour as needed to keep a deep and stable level of anesthesia (18). To create a stable airway and reduce the respiratory noise, animals were placed in surgical tracheostomy. For this purpose, the hair in front of the neck area was removed. Then, soft tissues of the neck region were pushed aside to reach the trachea. A transverse incision was made in the trachea, and a 1.5-mm diameter polyethylene tube was inserted into it. After that, the tracheostomy rat was slowly placed in a stereotactic device (Stoelting, USA). The rat’s skull was cleaned, and the Bergema was identified and used as a surgical reference point. A hole of 2 mm was created according to the atlas of Paxinus (-3.8 mm AP, ± 2.2 mm ML, -2.4 mm DV) at the top of the CA1 region. The body temperature was maintained at 36 - 37°C throughout the experiments using a heating pad (19).
3.3. Extracellular Single-Unit Recording
In this research, using the single-unit recording method, the effect of lovastatin and its solvent on the electrical activity of pyramidal hippocampal neurons was evaluated. To do this, a tungsten electrode, a tungsten-coated parylene microelectrode with a diameter of 127 μm, and 5-MΩ slowly were inserted into the brain to reach the top of the hippocampus. Then, the electrode was gently pulled down with the aid of a manual microdrive until it reached the pyramidal cell layer of the CA1 region of the hippocampus, while the electrical potentials that reached the tip of the electrode were monitored. The electric signals generated by the action potentials of pyramidal neurons (microvolt) from the electrode tip were amplified 10000 times after reaching the amplifier. Then, after passing a ban-pass filter, a signal was sent to the data acquisition device and eventually displayed on a computer. Electrical data were recorded using Spike software (Spike, Science Beam, Tehran, Iran). To find the pyramidal neuron with spontaneous activity, the electrode was dropped into the hippocampus, the tip of which was sometimes close to a neuron that could remove the potentials from it and transfer it to the amplifier. At that time, the peak of the signals generated by the neurons was sufficiently large to easily identify the activity of the surrounding peripheral neurons. Only neurons with a signal of less than 8 times per second were accepted for review in this study (20). The total recording time for this research was 7200 seconds with a 1000-ms bin size, which is constantly stored on a hard disk. The average frequency of each unit was calculated by a computer. Peri-stimulus time histograms (PSTHs) from 15 minutes before until 105 minutes after injection of the drug or solvent were determined. The findings were analyzed using offline software for windows. Increasing or decreasing the frequency of neurons more than twice the standard deviation of the baseline activity of neurons for the 3 following points was selected as excitatory and inhibitory neuronal activity.
3.4. Histological Verification
After the electrophysiological record was completed, the animal’s brain was removed and placed in formalin 10%. After the preparation of the block, 20-μm sections were prepared from a nearby electrode. Slices were stained with hematoxylin and eosin. Then, using the microscope, the location of the electrode was determined in the CA1 region of the hippocampus (Figure 2).
A, Schematic position of the CA1 region of the hippocampus in the Paxinus atlas; B, Sample from the entry point of the electrode to the CA1 region of the hippocampus.
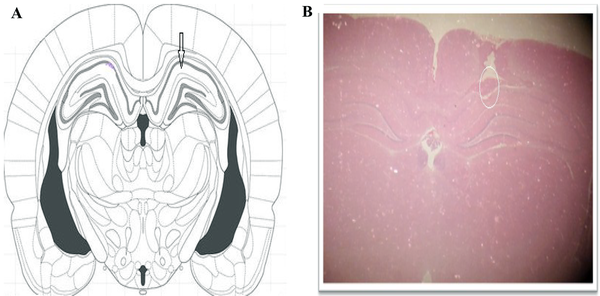
3.5. Statistical Analyze
Data were analyzed using SPSS version 20 (SPSS Inc, Chicago, Ill, USA). To perform statistical comparisons in the electrophysiology recording, the paired sample t-test was used to evaluate the effect of the drug and vehicle on the spontaneous activity of each neuron in each group in a 2-hour record. Also, 1-way analysis of variance (ANOVA) and Tukey’s post hoc test were used to evaluate the effect of the drug on different groups. In addition, GraphPad Prism version 6.07 was used to plot the number of excitatory, inhibitory, and ineffective neurons in the electrophysiology section. Data were expressed as mean ± SD. P values less than 0.05 were considered statistically significant.
4. Results
To study the effect of DMSO on the firing activity of pyramidal neurons, 0.2 mL of DMSO was injected intraperitoneal after baseline, and then the record continued for 105 minutes. The results of the t-test for spontaneous activity of neurons in the lesion group showed that the injection of DMSO had no significant improvement in the firing neurons after administration compared to baseline recording (P > 0.05). In the 10-mg/kg lovastatin group, a paired t-test analysis showed no significant increase in the frequency of neuronal activity after drug injection compared to the baseline recording (P > 0.05; Figure 3A). Fourteen neurons from 10 rats were registered in this group, showing an excitatory effect on 3 neurons, an inhibitory effect on 3 neurons, and no effect on 8 neurons (Figure 3B). In the 20-mg/kg lovastatin group, a paired t-test analysis showed a significant increase in the frequency of neuron activity after drug injection compared to the baseline recording (P = 0.046; Figure 4A). Fifteen neurons from 10 rats were recorded in this group, showing an excitatory effect on 5 neurons, an inhibitory effect on 2 neurons, and no effect on 8 neurons (Figure 4B). Also, administration of 20-mg/kg lovastatin led to an enhancement of 48% to 97% in 3 neurons, an increase in activity of 130% to 180% in 2 neurons, and an inhibitory effect of 53% to 55% in 2 neurons. The statistical analysis results in the 30-mg/kg lovastatin group showed a significant increase in neuron frequency compared to baseline recording (P < 0.001; Figure 5A and B). In this group, 17 neurons from 10 rats were recorded, showing a stimulatory effect on 11 neurons, an inhibitory effect on 2 neurons, and no effect on 4 neurons (Figure 5C). Injection of 30-mg/kg lovastatin led to an enhancement in the activity of 85% to 170% in 8 neurons, 230% in 1 neuron, and 312% to 370% in 2 neurons. Also, the results of 1-way ANOVA of the mean excitatory activity showed a significant difference between the 30-mg/kg lovastatin and DMSO groups (P = 0.032). However, no significant difference was observed between the 20-mg/kg lovastatin and 10-mg/kg lovastatin groups with the DMSO group (P > 0.05; Figure 6).
A, Diagram of pyramidal neuron in the CA1 region of the hippocampus with different responses to 10-mg/kg lovastatin injection. The firing frequency did not change relative to the baseline firing (P > 0.05); B, Scatterplot illustrating pyramidal neurons with different responses to intraperitoneal injection of dimethyl sulfoxide and 10-mg/kg lovastatin.
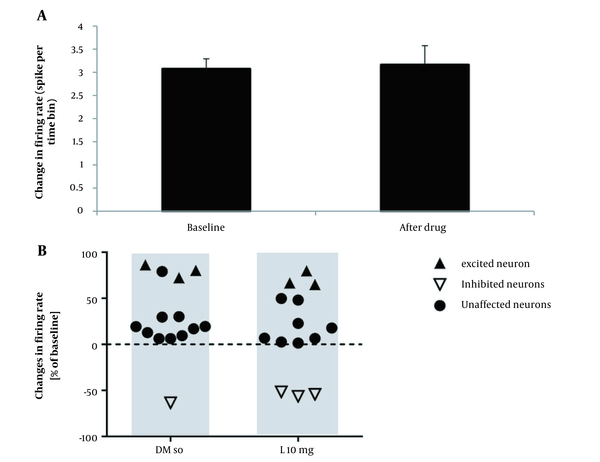
A, Diagram of pyramidal neuron in the CA1 region of the hippocampus with different responses to 20-mg/kg lovastatin injection. The firing frequency significantly changed relative to the baseline firing (P < 0.05); B, Scatterplot illustrating pyramidal neurons with different responses to intraperitoneal injection of dimethyl sulfoxide and 20-mg/kg lovastatin.
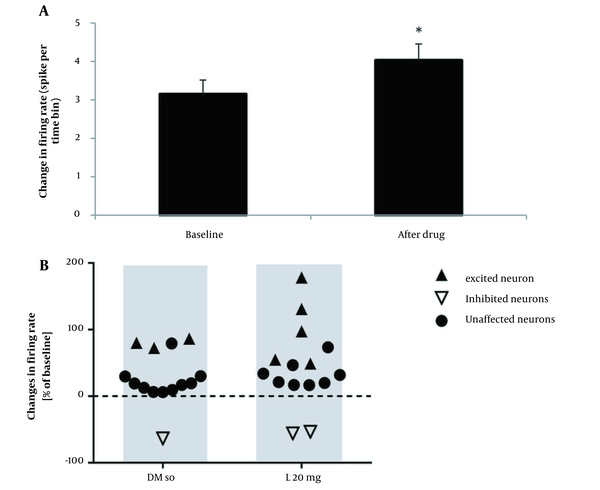
A, Diagram of pyramidal neuron in the CA1 region of the hippocampus with different responses to 30-mg/kg lovastatin injection. The firing frequency significantly changed relative to the baseline firing (P < 0.001); B, Firing diagram of pyramidal neurons in the CA1 region of the hippocampus before and after 30-mg/kg lovastatin injection. Histogram representing spike count per bin of 1 minute over the entire recording; C, Scatterplot illustrating pyramidal neurons with different responses to intraperitoneal injection of dimethyl sulfoxide and 30-mg/kg lovastatin.
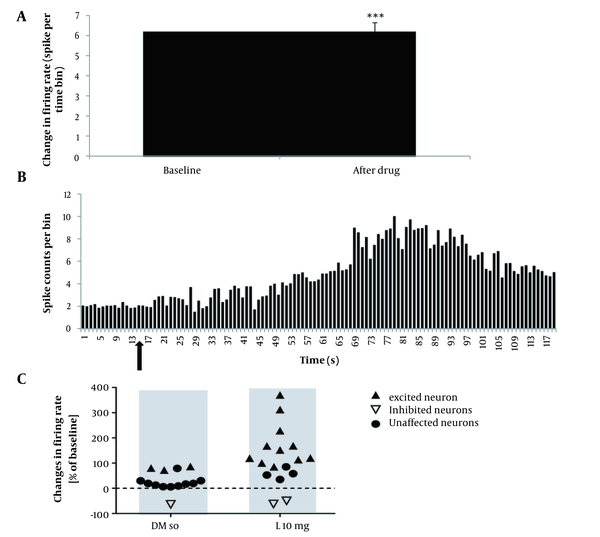
Comparison of the mean excitatory activity (mean ± SD) in the dimethyl sulfoxide and lovastatin (10, 20, and 30 mg/kg) groups (*P < 0.05 in comparison with the dimethyl sulfoxide group).
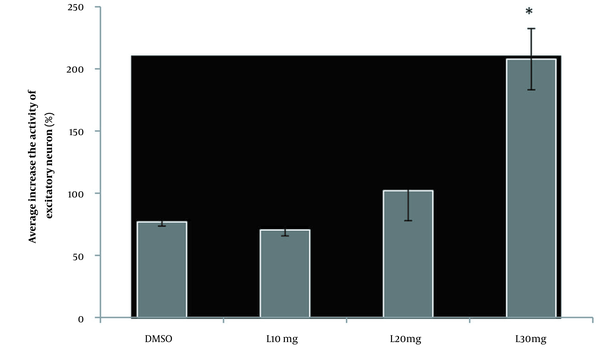
5. Discussion
In this study, the effects of different doses of lovastatin were examined on the activity of pyramidal neurons in a rat model of AD. It was found that 20- and 30-mg/kg lovastatin had positive effects and increased spontaneous activity of pyramidal neurons of the hippocampal CA1 region. Destruction of the cholinergic system of NBM in rodents and primates decreases the cholinergic function of the cortex and leads to memory deficits and learning (21). The NBM is involved in the storage of working memory, as well as the storage of reference memory information (22). Studies have shown that impaired performance from the cholinergic system derived from the basal forebrain that irritates several cortical regions and the hippocampus, associated with memory deficit and neurodegeneration observed in several types of dementia, including AD (23). Studies have shown that lipophilic statins (such as lovastatin) reduce the activity of the acetylcholinesterase enzyme in the frontal cortex in rats, which leads to an increase in acetylcholine levels in the synaptic cleft (24). The release of acetylcholine and activation of muscarinic receptors leads to increased learning and memory in an extensive range of behavioral tests (25). The activation of muscarinic receptors leads to the depolarization and enhanced excitation of the pyramidal neurons of the CA1 region by activating cationic conduction and inhibiting potassium activity (26). Studies have shown that acetylcholine leads to inhibition of a calcium-activated potassium current, inhibition of leak K+ conductance, and enhanced conductance in the high-voltage-activated Ca2+ channel (27). It has also been reported that lovastatin results in upregulation of ɑ7-nAchRs, which, in an independent cholesterol-based mechanism, leads to an increase in ɑ-APPs production during APP processing, resulting in a decrease in Aβ levels (24). Chen et al. reported that treatment of hippocampal slices of mice with simvastatin led to an increased presynaptic glutamate release and an increase in the amplitude of N-methyl-D-aspartate (NMDA) and receptor-dependent long-term potentiation (LTP) amplitude. Isoprenoid supplementation of farnesyl pyrophosphate resulted in the elimination of the effect of simvastatin on its release of glutamate and LTP enhancement. The results of this study suggested that by reducing farnesyl pyrophosphate, simvastatin resulted in increased expression of α7-nAchR and phosphorylation of protein kinase B and ERK2 dependent on the α7-nAchR receptor, which led to an increase in LTP in the hippocampal slices (28).
Mans et al. (29) showed that the ability of simvastatin to inhibit the production of isoprenoids resulted in an increase in the induction of LTP. In this study, the use of farnesyl reduced the amplitude of induced LTP by simvastatin in the hippocampal slices of C57BL/6 mice. On the other hand, the effect of simvastatin on LTP can be imitated by the inhibition of farnesyltransferase. It has also been shown that the high expression of Ras decreases the tyrosine phosphorylation NR2A and LTP. These findings suggest that inhibition of Ras can enhance the NMDA receptor-dependent synaptic plasticity. Mans et al. also reported that LTP increased after treatment with a farnesyltransferase inhibitor, which led to the inhibition of farnesylation of Ras. It is worth mentioning that the brain of AD patients produces a large amount of farnesyl pyrophosphate and granyl-granyl pyrophosphate (29). However, Maggo and Ashton indicated that simvastatin and atorvastatin resulted in a significant decrease in LTP in the hippocampal slices of guinea pigs. In contrast, in our study, the injection of lovastatin resulted in an increase in the spontaneous activity of pyramidal neurons of the CA1 region of the hippocampus. The reason for the difference in the results could be due to the different types of animal models (guinea pigs vs mice and rats) and that the LTP induction protocol is an important factor in the observed difference. The intensity of stimulation is a key factor in the induction of LTP (30). Thus, according to the findings of this research and other related studies, lovastatin may be associated with mechanisms such as reducing isoprenoid production, increased expression of acetylcholine receptors, activation of protein kinase B, and enhancement of NMDA receptor subunits in increased spontaneous activity of pyramidal neurons in the CA1 region of the hippocampus.
Acknowledgements
References
-
1.
Brem AK, Ran K, Pascual-Leone A. Learning and memory. Handb Clin Neurol. 2013;116:693-737. [PubMed ID: 24112934]. [PubMed Central ID: PMC4248571]. https://doi.org/10.1016/B978-0-444-53497-2.00055-3.
-
2.
Tabatabaei M, Shakeri M, Ebadi H. [Evaluation of extract of Ginkgo Biloba (EGB) and its effect on memory of healthy volunteer students in Mashad University]. Med J Mashhad Univ Med Sci. 2005;47(86):367-2. Persian.
-
3.
Narwal S, Saini DR, Kumari K, Narwal S, Singh G, Negi RS, et al. Behavior & Pharmacological Animal Models for the Evaluation of Learning & Memory Condition. Indo Glob J Pharm Sci. 2012;2(2):121-9.
-
4.
Dua JS, Prasad DN, Tripathi AC, Gupta R. Role of traditional medicine in neuropsychopharmacology. Asian J Pharm Clin Res. 2009;2(2):72-6.
-
5.
Akaike A, Takada-Takatori Y, Kume T, Izumi Y. Mechanisms of neuroprotective effects of nicotine and acetylcholinesterase inhibitors: role of alpha4 and alpha7 receptors in neuroprotection. J Mol Neurosci. 2010;40(1-2):211-6. [PubMed ID: 19714494]. https://doi.org/10.1007/s12031-009-9236-1.
-
6.
Szigeti C, Bencsik N, Simonka AJ, Legradi A, Kasa P, Gulya K. Long-term effects of selective immunolesions of cholinergic neurons of the nucleus basalis magnocellularis on the ascending cholinergic pathways in the rat: a model for Alzheimer's disease. Brain Res Bull. 2013;94:9-16. [PubMed ID: 23357177]. https://doi.org/10.1016/j.brainresbull.2013.01.007.
-
7.
Rabiei Z, Rafieian-Kopaei M, Heidarian E, Saghaei E, Mokhtari S. Effects of Zizyphus jujube extract on memory and learning impairment induced by bilateral electric lesions of the nucleus Basalis of Meynert in rat. Neurochem Res. 2014;39(2):353-60. [PubMed ID: 24379110]. https://doi.org/10.1007/s11064-013-1232-8.
-
8.
Nyakas C, Granic I, Halmy LG, Banerjee P, Luiten PG. The basal forebrain cholinergic system in aging and dementia. Rescuing cholinergic neurons from neurotoxic amyloid-beta42 with memantine. Behav Brain Res. 2011;221(2):594-603. [PubMed ID: 20553766]. https://doi.org/10.1016/j.bbr.2010.05.033.
-
9.
Cuello AC, Bruno MA, Allard S, Leon W, Iulita MF. Cholinergic involvement in Alzheimer's disease. A link with NGF maturation and degradation. J Mol Neurosci. 2010;40(1-2):230-5. [PubMed ID: 19680822]. https://doi.org/10.1007/s12031-009-9238-z.
-
10.
Francis PT, Parsons CG, Jones RW. Rationale for combining glutamatergic and cholinergic approaches in the symptomatic treatment of Alzheimer's disease. Expert Rev Neurother. 2012;12(11):1351-65. [PubMed ID: 23234396]. https://doi.org/10.1586/ern.12.124.
-
11.
Peng W, Yang J, Yang B, Wang L, Xiong XG, Liang Q. Impact of statins on cognitive deficits in adult male rodents after traumatic brain injury: a systematic review. Biomed Res Int. 2014;2014:261409. [PubMed ID: 25157352]. [PubMed Central ID: PMC4135130]. https://doi.org/10.1155/2014/261409.
-
12.
van der Most PJ, Dolga AM, Nijholt IM, Luiten PG, Eisel UL. Statins: mechanisms of neuroprotection. Prog Neurobiol. 2009;88(1):64-75. [PubMed ID: 19428962]. https://doi.org/10.1016/j.pneurobio.2009.02.002.
-
13.
Li R, Xu DE, Ma T. Lovastatin suppresses the aberrant tau phosphorylation from FTDP-17 mutation and okadaic acid-induction in rat primary neurons. Neuroscience. 2015;294:14-20. [PubMed ID: 25770969]. https://doi.org/10.1016/j.neuroscience.2015.03.005.
-
14.
Chen SF, Hung TH, Chen CC, Lin KH, Huang YN, Tsai HC, et al. Lovastatin improves histological and functional outcomes and reduces inflammation after experimental traumatic brain injury. Life Sci. 2007;81(4):288-98. [PubMed ID: 17612572]. https://doi.org/10.1016/j.lfs.2007.05.023.
-
15.
Chauhan NB, Gatto R. Restoration of cognitive deficits after statin feeding in TBI. Restor Neurol Neurosci. 2011;29(1):23-34. [PubMed ID: 21335666]. https://doi.org/10.3233/RNN-2011-0573.
-
16.
Henze DA, Borhegyi Z, Csicsvari J, Mamiya A, Harris KD, Buzsaki G. Intracellular features predicted by extracellular recordings in the hippocampus in vivo. J Neurophysiol. 2000;84(1):390-400. [PubMed ID: 10899213]. https://doi.org/10.1152/jn.2000.84.1.390.
-
17.
Moazedi AA, Moosavi M, Chinipardaz R. [The effect of estrogen on passive avoidance memory in an experimental model of Alzheimer disease in male rats]. Physiol Pharmacol. 2011;14(4):416-25. Persian.
-
18.
Riahi E, Arezoomandan R, Fatahi Z, Haghparast A. The electrical activity of hippocampal pyramidal neuron is subjected to descending control by the brain orexin/hypocretin system. Neurobiol Learn Mem. 2015;119:93-101. [PubMed ID: 25680816]. https://doi.org/10.1016/j.nlm.2015.02.001.
-
19.
Moldestad O, Karlsen P, Molden S, Storm JF. Tracheotomy improves experiment success rate in mice during urethane anesthesia and stereotaxic surgery. J Neurosci Methods. 2009;176(2):57-62. [PubMed ID: 18778735]. https://doi.org/10.1016/j.jneumeth.2008.08.015.
-
20.
Green JT, Arenos JD. Hippocampal and cerebellar single-unit activity during delay and trace eyeblink conditioning in the rat. Neurobiol Learn Mem. 2007;87(2):269-84. [PubMed ID: 17046292]. [PubMed Central ID: PMC1907365]. https://doi.org/10.1016/j.nlm.2006.08.014.
-
21.
Gratwicke J, Kahan J, Zrinzo L, Hariz M, Limousin P, Foltynie T, et al. The nucleus basalis of Meynert: a new target for deep brain stimulation in dementia? Neurosci Biobehav Rev. 2013;37(10 Pt 2):2676-88. [PubMed ID: 24035740]. https://doi.org/10.1016/j.neubiorev.2013.09.003.
-
22.
Lee JE, Jeong DU, Lee J, Chang WS, Chang JW. The effect of nucleus basalis magnocellularis deep brain stimulation on memory function in a rat model of dementia. BMC Neurol. 2016;16:6. [PubMed ID: 26757896]. [PubMed Central ID: PMC4711102]. https://doi.org/10.1186/s12883-016-0529-z.
-
23.
Contestabile A, Ciani E, Contestabile A. The place of choline acetyltransferase activity measurement in the "cholinergic hypothesis" of neurodegenerative diseases. Neurochem Res. 2008;33(2):318-27. [PubMed ID: 17940885]. https://doi.org/10.1007/s11064-007-9497-4.
-
24.
Dolga AM, Nijholt IM, Ostroveanu A, Ten Bosch Q, Luiten PG, Eisel UL. Lovastatin induces neuroprotection through tumor necrosis factor receptor 2 signaling pathways. J Alzheimers Dis. 2008;13(2):111-22. [PubMed ID: 18376053]. https://doi.org/10.3233/jad-2008-13201.
-
25.
Haam J, Yakel JL. Cholinergic modulation of the hippocampal region and memory function. J Neurochem. 2017;142 Suppl 2:111-21. [PubMed ID: 28791706]. [PubMed Central ID: PMC5645066]. https://doi.org/10.1111/jnc.14052.
-
26.
Yamada-Hanff J, Bean BP. Persistent sodium current drives conditional pacemaking in CA1 pyramidal neurons under muscarinic stimulation. J Neurosci. 2013;33(38):15011-21. [PubMed ID: 24048831]. [PubMed Central ID: PMC3776055]. https://doi.org/10.1523/JNEUROSCI.0577-13.2013.
-
27.
Fraser DD, MacVicar BA. Cholinergic-dependent plateau potential in hippocampal CA1 pyramidal neurons. J Neurosci. 1996;16(13):4113-28. [PubMed ID: 8753873]. [PubMed Central ID: PMC6578995].
-
28.
Chen T, Wang C, Sha S, Zhou L, Chen L, Chen L. Simvastatin Enhances Spatial Memory and Long-Term Potentiation in Hippocampal CA1 via Upregulation of alpha7 Nicotinic Acetylcholine Receptor. Mol Neurobiol. 2016;53(6):4060-72. [PubMed ID: 26198568]. https://doi.org/10.1007/s12035-015-9344-6.
-
29.
Mans RA, McMahon LL, Li L. Simvastatin-mediated enhancement of long-term potentiation is driven by farnesyl-pyrophosphate depletion and inhibition of farnesylation. Neuroscience. 2012;202:1-9. [PubMed ID: 22192838]. [PubMed Central ID: PMC3626073]. https://doi.org/10.1016/j.neuroscience.2011.12.007.
-
30.
Maggo S, Ashton JC. Effects of HMG-CoA reductase inhibitors on learning and memory in the guinea pig. Eur J Pharmacol. 2014;723:294-304. [PubMed ID: 24296319]. https://doi.org/10.1016/j.ejphar.2013.11.018.