Abstract
Background:
Detergents and difficulties of their presence in the water and wastewater cause various problems such as foam production, anomalies in the growth of algae, and accumulation and dispersion in aqueous environments.Objectives:
The aim of this study was to examine and compare the moving bed biofilm reactor with another reactor without media (carries) for anionic surfactants removal.Materials and Methods:
In this study, the media was from the 2H Company with specific surface of 535 m2/m3 and the experiment reactor was made of a 4-mm plexiglass. The experiment was conducted by using two reactor, as one of them was without media, with exactly similar conditions to compare their operations. The standard method (MBAS) was used to measure the anionic surfactant. The concentrations of the examined anionic surfactant were 50, 100, 200, 300, and 400 mg/L in hydraulic retention times (HRTs) of 72, 24 and 8 hours.Results:
The removal percentage for both reactors at 50 mg/L concentration of pollutant was same, but after gradually increasing the pollutant concentration and decreasing the HRT, the media-containing reactor had significant efficiency in comparison with the other one. The optimum condition in this experiment was obtained at HRT of about 24 hours and 200 mg/L pollutant concentration with 99.2% removal.Conclusions:
Regarding the anionic surfactant standard in Iran which is 1.5 mg/L for surface water discharge, using this process is suitable for treating municipal wastewater and industrial wastewater, containing 100-200 mg/L pollutant. However, for the industries that produce detergents, discharging more than 200 mg/L surfactants to the wastewater, using supplementary treatments for achieving the discharge standard are required.Keywords
1. Background
Of the commonest pollutants of wastewater and water are detergents (1). The main detergents composition is surfactant, originated form the words surface, active and agent. Surfactants are in the organic group (2). They promote cleaning power, foaming and so on (3). Reducing the water surface tension is the basic role of surfactants in detergents (2). Surfactants are typical ingredients of hygienic products and shampoos and are used for different industrial purposes (4). Surfactants are categorized in four groups: anionic, cationic, nonionic, and amphoteric (5). Among these groups, anionic and cationic surfactants are more produced because of their vast usage and popularity, among which, anionic ones are the commonest (6). Linear alkylbenzene sulfonate (LAS) is one of the synthetic anionic surfactants which has gained the alkyl chain from C10-C13. The 12-C type of LAS is called sodium dodecylbenzene sulfonate (SDBS) with a structure similar to LAS. Due to lack of benzene in the SDBS composition and its high level of cleaning power, it is an appropriate substitution for LAS (2). Approximately, 13 million tons of surfactants are produced yearly, among which anionic surfactants are prominent (3). The presence of surfactants in water leads to formation of stable and hardly degradable emulsions and causes anomalies in growth of algae which remove the surfactants from wastewater before they get discharged (1). Different processes have been applied for treatment of surfactants, such as coagulation (3), microwave irradiation, oxidation with ozone, photo catalytic degradation, adsorption of Fenton’s reagent, and electro-chemical process (1). Other biological methods of surfactants degradation are activated sludge and sequencing batch reactors (SBR) (7). Among these methods, moving bed biofilm reactor (MBBR) is another biological process which uses suspended attached growth carriers (media) with specific weights less than water, helping them to be submerged during the process (8). MBBR has diverse advantages such as low sensitivity, easily achieving the mixed-liquor suspended solids (MLSS) of up to 12000 mg/L, requiring a very small area, no sludge recycle, high sludge age, and high expandability (9, 10). According to other researchers who used MBBR for removing detergents (2), the media were natural stones without specific determined surfaces. The detergent removal results revealed that this process can be optimized.
2. Objectives
The aim of this study was to examine and compare the MBBR with another reactor without media (carries) for anionic surfactants removal.
3. Materials and Methods
The synthetic wastewater was daily prepared by solving 100 g/L SDBS (Aldrich) in tap water. The microbial growth nutrient was prepared by mixing the nutrient solution and the synthetic wastewater, keeping the COD/N/P ratio close to 100:5:1 (9). The nutrient ingredients were K2HPO4, KH2PO4, NH4Cl, CaCO3, NaHCO3, respectively 5, 15, 120, 10, and 10 grams, for each liter of tap water (11). Before using the synthetic wastewater for microorganism, its pH was adjusted close to neutral. The structure of the MBBR reactor used in this operation is shown in Figure 1. It consisted of a rectangular cubic 4-mm plexiglass bioreactor (10 × 34 × 10 cm) with a total useful volume of 3 L, as well as a free board, an air injection pump (HAILEA ACO-328 70 L/min), a timer switch, and other equipment. In this experiment, instead of feeding pump, we used gravity feeding tank to manage the droplets injection to the bottom of the bioreactor. The media selected for MBBR was from the 2H Company, with a specific surface area of 535 m2/m3 (Figure 2). The media volume was determined about 30% of the reactor experimental volume. We filled 1/3 of the bioreactor with the sludge from the return sludge of wastewater treatment of a detergent manufacturer company and 2/3 of it with supernatant of a return sludge. We also applied another reactor as a control, exactly under the conditions of the main reactor except for having media, to compare their efficiencies for SDBS removal.
Graphical Scheme of the Reactor
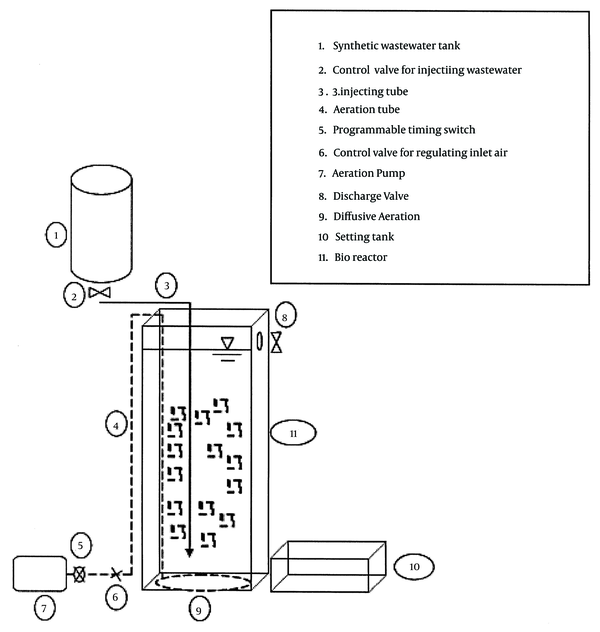
The Media Used in The Experiment With 535 m2/m3 Specific Surface
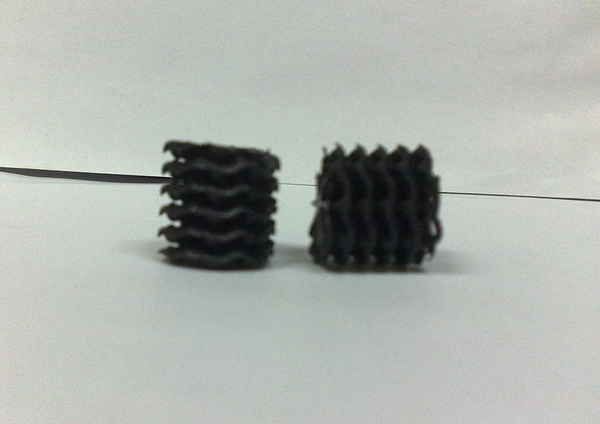
The bioreactors operated at room temperature. To maintain the dissolved oxygen in the wastewater, about 2 mg/L aeration had been doing by 1.6 L/min. At the beginning, the adaptation phase was started for microorganism acclimation. In this condition, the bioreactors were used as batch reactors, which were aerated about two hours and settled down about half an hour. The nutrient and pollutant were regularly injected as SDBS sources. We started feeding the reactors by SDBS from 5 mg/L to 45 mg/L. After bringing the bioreactors to our beginning concentration (50 mg), they were converted to the continuous condition, in which nutrient and SDBS were injected as gravity inlets and permanent aeration was performed. During the operation, foam production was controlled using anti-foam (BASF Company, Germany), of which 2-3 droplets were used for defoaming. The effluent exited from the valve, which was on the boundary line between the free space and the wastewater surface of each bioreactors. The operations were conducted under two situations: concentration variation and constant hydraulic retention time (HRT). We applied SDBS concentrations of 50, 100, 200, 300, and 400 mg/L as pollutants with HRT, respectively, after 8, 24 and 72 hours. To catch the pseudo-steady state, the reactors were kept operating. Constant removal percentages showed that pseudo-steady state was obtained (with variations about 3%) (12). The mineralization degree of SDBS was measured by the chemical oxygen demand (COD) method (13). Obtaining each pseudo-steady state took almost 21-25 days. Table 1 shows the operation conditions.
Stage | Day | Inlet SDBS, mg/L | HRT, h |
---|---|---|---|
A | 0-15 | 50 | 72 |
B | 15-31 | 100 | 72 |
C | 31-54 | 200 | 72 |
D | 54-76 | 200 | 24 |
E | 76-99 | 200 | 8 |
F | 99-115 | 300 | 24 |
G | 115- 132 | 400 | 24 |
SDBS and COD analyses were conducted daily for each reactor. The samples were filtered by 0.42-µm Whatman filters before omitting the particles. The SDBS removal was measured by UV/VIS scanning spectrophotometer (PHILIPS Pu 8750) in 652 nm, using the methylene blue active substances (MBAS) assay, described in standard methods (14). All the materials for the MBAS assay such as sulfuric acid, chloroform, methylene blue and other required materials were procured from the Merck Company. COD was exactly performed according to the standard methods (14). In addition, MLSS was measured to assess the suspended biomass in the reactors (14). The pH values of the influent and the synthetic wastewater were measured by a sensitive electrode (Jenway 3505). The biofilm growth was visualized to show the biofilm morphology, using the scanning electron microscope (SEM) (Hitachi S4160). Before the SEM analysis, the media samples were taken from the bioreactors and dried at room temperature for 16 hours (11).
4. Results
The MBBR performance in biodegradation of SDBS was investigated at inlet SDBS concentrations of 50, 100, 200, 300, and 400 mg/L and HRTs of 72, 24, and 8 hours. Figures 3 and 4 show the results of SDBS biodegradation and removal by MBBR under the explained conditions (Table 1). To assess the mineralization of SDBS, reduction of COD equal to SDBS was investigated periodically. As observed in zones A, B and C of Figure 3, the mean percentage of pseudo-steady state removal of SDBS at 72 hours of HRT were 99.2 %, 99.6%, and 99.4%, for 50, 100, and 200 mg/L SDBS inlet concentrations, respectively, and COD removal percentages equal to SDBS were almost close to these amounts with ± 0.25% variations. Compared with the control reactor, SDBS removal percentages were 99.2%, 98%, and 92%, respectively, for 50, 100 and 200 mg/L SDBS inlet concentrations. These results showed that we achieved the standard SDBS removal for discharging in surface water (1.5 mg/L) by MBBR and defined the inlet concentrations and HRTs. Nevertheless, by using a same reactor without media under similar conditions, the standard of SDBS outlet for discharging into the surface water was not achieved for 100 and 200 mg/L of SDBS concentrations at 72 hours of HRT. It implied that the biomass attached as biofilm on the media surfaces played an important role in biodegradation of SDBS (9). However, when shifting the operation from the 72 hours HRT to 24 hours (by increasing the droplets from 1 mL/88 seconds to 1 mL/29 seconds, considering that each 15-16 droplets is equal to 1 mL) in zone D of Figure 4, degradation levels and COD removal of SDBS suddenly decreased to below 88% and 80%, respectively, for both MBBR and control reactors. The abrupt decreases in SDBS removal efficiency with HRT decrease can be connected to the prohibitory effect of SDBS on metabolism, occurring in microbial nutrients as consequence of an increased volumetric loading rate in the bioreactor (15). However, the removal efficiency in both reactors gradually increased, until they reached to the pseudo-steady state.
Time Course Results of Biodegradation and Sodium Dodecylbenzene Sulfonate Removal in the Moving Bed Biofilm Reactor With Permanent Hydraulic Retention Time in 72 Hours
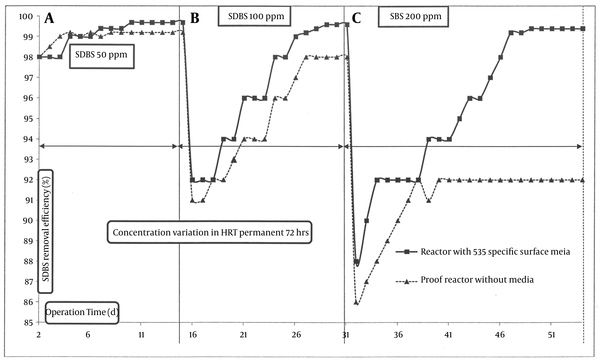
Time Course Results of Biodegradation and Sodium Dodecylbenzene Sulfonate Removal in the Moving Bed Biofilm Reactor With Hydraulic Retention Time Variations and Concentrations of Sodium Dodecylbenzene Sulfonate More Than 200 mg/L With Permanent Hydraulic Retention Time
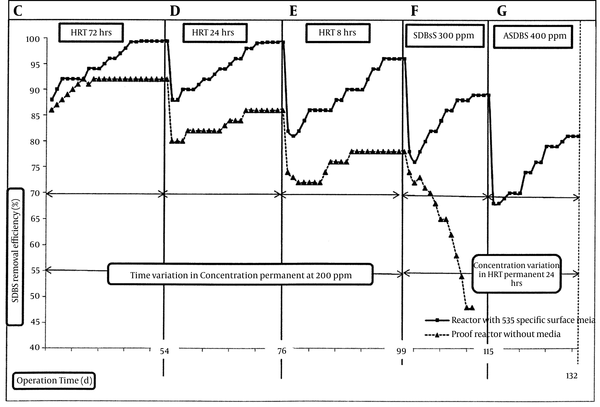
We decreased the HRT again from 24 hours to 8 hours (by increasing the droplets from 1 mL/29 seconds to 1 mL/10 seconds) in Zone E of Figure 4. In this conditions, again we faced decreased SDBS removal efficiency for both MBBR and control reactors to below 82% and 74%, respectively. However, in both reactors, day-by-day increase of the SDBS removal was observed until we reached the pseudo-steady state. We changed the HRT from 72 hours to 24 hours and 8 hours respectively, with 200 mg/L SDBS permanent concentration. Under this situation, the mean percentage of pseudo-steady state removal of SDBS at the HRTs of 24 and 8 hours were 99.2% and 96%, respectively. In comparison with the control reactor, removal percentages of SDBS at the HRTs of 24 and 8 hours were 86% and 78%, respectively. The COD removal percentage varied about ± 0.5% in comparison with the SDBS removal percentage, to find mineralization. In 24 hours of HRT, the standard of discharging into the surface water was obtained by the MBBR reactor; but in the HRT of 8 hours, the standard of discharging into the surface water was not obtained, although the removal percentage was desirable. Under the both conditions of HRT variation (zones D and E), the control reactor did not reach the desirable removal percentages. It attributes again to the biofilm on the media, which significantly enhances the efficiency of removal (16). We continued operating both reactors to obtain the efficient SDBS removal capacity. Since we obtained the discharge standard in the HRT of 24 hours for 200 mg/L SDBS, the reactors were operated in the same HRT with concentration variations of 300 and 400 mg/L. The removal percentages decreased after increasing the concentration from 200 to 300 mg/L and the HRT from 8 hours to 24 hours in zones F and G of Figure 4. The average pseudo-steady state removal percentage of SDBS at HRT of 24 hours for 300 mg/L inlet concentration in MBBR and control reactors were 89% and 48% respectively. The control reactor in that removal percentage collapsed, because it could not be tolerated. Therefore, the metabolic of microbial degradation was precluded (11). The MBBR reactor was kept operating in HRT of 24 hours at the inlet SDBS concentration of 400 mg/L, until the mean percentage of the pseudo-steady state removal reached to 81%. At zones F and G of Figure 4, the COD removal percentage was equal to SDBS removal, which varied almost ± 1.5% in comparison with SDBS removal percentage. MLSS of the primary sludge, prepared from the return sludge line of a wastewater treatment, was measured as 2056 mg/L. At the end of the operation, the MLSS of reactors was measured and the result was 1192 mg/L for the MBBR and 5236 mg/L for the control reactor. It showed that the difference between was due to the existence of biofilms on the media (carriers). During the operation, to prevent foaming, antifoam was applied to reduce the effect of foaming on COD removal, especially in high SDBS concentrations like 100 mg/L and higher. A limited amount of antifoam was used, which was at most about 5-6 droplets for each reactor.
5. Discussion
Biofilm growth on the media surface is related to the properties of the media and connected hydrodynamic situation (17). Visualization of the biofilm surface morphology on the media was conducted by SEM micrographs (Figure 5). The SEM micrograph showed that clean 2H media surface was smooth; but because of biofilm formation on their surface they appeared rough. Sclerotic and homologous biofilm structures on the media can be related to the tautness, originated from the media swirl in the bioreactor (18) sclerotic and homologous biofilm. It can improve the substrate rates and transition of oxygen, decrease the biofilm dispersal ambit, and speedup the biochemical reactions. It is a result of biological degradation enhanced rate (19).
Morphology of the Biofilm Surface Formation on 2H Media, Visualized With Scanning Electron Microscope Micrographs
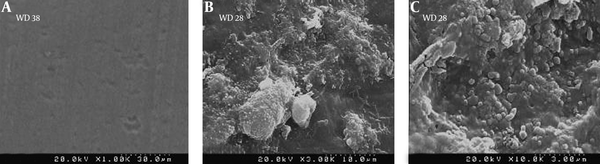
Since for concentrations more than 200 mg/L the MBBR reactor did not achieve the discharge standard for surface water, we can say that for high SDBS concentrations, we should start from the wastewater to obtain the discharge standard and using supplemental treatment after MBBR reactor would be necessary. For treating urban wastewater which contains at most 5 mg/L detergents, biological processes easily remove them, with no different results from MBBR r other bioreactors such as activated sludge or SBBR. To treat wastewaters containing anionic surfactants in the range of 50-200 mg/L, MBBR is recommended because of thr advantages described before and its efficiency for treating anionic surfactants in those ranges. In this experiment, the removal percentage for up to 200 mg/L concentration at HRT of about 24 hours was 99.2%, which was more sufficient than other processes such as electro-Fenton that removed 50 mg/L of anionic surfactant (3), and activated sludge which had 99% removal efficiency for only 5 mg/L detergent in wastewater (7). Production of foam in aerobic processes from anionic surfactants is a main problem, especially when its dose is high. In that case, there can be two suggestions; first, using antifoam materials and second, improving MLSS in the bioreactor to decreases the foam production significantly (20). Considering the media age of more than 20 years as well as the electrical energy reduction which can be applied in other processes for returning the sludge, we can nominate this process as a qualified one for treating wastewaters containing detergents. Furthermore, biological processes are more environment-friendly as they do not include chemical materials and are more flexible and easily handling. They also have lower costs of electrical facilities, professional workers and auxiliary funds. The MBBR process was proposed and experimented in this study to promote the anionic surfactants treatment. Performance of MBBR containing 2H media with 535 m2/m3 specific surface was compared with another reactor without media, testing the effect of biofilm media for biodegradation and COD removal of surfactants in same operational conditions. The MBBR process acted better than the reactor without media in degradation and mineralization of anionic surfactants.
In conclusion, The MBBR process was nominated as a sufficient, cost-effective, easy-operating, and suitable bioprocess, an alternative for treating the pollutants.
Acknowledgements
References
-
1.
Ariapad A, Zanjanchi MA, Arvand M. Efficient removal of anionic surfactant using partial template-containing MCM-41. Desalination. 2012;284:142-9. https://doi.org/10.1016/j.desal.2011.08.048.
-
2.
Ayati B, Nouri RH, Ganjidoust H. Investigation of surfactants removal by moving bed biofilm reactor (MBBR). Environ Sci. 2008;5(4):134-23.
-
3.
Panizza M, Barbucci A, Delucchi M, Carpanese MP, Giuliano A, Cataldo-Hernández M, et al. Electro-Fenton degradation of anionic surfactants. Sep Purif Technol. 2013;118:394-8. https://doi.org/10.1016/j.seppur.2013.07.023.
-
4.
Lara Martín PA, Gómez Parra A, González Mazo E. Reactivity and fate of synthetic surfactants in aquatic environments. Trends Analyt Chem. 2008;27(8):684-95. https://doi.org/10.1016/j.trac.2008.05.005.
-
5.
Lawrence KW, Hung TY, Howard HL, Yapijakis C. Handbook of Industrial and Hazardous Wastes Treatment. New York: Marcel and Dekker; 2004.
-
6.
Cirelli AF, Ojeda C, Castro MJL, Salgot M. Surfactants in sludge-amended agricultural soils: a review. Environ Chem Lett. 2008;6(3):135-48. https://doi.org/10.1007/s10311-0080146-1.
-
7.
Mahvi A, Alavi Nakhjavan N, Nadafi K. Investigating of detergents removal from the ghods county wastewatre treatment by activated sludge process. Sci Horiz. 2004;2:41-36.
-
8.
Zhang S, Wang Y, He W, Wu M, Xing M, Yang J, et al. Impacts of temperature and nitrifying community on nitrification kinetics in a moving-bed biofilm reactor treating polluted raw water. Chem Eng J. 2014;236:242-50. https://doi.org/10.1016/j.cej.2013.09.086.
-
9.
Zhang S, Wang Y, He W, Wu M, Xing M, Yang J, et al. Responses of biofilm characteristics to variations in temperature and NH4(+)-N loading in a moving-bed biofilm reactor treating micro-polluted raw water. Bioresour Technol. 2013;131:365-73. [PubMed ID: 23376201]. https://doi.org/10.1016/j.biortech.2012.12.172.
-
10.
Chu L, Wang J. Comparison of polyurethane foam and biodegradable polymer as carriers in moving bed biofilm reactor for treating wastewater with a low C/N ratio. Chemosphere. 2011;83(1):63-8. [PubMed ID: 21272910]. https://doi.org/10.1016/j.chemosphere.2010.12.077.
-
11.
Aghapour AA, Moussavi G, Yaghmaeian K. Investigating the performance of a novel cyclic rotating-bed biological reactor compared with a sequencing continuous-inflow reactor for biodegradation of catechol in wastewater. Bioresource technology. 2013;138:369-72. https://doi.org/10.1016/j.biortech.2013.03.133.
-
12.
Moussavi G, Mohseni M. The treatment of waste air containing phenol vapors in biotrickling filter. Chemosphere. 2008;72(11):1649-54. [PubMed ID: 18625512]. https://doi.org/10.1016/j.chemosphere.2008.05.040.
-
13.
Moussavi G, Mahmoudi M, Barikbin B. Biological removal of phenol from strong wastewaters using a novel MSBR. Water Res. 2009;43(5):1295-302. [PubMed ID: 19131088]. https://doi.org/10.1016/j.watres.2008.12.026.
-
14.
Standard Methods for the Examination of Water and Wastewater. Washigton DC: American Public Health Association; 2012.
-
15.
Sarfaraz S, Thomas S, Tewari UK, Iyengar L. Anoxic treatment of phenolic wastewater in sequencing batch reactor. Water Res. 2004;38(4):965-71. [PubMed ID: 14769416]. https://doi.org/10.1016/j.watres.2003.10.039.
-
16.
Fernandes H, Jungles MK, Hoffmann H, Antonio RV, Costa RHR. Full-scale sequencing batch reactor (SBR) for domestic wastewater: performance and diversity of microbial communities. Bioresour Technol. 2013;132:262-8. https://doi.org/10.1016/j.biortech.2013.01.027.
-
17.
Pizarro C, Donoso Bravo A, Jeison D, Ruiz Filippi G, Chamy R. Biofilm formation for organic matter and sulphate removal in gas-lift reactors. Electron J Biotechnol. 2011;14(4):235-9. https://doi.org/10.2225/vol14-issue4-fulltext-3.
-
18.
Wasche S, Horn H, Hempel DC. Influence of growth conditions on biofilm development and mass transfer at the bulk/biofilm interface. Water Res. 2002;36(19):4775-84. [PubMed ID: 12448520].
-
19.
Taherzadeh D, Picioreanu C, Horn H. Mass transfer enhancement in moving biofilm structures. Biophys J. 2012;102(7):1483-92. [PubMed ID: 22500748]. https://doi.org/10.1016/j.bpj.2012.02.033.
-
20.
Moussavi G, Heidarizad M. The performance of SBR, SCR, and MSCR for simultaneous biodegradation of high concentrations of formaldehyde and ammonia. Sep Purif Technol. 2011;77(2):187-95. https://doi.org/10.1016/j.seppur.2010.11.028.