Abstract
Background:
Pediatric observational studies have indicated that most critically ill children have low serum selenium level, which is associated with the increased incidence of multiple organ failure and deteriorated clinical outcomes. Selenium plays a key role in the endogenous antioxidant defense mechanism and inflammatory pathways.Objectives:
The present study aimed to assess the effects of high-dose selenium supplementation on the improvement of inflammatory and oxidative stress indices, as well as clinical outcomes, in pediatric patients with severe oxidative stress and inflammation following major gastrointestinal surgeries.Methods:
This prospective, single-blind, randomized, parallel group superiority trial was conducted at the pediatric intensive care unit (PICU) of Akbar Pediatric Hospital in Mashhad, Iran in 2019. Patients were assigned to the supplementation (high-dose selenium: 20 µg/kg/d) and control groups (placebo with the recommended dietary allowance doses of selenium) using stratified blocks. Among 72 eligible critically ill children after gastrointestinal surgery, 66 patients completed the study. Inflammatory markers were measured and compared between the groups, including high-sensitivity C-reactive protein (hsCRP), interleukin 1 beta (IL-1β), prooxidant-antioxidant balance (PAB) assay, and clinical outcomes. Data analysis was performed in SPSS version 20 using the intention-to-treat approach.Results:
Only 14 patients had optimal serum selenium concentrations before the surgery and PICU admission. At the end of the study, 90.6% of the patients (n = 29) in the intervention group and 100% (n = 34) of those in the placebo group had suboptimal serum selenium levels (< 50 ng/mL). Although supplementation with high-dose selenium decreased the inflammatory markers in the post-surgical critically ill children (-18 mg/mL and -37.5 pg/mL for hsCRP and IL-1β, respectively), the administered dose could not improve the serum glutathione peroxidase (GPx) concentrations as the selenium functional marker, as well as the PAB assay as the single test to assess the balance/imbalance of the oxidants and antioxidants simultaneously. Additionally, clinical outcomes such as infections, length of ICU stay, and 28-day mortality did not improve after the intervention.Conclusions:
According to the results, high-dose selenium supplementation (20 µg/kg/d) in the post-surgical critically ill children could improve the serum inflammatory markers. However, the changes were suboptimal with no significant effects of the serum GPx concentrations, antioxidant defense system, and clinical outcomes.Keywords
Inflammation Oxidative Stress Selenium Major Gastrointestinal Surgery Intensive Care Unit Children
1. Background
Major surgeries that control acute damages and induce acute-phase response may stimulate the immune system of the human body, thereby increasing inflammatory mediators and reactive oxygen species (ROS) (1, 2). Although the presence of ROS is essential to the activation of the signaling pathways of antioxidant defense systems, their excessive production leads to multiple complications, such as delayed wound healing, and deterioration of the clinical condition (3, 4).
Children undergoing major surgeries may experience metabolic and hormonal alterations, inflammation, and oxidative stress, which could lead to their admission to the intensive care unit (ICU) (2). The oxidative stress and inflammatory responses following surgical traumas may lead to insulin resistance, cell necrosis and apoptosis, immunosuppression, organ failure, and increased length of ICU and hospital stay (5). Considering the growth and development in childhood, interventions to reduce the rate of inflammation and oxidative stress are of utmost importance during ICU stay (6).
Previous studies have reported the beneficial effects of selenium on inflammatory responses and modulation of the anti-oxidative defense system (3, 7). Serum selenium levels could be used as a predictor of survival in the ICU since its higher levels (close to the normal range) are associated with decreased length of hospital stay and mortality, while low levels of serum selenium are associated with organ failure, increased length of ventilator dependency, ICU, and hospital stay, and increased 28-day mortality (2, 4, 8, 9).
According to the literature, serum selenium levels in systemic inflammations (e.g., major surgeries) are likely to decrease for various reasons, such as endothelial injury, altered metabolic processes, redistribution of selenium, and insufficient selenium intake (8, 10, 11). However, serum selenium levels may decrease partly due to systemic inflammation. Therefore, systemic inflammatory markers should be considered in the interpretations in this regard (8, 12).
According to the guidelines of the Australian Association of Intravenous and Intestinal Nutrition, the daily requirement of selenium may increase in the acute phase following major surgeries due to short-term metabolic and antioxidant maintenance needs. Consequently, the patients may benefit from high-dose selenium administration, while the approved dosage for such conditions remains unclear (13).
2. Objectives
To the best of our knowledge, no studies have evaluated the use of selenium supplementation at higher doses than the physiological values in critically ill children undergoing major surgeries. The present study aimed to investigate the effects of high-dose selenium supplementation on oxidative stress and inflammatory factors in critically ill pediatric patients after major gastrointestinal surgeries.
3. Methods
3.1. Ethical Approval and Recruitment
The study protocol was approved by the Ethics Committee of Mashhad University of Medical Sciences in Mashhad, Iran. This was a single-blind, randomized, parallel-group superiority clinical trial carried out at the pediatric intensive care units (PICUs) of Akbar Pediatric Hospital in Mashhad city during March 2019-January 2020.
The patients were aged 0 - 10 years and underwent major GI surgeries, including esophageal atresia, intestinal atresia, biliary atresia, intestinal obstruction, omphalocele, Hirschsprung’s disease, and gastric pull-up. The patients were admitted to the ICU after major GI surgeries due to their medical indications and were therefore considered eligible for recruitment. The exclusion criteria of the study were as follows: (1) not receiving nutritional support care within the first 24 - 48 hours postoperatively; (2) earlier PICU discharge than five days after the surgery; (3) sensitivity to sodium selenite or its contents; (4) baseline (preoperative) serum selenium concentrations of > 106 μg/L; (5) diagnosis of autoimmune disorders, cancer, severe hepatic failure, renal failure, HIV infection, and severe sepsis upon admission; (6) history of chemotherapy and radiotherapy within the past month; (7) laboratory/clinical symptoms of selenium intoxication; (8) severe and active bleeding and (9) preterm neonates.
3.2. Sample Size and Randomization
Considering the lack of similar studies, 32 participants were assigned to each group with 95% confidence intervals, 80% test power, and 70% effect size to achieve significant results. With an expected 10% sample loss, the final sample size was estimated to be 35 participants per each group (main outcome: hs-CRP) (14).
Block stratified randomization was used for the random allocation of the samples to minimize the potential confounding factors. Moreover, randomization in the assigning of the samples to the intervention and control groups was performed based on age, type of surgery (upper and lower GI tract), and nutritional status classification (WHO z scores).
3.3. Dose Determination and Safety Monitoring
The research team established several expert panels on the dose determination at the beginning of the study, and there were numerous concerns in this regard. The optimal dose for short-term selenium supplementation in the children was estimated using the NAIR equation (equivalent dose for children: 48 µg/kg/d) (15-17).
Considering the estimated optimal selenium dose of 48 µg/kg/d based on the NAIR formula and in terms of the established recommended dietary allowance (RDA) of selenium 2 µg/kg/d (15), studies are lacking on high-dose selenium supplementation (> 10 μg/kg/d) in children (18). In this regard, the selenium dose has been suggested to be within the range of 10 - 48 µg/kg/d, and therefore in the current study, 20 µg/kg/d of selenium is recommended for children admitted to the ICU after GI surgeries.
The neonatologists, pediatric critical care subspecialists, and corresponding researchers were aware of the random allocation of the data. Selenium intoxication was evaluated based on various clinical signs and symptoms in daily visits. The procedures had to be discontinued in case the serum selenium quantities were higher than 0.813 μg/mL (15).
3.4. Interventions
Initially, a customized step-by-step protocol developed and implemented for the nutritional support of the patients in line with the recent guidelines for critically ill children (19, 20). Anthropometric indices were measured at the outset of the study. In addition, the daily measurement of body weight was performed during the ICU stay of the patients. As for the oxidative stress status and inflammatory markers, venous blood (3 mL) and urine samples (2 mL) were obtained on day -1 (before surgery), day +1, and upon discharge from the ICU (minimum: day five, up to day 10 preoperatively). All the patients were visited and monitored daily by clinical nutritionists. Moreover, not receiving concomitant supplementations (e.g., vitamin C, vitamin E, beta-carotene, zinc, copper, chromium, and manganese) was also monitored by the clinical nutritionists.
All the participants with enteral or parenteral nutrition received the RDA dose of selenium. In the intervention group, selenium supplementation was provided at the dose of 20 μg/kg/day (RDA dose). Selenium was injected intravenously, in the form of sodium selenite at the concentration of 50 μg/mL within 60 minutes. In the control group, placebo (5% dextrose serum) was administered in vials similar to the supplements with a similar form and volume. The supplement/placebo was administered to the patients up to 10 days, until discharge from the ICU or death.
To prevent fluid overload in the patients, the volume of the administered fluid in both groups was subtracted from the daily serum volume of the patients.
The patients, their parents, and the nursing team were blinded to the supplement/placebo assignments. Since the patients received the placebo/supplementation by nurses under the supervision of the clinical nutritionists, adherence assessment was not required. Furthermore, an independent academic professor in pediatrics monitored the data and audited the trial at one-month intervals.
3.5. Assessments
The body weight of the patients was measured using a digital scale (Balas) with an accuracy of 10 grams. Length and height were also measured using a portable infantometer and stadiometer for the children aged < 24 and > 24 months, respectively. However, using the standard methods of length and height measurement was not possible in all the cases, and a predictive equation was applied to estimate the stature based on the ulna length (21, 22).
3.6. Laboratory Assays
Serum and urine selenium were analyzed using an atomic absorption spectrometer (Perkins-Elmer HGA-700 Graphite Furnace, USA). Serum Interleukin 1 beta (IL-1β) and glutathione peroxidase (GPx) were assessed using Zellbio ELISA kits (Germany). In addition, high-sensitivity C-reactive protein (hsCRP) was evaluated using a biochemical auto-analyzer (Premium Biolis 24i, Japan). The prooxidant-antioxidant balance (PAB) assay was also carried out using the Stat Fax 2100 microplate reader (AWARENESS, USA).
3.7. Outcomes Measures
The primary outcomes included the PAB status, IL-1β, and hsCRP, which were measured at baseline (day -1 and before the surgery) and upon discharge from the PICU (day 10; minimum: five days).
The secondary outcomes were also measured on day -1 (preoperatively), day +1, and upon discharge from the PICU (day 10; postoperatively; serum GPx, serum and urine selenium). The final values and changes during the study were compared between the intervention and control groups. The other clinical outcomes in the study groups were also recorded, including the duration of ventilator dependency, length of ICU and hospital stay, possible infections (e.g., nosocomial infections and ventilator-associated pneumonia), and 28-day mortality.
3.8. Statistical Analyses
The intragroup and intergroup data analysis was performed in SPSS version 20 using the intention-to-treat approach at the significance level of less than 0.05 with 95% confidence interval.
4. Results
Figure 1 depicts the enrollment, randomization, and completion of the study outcomes.
The enrollment, randomization and completion of the study outcomes
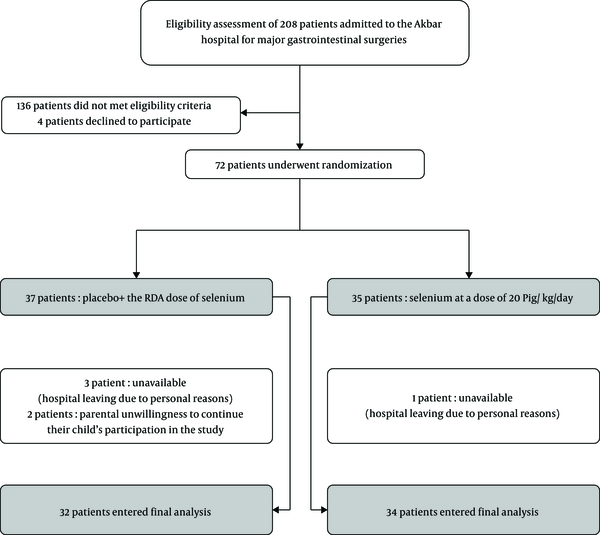
Table 1 shows the demographic characteristics of the patients upon admission, as well as their nutritional status and surgical diagnosis. As can be seen, the intervention and placebo groups had no significant differences in terms of age, body weight, height, gender, nutritional status upon ICU admission, and surgical diagnosis (P > 0.05).
Demographic Characteristics and Nutritional Status of Patients Upon ICU Admission and Their Surgical Diagnosis
Variable | Intervention (N = 34) | Control (N = 32) | P Value |
---|---|---|---|
Age (month) | 2 (14.05) | 1.25 (11.88) | 0.7a |
Weight (kg) | 4.8 (6.1) | 3.7 (4.1) | 0.5a |
Height (cm) | 58 (24.6) | 54 (22.8) | 0.5a |
Gender (female) | 17 (50) | 12 (37.5) | 0.3b |
Nutritional status upon admissionc | |||
Normal | 25 (73.5) | 28 (87.5) | 0.15b |
Moderate malnutrition | 5 (14.7) | 1 (3.1) | 0.1b |
Severe malnutrition | 4 (11.7) | 3 (9.3) | 0.7b |
Surgical diagnosis | |||
Esophageal atresia | 4 (11.7) | 5 (15.6) | 0.6b |
Congenital diaphragmatic hernia | 1 (2.9) | 1 (3.1) | 0.9b |
Gastric pull-up | 1 (2.9) | 2 (6.25) | 0.5b |
Intestinal atresia | 11 (32.3) | 6 (18.75) | 0.2b |
Biliary atresia | 1 (2.9) | 1 (3.1) | 0.9b |
Hirschsprung disease | 13 (38.2) | 11 (34.3) | 0.2b |
Obstruction | 3 (8.8) | 6 (18.75) | 0.2b |
The nutritional adequacy and clinical outcomes of the patients are presented in Table 2. According to the obtained results, the study groups had no significant differences in terms of energy and protein adequacy during ICU stay and the number of intervention days (P = 0.3, P = 0.9, and P = 0.5, respectively). Additionally, the clinical outcomes (nosocomial infections, number of ventilator dependency days, ICU mortality, and 28-day mortality) were not significantly different between the placebo and supplementation groups (P > 0.05).
Nutritional Adequacy, Number of Intervention Days, and Clinical Outcomes in Study
Variable | Intervention (N = 34) | Control (N = 32) | P Value |
---|---|---|---|
Energy adequacya | 26 (76.4) | 21 (65.6) | 0.3b |
Protein adequacya | 31 (91.1) | 29 (90.6) | 0.9b |
Number of intervention days | 6.5 (5) | 6.5 (5) | 0.5c |
Number of ventilator dependency days | 1 (2.5) | 1.5 (3) | 0.5c |
PICU length of stay (day) | 6.5 (5) | 6.5 (7.25) | 0.8c |
Hospital length of stay (day) | 8.5 (6) | 8 (8) | 0.8c |
Infections | 1 (2.9) | 4 (12.5) | 0.14b |
ICU mortality | 1 (2.9) | 3 (9.3) | 0.27b |
28-day mortality | 1 (2.9) | 2 (6.2) | 0.5b |
Only 21% of the participants (14 patients) had optimal selenium concentration (> 50 ng/mL) at the beginning of the study. The median [interquartile range (IQR)] of serum selenium concentration at the end of the supplementation period in the intervention group was 37 ng/ml (16), which was significantly higher (P < 0.001) compared to the median (IQR) of serum selenium concentration in the placebo group [32 (5.4) ng/mL]. At the end of the placebo infusion and selenium supplementation, the number of the children with the minimum serum selenium level for adequate selenium function (> 50 ng/mL) was three in the intervention group and zero in the placebo group (P = 0.08). According to the information in Table 3, urinary selenium and serum GPx concentrations had no significant differences between the study groups on days -1 and +1 and at the time of PICU discharge.
Serum and Urinary Selenium and Serum Glutathione Peroxidase Enzyme Concentrations in Intervention and Control Groups During Study
Variable | Intervention (N = 34) | Control (N = 32) | P Value |
---|---|---|---|
Serum Selenium Concentrationsa (ng/mL) | |||
Day -1 (before surgery) | 37.5 (11.2) | 35.5 (9.9) | 0.3 |
Day +1 (after surgery) | 36 (8) | 38 (18) | 0.5 |
PICU Discharge | 37 (16) | 32 (5.4) | < 0.001 |
Urinary Selenium Concentrationsa (ng/mL) | |||
Day -1 (before surgery) | 0.98 (1.2) | 1.1 (0.68) | 0.14 |
Day +1 (after surgery) | 0.83 (1.6) | 1.05 (0.75) | 0.09 |
PICU Discharge | 0.9 (1.4) | 1.2 (0.7) | 0.07 |
Serum Glutathione Peroxidase Concentrationsb (unit/mL) | |||
Day -1 (before surgery) | 324.8 ± 192.6 | 352.7 ± 177.8 | 0.5 |
Day +1 (after surgery) | 239.3 ± 195.9 | 248.3 ± 155.9 | 0.8 |
PICU Discharge | 340.5 ± 269.3 | 268.5 ± 182.8 | 0.2 |
The results of linear regression analysis indicated a significant association between serum selenium concentration and GPx activity in stress-free conditions (day -1: P < 0.001; Pearson’s correlation-coefficient = -0.42). Despite the observed correlation at baseline (preoperatively), no significant correlation was observed between serum selenium concentration and GPx activity after the placebo infusion/supplementation, following the acute phase of stress caused by the major GI surgeries. Although no significant difference was observed between the groups in terms of serum GPx concentration, the intragroup changes in this index were considered significant in the placebo group (P = 0.03), while non-significant in the supplementation group (P = 0.055).
The results of PAB assay indicated no significant difference between the groups in terms of the hydrogen peroxide rate in the standard solution at the beginning and end of the study (P = 0.6 and P = 0.9, respectively).
Table 4 shows the serum concentrations of hsCRP and IL-1β in the study groups. Accordingly, the difference in the serum concentrations of hsCRP in the supplementation group was more significant compared to the control group at the end of the placebo infusion/selenium supplementation (P = 0.01). Furthermore, a significant difference was observed between the intervention and control groups in the hsCRP concentration at the end of the placebo infusion/supplementation and before surgery, with less significance in the supplementation group (P = 0.007).
Serum Concentrations of hsCRP and IL-1β in Intervention and Control Groups
Variable | Intervention (N = 34) | Control (N = 32) | P Value |
---|---|---|---|
Serum concentrations of hsCRP (mg/mL) | |||
Before surgery | 1.5 (0.7) | 1.7 (1.8) | 0.3a |
PICU discharge | 19.7 (34.7) | 47 (22.4) | 0.01a |
Before surgery-PICU discharge | 13.2 (29.5) | 43.4 (42.8) | 0.007a |
P value of concentration changes during studyb | < 0.001 | < 0.001 | - |
Serum concentrations of IL-1β (pg/mL) | |||
Before surgery | 64.2 ± 32 | 58.8 ± 25.9 | 0.4c |
PICU discharge | 63.9 ± 43.8 | 98.1 ± 78.8 | 0.01c |
Before surgery-end of intervention | -0.34 ± 44.8 | 39.3 ± 75.6 | 0.03c |
P value of concentration changes during studyd | 0.9 | 0.006 | - |
After adjusting the effect of preoperative hsCRP concentration as the confounding factor, the difference between the groups was considered significant (P = 0.009) after the placebo infusion/selenium supplementation (Table 5). The hsCRP concentration decreased by 18 mg/ml on average in the intervention group compared to the control group at the end of the placebo infusion/supplementation.
Significant Differences in hs-CRP and IL-1β Concentrations After Placebo Infusion/Selenium Supplementation and Controlling of Preoperative Concentrations
Regression Coefficient | Standard Error | T | P Value | |
---|---|---|---|---|
hs-CRP | ||||
Intervention | -18.622 | 6.84 | 2.7 | 0.009 |
Control | 0 | |||
Concentration of hs-CRP at PICU discharge (mg/mL) | -0.14 | 0.412 | 0.35 | 0.7 |
IL-1β | ||||
Intervention | -37.5 | 15.1 | 2.4 | 0.016 |
Control | 0 | |||
Concentration of IL-1β at PICU discharge (pg/mL) | 0.61 | 0.26 | 2.3 | 0.02 |
At the end of the placebo infusion/selenium supplementation, the mean serum concentration of IL-1β was 43.8 (SD = 63.9) pg/mL in the supplementation group, which was significantly different compared to the control group [78.8 (SD = 98.1) pg/mL; P = 0.03]. In addition, the results of the paired t-test indicated that the intragroup changes in the IL-1β concentration were significant in the control group during the study (P = 0.006). Evaluation of the significant difference in IL-1β concentration at the end of the placebo infusion/selenium supplementation by adjusting the effect of preoperative IL-1β concentrations demonstrated a significant difference between the intervention and control groups in this regard (P = 0.016). At the end of the placebo infusion/selenium supplementation, the IL-1β concentrations in the intervention group decreased by 37.5 pg/mL on average compared to the control group.
5. Discussion
To the best of our knowledge, the present study is the first investigation regarding the effects of high-dose selenium supplementation on the inflammatory markers and oxidative stress status in children admitted to the ICU after major GI surgeries. Considering that the lowest concentration for adequate selenium function (i.e., sufficient expression of selenoproteins) in all the age groups has been reported to be 50 ng/ml in previous studies, only 21% (N = 14) of our patients had an optimal selenium concentration at the beginning of the study (23). At the end of the placebo infusion/selenium supplementation, three children in the intervention group and none of the children in the placebo group had the minimum serum selenium level for adequate selenium function, which was close to the significance level (P = 0.08). In a study conducted by Sakr et al. on adult surgical ICU patients, 92% of the patients had lower serum selenium levels than the normal range, and serum selenium levels decreased during ICU stay, while the most significant reduction was observed in the patients with organ failure, especially those with infections. Moreover, lower serum selenium levels were associated with a higher risk of cell damage, organ failure, and mortality (24).
The cause of decreased selenium in the acute phase of major surgical patients may be multifactorial, and the main influential factors in this regard include increased selenium requirement in the acute phase, decreased binding proteins (mainly albumin) due to increased requirement or redistribution, blood dilution due to excessive fluid therapy, the incomplete replacement of the lost biological fluids that are abundant in micronutrients, and redistribution due to the selective uptake of selenium by the tissues with metabolically enhanced requirements (e.g., GPx synthesis) (25). According to the results of the present study and previous studies in Iran, selenium deficiency prior to major surgeries cannot be overlooked in various populations (25, 26).
In the current research, the low urinary selenium concentrations (< 1.5 ng/mL) and lack of a significant difference between the groups in this regard may indicate that 20 mg/kg of daily supplementation was not more than the daily requirements in the acute phase of stress following major GI surgeries in the critically ill children. Although the median (interquartile range) of the serum selenium concentration at the end of the placebo infusion/selenium supplementation was significantly higher in the intervention group compared to the control group, the increased concentration in the intervention group was below the minimum level of sufficient selenium to function, which could be due to the insufficient dose of supplementation.
According to the literature, selenium supplementation reduces nuclear factor kappa B and tumor necrosis factor α (TNF-α) through affecting antioxidant mechanisms (27). In patients with severe systemic inflammation, the short-term infusion of high doses of selenium has been reported to increase the expression of selenoprotein P, which leads to the promotion of endothelial function and reduction of organ dysfunction in critically ill patients (28). According to the results of the present study, high-dose selenium supplementation had no effect on the antioxidant defense system in the acute phase of stress. On the other hand, serum selenium level and GPx activity were correlated in the preoperative phase, while no such correlation was observed after the intervention. In addition, no significant difference was observed between the groups. Based on the intragroup changes in the supplementation group and significant intragroup changes in the control groups, it could be concluded that the suboptimal dose for the effectiveness of selenium supplementation in the translation of selenoproteins also affected the antioxidant defense.
In the current research, the evaluation of serum hsCRP and IL-1β concentrations demonstrated that supplementation with high-dose selenium in the acute phase of stress could decrease the inflammatory markers in the critically ill children postoperatively. The serum concentrations of hsCRP and IL-1β in the intervention group were respectively 18 and 37.5 units lower compared to the placebo group following selenium supplementation.
Although we could not find any clinical trials investigating the possible anti-inflammatory effects of high doses of selenium in critically ill children, several observational studies in pediatrics have reported the reduction of serum selenium levels and its association with the severity of inflammation and oxidative damage, which is in line with the results of the present study (2, 10). In addition, the previous studies conducted on adults have denoted that selenium deficiency exacerbates the inflammatory responses in critically ill patients, while selenium supplementation could reduce inflammatory interleukins, CRP, and TNF-α through the modulation of inflammatory pathways, regulating the function of eicosanoids synthesis pathways (increased leukotriene and prostacyclin synthesis, decreased prostaglandin and thromboxane synthesis), and reducing the expression of cytokines and adhesion molecules (27, 29). In addition, selenium may promote apoptosis in the circulating cells in which pro-inflammatory mechanisms are activated (29).
Although the levels of triodothyronine (T3) is low and reverse T3 is elevated, and also TSH and thyroxin (T4) are low, parallel with the low plasma selenium levels and severity of the disease in critical illnesses, it is reported that selenium supplementation play an indirect role thorough the reduction of cytokine release in increasing the expression levels of thyroid hormones (30). Therefore, thyroid hormones may play a role in the evolution of the studied critically ill children; however, further studies are required to investigate the exact relation between selenium and thyroid selenoenzyme expression levels via critical illnesses.
The findings of the current research indicated no significant differences between the intervention and placebo groups in terms of clinical outcomes, including nosocomial infections, number of ventilator dependency days, ICU mortality, and 28-day mortality. To date, no other randomized or non-randomized clinical trial has evaluated the effects of high-dose selenium supplementation on clinical outcomes in critically ill children. In the CRISIS study, low-dose selenium supplementation (40 μg/d in children aged 1 - 3 years, 100 μg/d in children aged 3 - 5 years, 200 μg/d in children aged 5 - 12 years, and 400 μg/d in children aged > 12 years) was administered as a cocktail containing selenium, zinc, glutamine, and metoclopramide to 293 critically ill children, and no improvement was observed in their clinical outcomes.
According to a meta-analysis conducted by Zhao et al. (2019), the efficacy of high-dose selenium supplementation was assessed in the adult patients admitted to ICUs, which had no significant effects on the incidence of infections, number of ventilator dependency days, and 28-day mortality. However, the mentioned study indicated that selenium could reduce the length of hospital stay and total mortality. It is notable that the study by Zhao et al. was mainly focused on the need for more precise randomized clinical trials in order to address various clinical questions (20). Another meta-analysis in this regard was conducted by Manzanares et al. (2016), and selenium efficacy was not confirmed in the mentioned clinical outcomes (27), which may be due to the differences in the inclusion criteria of these studies.
Although studies regarding acute stress in adults have confirmed the safety and efficacy of approximate selenium doses of 15 - 25 micrograms per kilogram of the body weight in the acute phase (1,000 - 1,600 μg/d), the findings regarding higher and lower doses of this element have not shown efficacy in the improvement of clinical outcomes, and the higher doses (2,000 μg/d) have mainly been associated with increased mortality due to the possible prooxidant effects (26). The results of the present study indicated the insufficient dose provided to the critically ill children to have the minimum serum selenium level for proper function in the acute phase of stress.
Some of the limitations of the current research were the impossibility of conducting a double-blind clinical trial and examination of serum selenium in all the measurements to evaluate the selenium status in the patients due to the invasive nature of tissue biopsy and no evaluation of oxidant and antioxidant enzymes (e.g., malondialdehyde) and total antioxidant capacity separately.
5.1. Conclusion
According to the results, selenium supplementation in the critically ill children in the acute phase of stress caused by major GI surgeries at the daily dose of 20 micrograms per kilogram of the body weight could significantly increase the serum selenium levels. However, the supplementation of higher doses is recommended as the increased selenium levels were insufficient in terms of effect. In addition, the increased serum selenium levels could not promote selenium antioxidant function, while the administered dose reduced inflammatory markers. In conclusion, it is suggested that more clinical trials be conducted to determine the proper dose and accurate indications of selenium supplementations for achieving adequate selenium function in critically ill children.
Acknowledgements
References
-
1.
Reding KW, Cain KC, Jarrett ME, Eugenio MD, Heitkemper MM. Relationship between patterns of alcohol consumption and gastrointestinal symptoms among patients with irritable bowel syndrome. Am J Gastroenterol. 2013;108(2):270-6. [PubMed ID: 23295280]. [PubMed Central ID: PMC3697482]. https://doi.org/10.1038/ajg.2012.414.
-
2.
Broman M, Lindfors M, Norberg A, Hebert C, Rooyackers O, Wernerman J, et al. Low serum selenium is associated with the severity of organ failure in critically ill children. Clin Nutr. 2018;37(4):1399-405. [PubMed ID: 28689669]. https://doi.org/10.1016/j.clnu.2017.06.014.
-
3.
Aviello G, Knaus UG. ROS in gastrointestinal inflammation: Rescue Or Sabotage? British journal of pharmacology. 2017;174(12):1704-18. [PubMed ID: 26758851]. https://doi.org/10.1111/bph.13428.
-
4.
Leite HP, Nogueira PCK, Iglesias SB, Oliveira SV, Sarni ROS. Increased plasma selenium is associated with better outcomes in children with systemic inflammation. Nutrition. 2015;31(3):485-90. https://doi.org/10.1016/j.nut.2014.09.008.
-
5.
Stoppe C, Schalte G, Rossaint R, Coburn M, Graf B, Spillner J, et al. The intraoperative decrease of selenium is associated with the postoperative development of multiorgan dysfunction in cardiac surgical patients. Crit Care Med. 2011;39(8):1879-85. [PubMed ID: 21460705]. https://doi.org/10.1097/CCM.0b013e3182190d48.
-
6.
Abad-Jorge A. Nutrition Management of the Critically Ill Pediatric Patient: Minimizing Barriers to Optimal Nutrition Support. ICAN: Infant, Child, & Adolescent Nutrition. 2013;5(4):221-30. https://doi.org/10.1177/1941406413492821.
-
7.
Roman M, Jitaru P, Barbante C. Selenium biochemistry and its role for human health. Metallomics. 2014;6(1):25-54. [PubMed ID: 24185753]. https://doi.org/10.1039/c3mt00185g.
-
8.
Dao DT, Anez-Bustillos L, Cho BS, Li Z, Puder M, Gura KM. Assessment of Micronutrient Status in Critically Ill Children: Challenges and Opportunities. Nutrients. 2017;9(11):1185. [PubMed Central ID: PMC5707657]. https://doi.org/10.3390/nu9111185.
-
9.
Manzanares W, Langlois PL, Hardy G. Update on antioxidant micronutrients in the critically ill. Curr Opin Clin Nutr Metab Care. 2013;16(6):719-25. [PubMed ID: 24100672]. https://doi.org/10.1097/MCO.0b013e32836599e5.
-
10.
de Oliveira Ulbrecht MO, Goncalves DA, Zanoni LZG, do Nascimento VA. Association Between Selenium and Malondialdehyde as an Efficient Biomarker of Oxidative Stress in Infantile Cardiac Surgery. Biol Trace Elem Res. 2019;187(1):74-9. [PubMed ID: 29754283]. https://doi.org/10.1007/s12011-018-1378-y.
-
11.
Iglesias SB, Leite HP, Paes AT, Oliveira SV, Sarni RO. Low plasma selenium concentrations in critically ill children: the interaction effect between inflammation and selenium deficiency. Crit Care. 2014;18(3):R101.
-
12.
Carcillo JA, Dean J, Holubkov R, Berger J, Meert KL, Anand KJS, et al. The Randomized Comparative Pediatric Critical Illness Stress-Induced Immune Suppression (CRISIS) Prevention Trial. Pediatric Critical Care Medicine. 2012;13(2):165-73. [PubMed Central ID: PMC3302948]. https://doi.org/10.1097/PCC.0b013e31823896ae.
-
13.
Johnson CD, Kudsk KA, Fukatsu K, Renegar KB, Zarzaur BL. Route of nutrition influences generation of antibody-forming cells and initial defense to an active viral infection in the upper respiratory tract. Annals of surgery. 2003;237(4):565.
-
14.
Munro BH. Statistical Methods for Health Care Research. Lippincott Williams & Wilkins; 2001. 128 p.
-
15.
Bechard L, Ziegler J, Duggan C. Is Energy Expenditure of Infants Predictable After Surgery?A Review of the Evidence. Ican: Infant, Child, & Adolescent Nutrition. 2010;2(3):170-6. https://doi.org/10.1177/1941406410370850.
-
16.
Acar-Tek N, Agagunduz D, Celik B, Bozbulut R. Estimation of Resting Energy Expenditure: Validation of Previous and New Predictive Equations in Obese Children and Adolescents. J Am Coll Nutr. 2017;36(6):470-80. [PubMed ID: 28749749]. https://doi.org/10.1080/07315724.2017.1320952.
-
17.
Marra M, Montagnese C, Sammarco R, Amato V, Della Valle E, Franzese A, et al. Accuracy of Predictive Equations for Estimating Resting Energy Expenditure in Obese Adolescents. The Journal of Pediatrics. 2015;166(6):1390-13960. https://doi.org/10.1016/j.jpeds.2015.03.013.
-
18.
Aggarwal R, Gathwala G, Yadav S, Kumar P. Selenium supplementation for prevention of late-onset sepsis in very low birth weight preterm neonates. Journal of tropical pediatrics. 2016;62(3):185-93.
-
19.
Domellöf M, Szitanyi P, Simchowitz V, Franz A, Mimouni F, Braegger C, et al. ESPGHAN/ESPEN/ESPR/CSPEN guidelines on pediatric parenteral nutrition: Iron and trace minerals. Clinical Nutrition. 2018;37(6):2354-9. https://doi.org/10.1016/j.clnu.2018.06.949.
-
20.
Botran M, Lopez-Herce J, Mencia S, Urbano J, Solana MJ, Garcia A. Enteral nutrition in the critically ill child: comparison of standard and protein-enriched diets. J Pediatr. 2011;159(1):27-320. [PubMed ID: 21429514]. https://doi.org/10.1016/j.jpeds.2011.02.001.
-
21.
Ozaslan A, Koc S, Ozaslan I, Tugcu H. Estimation of stature from upper extremity. Mil Med. 2006;171(4):288-91. [PubMed ID: 16673740]. https://doi.org/10.7205/milmed.171.4.288.
-
22.
Weidauer L, Wey H, Slater H, Moyer-Mileur L, Specker B. Estimation of length or height in infants and young children using ulnar and lower leg length with dual-energy X-ray absorptiometry validation. Developmental medicine and child neurology. 2014;56(10):995-1000. [PubMed ID: 24840474]. https://doi.org/10.1111/dmcn.12491.
-
23.
Combs GFJ. Biomarkers of selenium status. Nutrients. 2015;7(4):2209-36. [PubMed ID: 25835046]. https://doi.org/10.3390/nu7042209.
-
24.
Sakr Y, Reinhart K, Bloos F, Marx G, Russwurm S, Bauer M, et al. Time course and relationship between plasma selenium concentrations, systemic inflammatory response, sepsis, and multiorgan failure. British Journal of Anaesthesia. 2007;98(6):775-84. https://doi.org/10.1093/bja/aem091.
-
25.
Nouarie M, Pourshams A, Kamangar F, Sotoudeh M, Derakhshan MH, Akbari MR, et al. Ecologic study of serum selenium and upper gastrointestinal cancers in Iran. World Journal of Gastroenterology. 2004;10(17):2544-6. [PubMed ID: 15300901]. https://doi.org/10.3748/wjg.v10.i17.2544.
-
26.
Nazemi L, Nazmara S, Eshraghyan MR, Nasseri S, Djafarian K, Yunesian M, et al. Selenium status in soil, water and essential crops of Iran. Iranian journal of environmental health science & engineering. 2012;9(1):11. [PubMed ID: 23369199]. https://doi.org/10.1186/1735-2746-9-11.
-
27.
Manzanares W, Lemieux M, Elke G, Langlois PL, Bloos F, Heyland DK. High-dose intravenous selenium does not improve clinical outcomes in the critically ill: a systematic review and meta-analysis. Critical Care. 2016;20:356. [PubMed Central ID: PMC5084353]. https://doi.org/10.1186/s13054-016-1529-5.
-
28.
Hardy G, Hardy I, Manzanares W. Selenium supplementation in the critically ill. Nutr Clin Pract. 2012;27(1):21-33. [PubMed ID: 22307489]. https://doi.org/10.1177/0884533611434116.
-
29.
Mosaed R, Ghadimi MH, Mojtahedzadeh M, Ahmadi A. What is the Optimal Dose of Selenium and Other Antioxidants in Critically Ill Patients? J Arch Mil Med. 2015;3(1). e26080. https://doi.org/10.5812/jamm.26080.
-
30.
Gärtner R. Selenium and thyroid hormone axis in critical ill states: An overview of conflicting view points. Journal of Trace Elements in Medicine and Biology. 2009;23(2):71-4. https://doi.org/10.1016/j.jtemb.2009.01.001.