Abstract
Background:
Apoptosis is proposed as a possible mechanism for diabetes-induced hippocampal neuronal cell death. Numerous studies have suggested that the therapeutic properties of plants, such as antioxidant and anti-apoptotic, are effective in improving the complications of diabetes in the hippocampus.Objectives:
This study aimed to evaluate the anti-apoptotic properties of Peganum harmala (P. harmala) in the brain hippocampal cells of diabetic rats.Methods:
In this experimental study, 48 male Wistar rats were divided into six groups (n = 8) as follows: Control (C), diabetic (D), harmine (H), diabetic plus harmine (DH), seed extract (S), and diabetic plus seed extract (DS). A single dose of streptozotocin (STZ) (60 mg/kg) was enough to cause diabetes. Seed extract and harmine were given at 150 mg/kg and 6.5 mg/kg, respectively (daily by oral gavage for 28 days). The glucose levels in the blood were measured, and the histological staining of the hippocampus was examined. Percentages of apoptotic hippocampal cells were identified with flow cytometry. Bax and Bcl-2 expression was assayed via Real time- polymerase chain reaction (PCR) and Western blot.Results:
In DH (P = 0.001) and DS (P = 0.01) rats, the mean fasting blood glucose level significantly reduced compared with the D group. Bax and Bcl-2 expression at both mRNA and protein levels significantly differed between the D group and other groups (P = 0.01). Harmine and the seed extract considerably reduced the Bax/Bcl-2 ratio in the hippocampal cells compared to the D group (P = 0.001).Conclusions:
Streptozotocin-induced apoptosis in the hippocampus of diabetic rats was reduced by administering the seed extract of Peganum harmala. The P. harmala seed extract and its active ingredient, harmine, could be used as anti-apoptotic drugs.Keywords
Peganum harmala Harmine Hippocampus Apoptosis Gene Expression
1. Background
Diabetes mellitus (DM) is a metabolic disorder with severe chronic complications, including myopathy, retinopathy, and neuropathy (1). Despite the view that there is no relationship between the central nervous system and diabetes mellitus (2), investigations presented evidence suggesting the influences of this disease on some parts of the nervous system (3). Diabetes has been proposed to affect the central nervous system (4). The hippocampus is a specific target of changes inside the central nervous system, which is relevant to diabetes (5). Hyperglycemia has been reported to increase neuronal damage in the hippocampus and subarachnoid hemorrhage, increasing neuronal necrosis and infarction size (6).
Apoptosis is responsible for several diabetes complications, such as diabetic neuropathy (7). In diabetes mellitus, the pathology of the central nervous system is related to hyperglycemia and the lack of insulin (8). The growing oxidative stress excessively releases free radicals, which is the main reason for neoplastic apoptosis (9, 10). Hippocampal oxidative stress is associated with increased lipid peroxidation products and reduced activity levels of endogenous antioxidant enzymes (11). Apoptosis is a dormant process to kill the hippocampal neuronal cells and synapses caused by hyperglycemia (12). It has been indicated that diabetes induction by streptozotocin (STZ) significantly increases the Bax expression in the hippocampus. Still, there is a significant decrease in Bcl-xl and Bcl-2 expression in protein and mRNA levels (13).
Research has indicated that treatment with antioxidants decreases the complications caused by diabetes (14). Peganum harmala L. (P. harmala), the wild-growing medical herb, consists of alkaloids including Harman, norharman, harmine, harmaline, and harmalol (15). Qualities such as anti-microbial, anti-fungal, anti-plasmodial, antioxidant, anti-genotoxic, and anti-tumor properties are attributed to P. harmala seeds (16). Furthermore, harmine has been discovered to have therapeutic impacts on kidney failure and protect it from acute damage in male mice (17).
There are few studies on the anti-apoptotic effect of P. harmala seed extracts (18). Recently, Kajbaf et al. have shown that the leaf extract of P. harmala cannot inhibit apoptosis by reducing caspase-3 expression in the diabetic kidney model (19). Also, it is demonstrated that the seed extract of P. harmala is more effective in controlling diabetes and apoptosis in the beta cells of the pancreas (20). However, Magdy et al. revealed that Harmal seed extract has potent antidiabetic activity in streptozotocin-induced diabetic rats and can be used as a dietary supplement by diabetic patients (21).
To date, there are few studies on the oral administration of P. harmala for the treatment of diabetes, and very few research works have reported its effect on the brain, especially the hippocampus, which can be damaged by diabetes. Whether P. harmala extract and its active ingredient (harmine) can protect brain cells from apoptosis induction is the main topic of investigation.
2. Objectives
The study investigated the effect of the oral administration of P. harmala extracts and its active ingredient on diabetes-induced apoptosis in the hippocampal cells of rats with diabetics and normal rats.
3. Methods
3.1. Chemicals
Drugs including harmine (7-Methoxy-1-methyl-9H-pyrido[3,4-b] indole) and STZ (N-(Methylnitrosocarbamoyl)-α-D-glucosamine) were bought from Sigma-Aldrich (USA).
3.2. Plant Collection and Preparation of Seed Extract of Peganum harmala
We collected P. harmala five kilometers outside Zahedan (Sistan and Baluchestan, Iran) in late spring. The Central Herbarium of Iran (TARI) confirmed P. harmala, registered with the number 107208. Also, we validated taxonomically on the website "http://www.ipni.org/."
We first crushed the plant sample to extract the methanolic seed extract of P. harmala. Then, 100 g of the sample was added to a thimble and placed in the middle of the Soxhlet extractor. Afterward, methanol (500 mL) was added to the as-collected samples in the Soxhlet at a rate of 20 siphons for four hours. Rotary evaporation separated the solvent from the extract, and it was stored at 4°C until subsequent use (22). The sample was analyzed with Fourier-transform Infrared Spectroscopy (FTIR) (WQF-510 FT-IR Spectrometer, China), High-performance Liquid Chromatography (HPLC), and a chromatographer (KNAUER, Germany) equipped with a C18 column. Phytochemical analysis results, lethal dose, spectroscopic data, and antioxidant properties of the extract are available in our previous paper (23).
3.3. Experimental Design
Forty-eight male Wistar rats (250 - 300 g) were kept in a 12/12 light cycle at a temperature of 22 ± 2°C, with a standard diet of pellet and water ad libitum. Then, they were randomly divided into six groups (n = 8): Control (C), diabetic (D), harmine (H), diabetic plus harmine (DH), seed extract (S), and diabetic plus seed extract (DS). A dose of 6.5 mg/kg body weight (BW) of Harmine (Sigma-Aldrich, USA) and 150 mg/kg BW of seed extract was gavaged to the treatment rats for 28 days (24, 25).
After the treatment period, all rats were anesthetized via ketamine-xylazine. Their brains underwent surgery, and the middle of their skulls was removed. The right and left hippocampus came apart by accomplishing the meninges via forceps. The hippocampus was utilized in histological research and the apoptosis assay. The Ethics Committee of the Islamic Azad University, Science and Research Branch, Tehran, Iran (ethical code: IR.IAU.SRB.REC.1396.39) confirmed all animal protocols. Animals were cared for following the guide to the care and use of experimental animals.
3.4. Diabetes Induction
Before inducing diabetes through STZ (60 mg/kg; Sigma-Aldrich, USA), liquefied in 3 mL of Milli-Q water, the rats were not fed for 12 h. The STZ dose was determined based on the body weight of the animals and injected intraperitoneally (26). Before the experiment began, each animal's weight was measured. The glucometer (Accuchek, Germany) was a tool to gather blood from the tail vein to examine blood glucose levels. The blood glucose level of fasting rats was evaluated every day until the end of the tests. Following 10 days, the rats' blood glucose was assessed 10 days repeatedly, showing a level of > 200 mg/dL, so they had diabetes. The study examined diabetic rats and rats with polydipsia and polyuria for the next 10 days.
3.5. Apoptosis Assay by Flow Cytometry
After diluting trypsin (0.25 g; Merck, Germany) in physiological serum (100 mL), the solvent of DNase (50 µL; Qiagen, Germany) consisted of trypsin (5 mL). The tissue of the hippocampus was rinsed with saline, which was buffered with phosphate to displace the outstanding blood and compressed immediately. Next, 100 μL of 0.25% trypsin and 0.5% DNase were added to the amount of compressed tissue in a microtube. Every 10 minutes, the microtube substance was steadily measured up and down by a pipet for 10 minutes and blended for 30 minutes. Pipetting assessment was performed at the temperature of 37°C for five minutes. Afterward, 5 µL of bovine serum was in the microtube to disable the enzyme. The content was centrifuged at 1500 rpm at 44°C for five minutes. Following throwing away the supernatant and adding PBS, the remnants were moved to a new microtube and reserved at -80°C. 100 µL of buffer, 10 µL of propidium iodide (PI), and 5 µL of annexin were added 10 minutes before flow cytometry examinations. Apoptosis was assessed (27). A flow cytometer (BD FACS Calibur, BD biosciences, San Jose, CA, USA) was utilized to analyze the samples.
3.6. Gene Expression of Bax and Bcl-2 Through Real-time Polymerase Chain Reaction
Before RT-PCR tests of genes, the hippocampus was cut into pieces, and then a small part was snap-frozen in liquid nitrogen. Total RNA was extracted from the hippocampal tissue of processed rats through the TriPure Isolation Reagent (Roche, Germany). Samples were kept at -80°C until analysis. A spectrophotometer was applied to identify the concentration and purity of mRNA samples. Only samples having an OD260/280 ratio of 1.8 to 2.0 were utilized. Reverse transcription (Thermo Scientific, USA) was employed to synthesize complementary DNA (cDNA) by a 20-liter apparatus. The denaturation of samples was done at 95°C for 10 minutes and magnified with 40 cycles of 93°C for 20 seconds, 59°C for 40 seconds, and 72°C for 20 seconds. Quantitative real-time polymerase chain reaction (RT-qPCR) was used to examine the relative expression of target genes through real Q-plus 2x Master mix Green with ROX (Amplicon, Denmark) and gene-specific primers. The primers for PCR are shown in Table 1. The abundance ratio of a target gene associated with Glyceraldehydes-3-phosphate dehydrogenase (GAPDH) (housekeeping gene) was applied to measure the relative gene expression. The ABI step one plus (ABI Step One, Applied Biosystems, Sequences Detection Systems, USA) instrument was used to conduct real-time PCR. The 2-ΔΔCt method (Applied Biosystems Research Bulletin No. 2 P/N 4303859) was used to calculate the data (6).
Identity and Sequence Details of PCR Primers Used to Analyze mRNA Expression
Gene | Primer (5'-3') | Length (bp) |
---|---|---|
Bax | 176 | |
Forward | TGCTACAGGGTTTCATCCAG | |
Reverse | TTGTTGTCCAGTTCATCGCC | |
Bcl-2 | 226 | |
Forward | ACTTCTCTCGTCGCTACCG | |
Reverse | ACCCCATCCCTGAAGAGTT | |
GAPDH | 68 | |
Forward | GGTGATGCTGGTGCTGAGTA | |
Reverse | GGAGATGATGACCCTTTTGGC |
3.7. Western Blotting to Discover Protein Expression
Proteins of each group were removed by dissolving RIPA and extraction buffer Kit, named Cat No: 89900, Thermo Scientific, located in the USA. The accumulation of proteins was recognized following the approach of Bradford through bovine serum albumin (BSA), known as a standard, at the wavelength of 630 nm (28). Electrophoresis of total proteins was done in 10% sodium dodecyl sulfate-polyacrylamide gel electrophoresis (SDS-PAGE) gel, transferred to nitrocellulose membranes. Following the transmembrane, the membrane was rinsed with TBST solution for 5 min. After closing by buffer (5% dried milk without fat in PBS) at ambient temperature for one h, the membrane was cultured with primary antibody (1:1,000) at ambient temperature overnight. The membrane was bathed three times via TBST (5 min each time) and then incubated with a secondary antibody (Biovision, USA) (1:1,000) at ambient temperature for 1 h. The membrane was rinsed three times via TBST (5 min each time). The membranes were expanded by raising with chemiluminescence reagents (Amersham Bioscience, USA). Protein bands located in the photographic film were assessed through densitometry scanning. The general densities of bands were evaluated by Image J software (29).
3.8. Haematoxylin & Eosin and Cresyl Violet Staining
The hippocampal tissues were kept in 10% paraformaldehyde for 24 hours before sectioning (hippocampal CA1 region). The slices (~3-5 µm thickness) were accumulated on glass slides and stained by common haematoxylin & eosin (H&E) staining. Afterward, a light microscope was applied to capture images.
The slides were submerged in xylene (Sigma, USA) twice for five minutes and then in 100% ethanol (Sigma, USA) twice for five minutes each time. The slides were placed in 95% ethanol and 70% ethanol for two minutes each time before being immersed in distilled water for two minutes. Then, the slides were immersed in cresyl violet staining solvent for 15 - 17 minutes before being rewashed in distilled water for two minutes. The slides were coverslipped with a permanent mounting medium and air-dried overnight (30).
3.9. Statistical Analysis
The data were analyzed by SPSS 20.0 (SPSS Inc., Chicago, USA) and presented as mean ± SD. Statistical analyses were done via ANOVA and Tukey's tests and GraphPad Prism 5 (Prism, GraphPad Software, San Diego, CA) at P < 0.05 as a statistically significant level.
4. Results
4.1. Body Weights and Glucose Levels
Initially, all groups had the same body weights. Bodyweight was enhanced in the control group after four weeks, whereas the diabetic groups significantly lost their body weight (P = 0.01) (Table 2).
Parameters | Experimental Groups | |||||
---|---|---|---|---|---|---|
C | D | DH | H | DS | S | |
Initial body weight (g) | 251 ± 12.1 | 254 ± 14.1 | 255 ± 14.2 | 257 ± 10.9 | 253 ± 13.2 | 254 ± 11.3 |
Final body weight (g) | 315 ± 11 | 205 ± 10.1 b | 299 ± 14.8 | 318 ± 11 | 296 ± 13.9 | 295 ± 10.5 |
The blood glucose level of the rats was investigated through a glucometer on days 10, 14, and 28 (Table 3). In the D group, glucose levels increased significantly compared to the other groups (P = 0.001). Furthermore, the blood glucose levels of healthy rats were not significantly different (C, S, and H groups). However, a significant decrease was observed in diabetic rats treated with seed extract (DS) and harmine (DH) (P = 0.01).
4.2. Apoptotic Experiments
Single cells were analyzed by flow cytometry to investigate the effect of treatments on hippocampal cell apoptosis (Figure 1A). Compared to the C group, STZ-induced apoptosis led to tissue destruction and damage in the rats' hippocampal region of the D group (P = 0.001). Furthermore, apoptosis was significantly reduced in the DS and DH groups (P = 0.01). The two groups had no statistically significant differences (Figure 1B).
Flow cytometry examination of annexin V, propidium iodide (PI) staining of hippocampal tissue (A); and apoptosis rate in the experimental groups (B). The necrotic cells, FITC-annexin V-negative, PI-positive, are illustrated in the upper left quadrants of each panel. The viable cells, FITC-Annexin V-negative, PI-negative, are shown in the lower left quadrants of each panel. The upper right quadrants consist of the late apoptotic cells, positive for FITC-Annexin V binding, PI uptake. The lower right quadrants show the early apoptotic cells, FITCAnnexin V-positive, PI-negative. a, b, c, d, values with various superscripts within a column vary significantly. Abbreviations: C, control rats; D, diabetes rats; S, seed extract-treated rats; DH, harmine-treated diabetic rats; H, harmine-treated rats; DS, seed extract-treated diabetic rats.
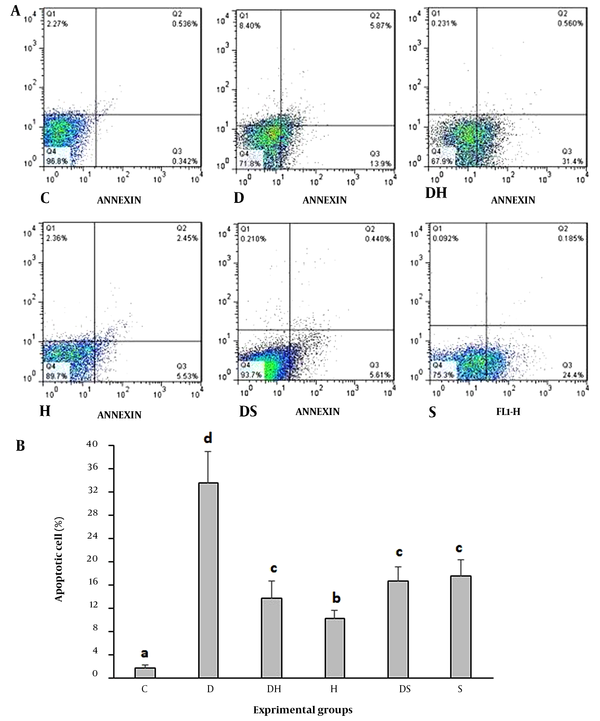
4.3. Expression Levels of Bax and Bcl-2 Genes and Proteins
Bax mRNA expression significantly increased in the D, DH, and DS groups compared to the control group (P = 0.01). In the DH and DS groups, Bax mRNA expression was significantly reduced compared to the diabetic group (Figure 2A). The Bcl-2 mRNA expression level significantly decreased in D, DH, and DS groups compared to the control group (P = 0.01). The Bcl-2 mRNA expression significantly increased in the DH and DS groups compared to the diabetic group (Figure 2B). Besides, the expression ratio of BAX to Bcl-2 was significantly increased in the diabetic group compared to the control, DH, and DS groups (P = 0.001) (Figure 2C). Western blot results indicated that Bax protein expression level was significantly lower in the DH (1.163 ± 0.06) and DS groups (1.113 ± 0.07) than in the diabetic (D) group (1.582 ± 0.08) (P = 0.01) (Figure 2D, E). The level of Bcl-2 protein expression in the D group (0.527 ± 0.03) had a significant reduction compared to the control (0.961 ± 0.057), DH (0.739 ± 0.046), and DS groups (0.992 ± 0.057) (P = 0.01) (Figure 2D, E).
Expression levels of genes and proteins of Bax and Bcl-2 in the experimental groups. Findings of reverse transcriptase real-time polymerase chain reaction (PCR) for mRNAs of Bax (A); Bcl-2 (B); and Bax/Bcl-2 ratio (C) (** P < 0.01, *** P < 0.001). Immunoblots of Bax and Bcl-2 (D); from hippocampal cell lysates. Densities of Bax and Bcl-2 protein bands in the experimental groups are presented (E). The bar graph shows the mean ± SD (one-way ANOVA and Tukey's test, P < 0.05) (* P < 0.01, # P < 0.01). Abbreviations: C, control rats; D, diabetes rats; S, seed extract-treated rats; DH, harmine-treated diabetic rats; H, harmine-treated rats; DS, seed extract-treated diabetic rats; NS, non-significant.
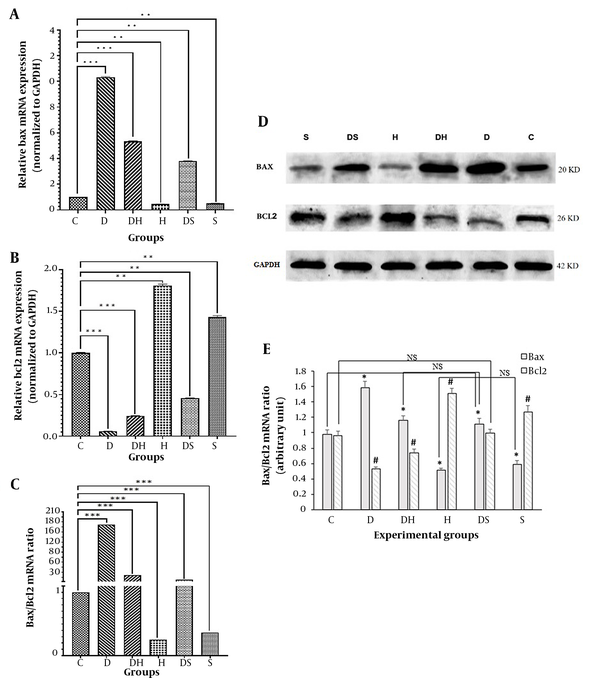
4.4. Histopathological Evaluation
Histopathological results of H&E (Figure 3A) and cresyl violet (Figure 3B) staining indicated that in the C group, the cells had a lower density in the cornu ammonis (CA1) region than in other regions. Also, the nuclei of cells were healthy, and their size and number were normally visible. However, in the D group, there were many heterochromatin nuclei and cell necrosis in the dentate gyrus (DG) region. In addition, the destruction of support cells and their related empty space was observed in some parts of CA1. In the experimental group treated with H, more cell density and order and less destruction were seen in the CA1 region. The thickness of the DG region was normal, and its granular layer was composed of healthy and regular cells. In harmine (DH)-treated diabetic rats, the cell density in the DG region was low in the periphery; nonetheless, in some heterochromatin cases, the cell density was low in the CA1 region but with minimal necrosis. In the S treated-experimental group, the cell density was low in the CA1 region, and some cells were damaged, though they were histologically healthy. The density of cells was high in the CA3 region, with heterochromatin cells in some parts. The destruction of support and heterochromatin cells was found in the DG region. In some parts, necrosis was observed in the support cells; however, tissue damage was much less than in the D group. The thicknesses of different areas of the hippocampus are compared in Figure 3C.
Histopathological evaluation of hippocampal tissue: A, H&E staining; B, cresyl violet staining; and C, average thickness of various areas of the hippocampus, dentate gyrus (DG), and CA1 (magnification 40x). Abbreviations: C, control rats; D, diabetes rats; S, seed extract-treated rats; DH, harmine-treated diabetic rats; H, harmine-treated rats; DS, seed extract-treated diabetic rats.
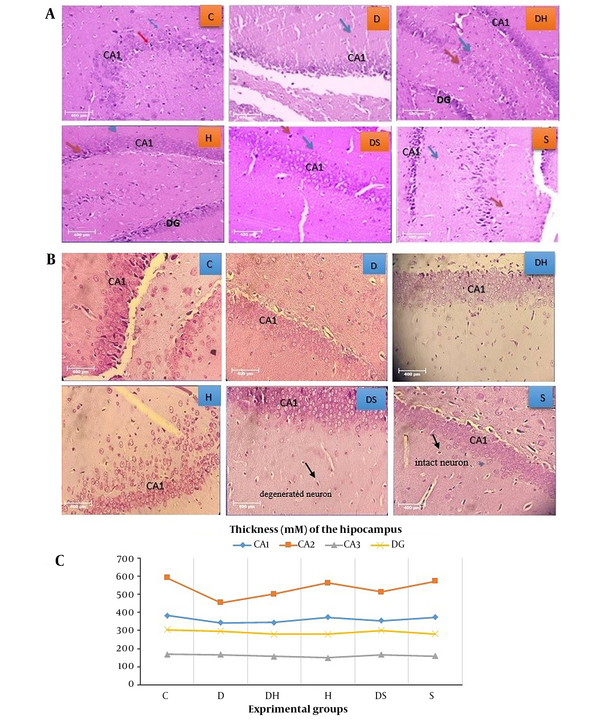
5. Discussion
The hippocampus is the most vulnerable part of the central nervous system (31). Streptozotocin-induced apoptosis in the hippocampal cells of D rats was significantly higher than that of the C one. This finding was similar to that of Wang et al., suggesting neurological complications of diabetes due to the increased oxidative stress caused by hyperglycemia (32). Our phytochemical analysis of the seed extract and its active ingredient, harmine, revealed that β-carboline possibly plays a role in preventing STZ-induced apoptosis in the DS group. In groups treated with both the seed extract and harmine, apoptosis increased compared to the control, suggesting that treating rats through these compounds results in side effects such as apoptosis and tissue destruction. Therefore, although the selected dose for inhibiting STZ-induced apoptosis seemed appropriate, it may associate with some complications in healthy rats.
Studies have shown that harmine has neuroprotective effects on the reactive oxygen species (ROS) due to its scavenging activity (33, 34). A previous survey has presented that using P. harmala seed extract for mice reduces memory impairments caused by sodium nitrate and ethanol and can reduce DNA fragmentation of the prefrontal cortex. Moreover, it diminishes the formation of thiobarbituric acid reactive substances (TBARS) in the brainby inhibiting acetylcholinesterase and consequently enhancing glutathione levels (35). As a result, our research supports P. harmala's role by demonstrating pharmacological apoptosis inhibition in diabetics subjects, which is consistent with other studies (19, 20). Bax gene expression in the D group, including rats with diabetes, was highly significant. The Bax gene expression in diabetes-induced apoptosis has previously been confirmed (6). In this regard, He et al. suggested that in the diabetic group, apoptosis of the hippocampal neurons is associated with subarachnoid bleeding (6). They confirmed that the induction of diabetes is related to the enhanced Bax/Bcl-2, enhanced Bax/Bcl-xL ratio, and caspase 3 activity in the hippocampus, which it is due to apoptosis in the hippocampal region (26).
The findings indicated that mRNA and protein levels of Bax expression significantly increased in the D group after four weeks, including the diabetic hippocampus, whereas the Bcl-2 expression was significantly decreased. This was consistent with previous studies (6, 26). Furthermore, Bax/Bcl-2 ratio had a significant increase in the D group, which indicates that apoptosis in the hippocampus of diabetic rats induced by STZ interfered with the mitochondrial pathway (36).
For combating insulin resistance, P. harmala is novel machinery in attenuating the insidious progression of Alzheimer's disease by enhancing both insulin and glucagon-like peptide 1 (GLP-1) trajectories in the hippocampus favoring glucose transporter type 4 (GLUT4) production (37). These results are in good agreement with our outcomes. Similar to our results, Das et al. found that flavonoids can be the remedial agents to induce apoptosis in glioblastoma cells (38). In the current study, the tissue status was improved in the diabetic groups treated with the extraction of seed and harmine, although the rate of improvement was higher in the DS group. Such improvement is expected concerning the antioxidant properties of the seed extract. It has also been indicated that the histopathology of melatonin antioxidants is changed for apoptosis and oxidative stress in the brain of rats with diabetes (39). Harmaline alkaloids improve pathological changes in rats' neurons in the CA1 region of the hippocampus and amend learning and memory ability in vascular destruction of the rats, which is likely linked with the inhibition of apoptosis in hippocampal cells (40). It has also been indicated that the expression of other proteins existent in pathways related to apoptosis, namely FAS, Gadd45a, and Hspb1, can be downregulated by β-carboline, and the expression of inflammation-related genes (Cxcl9, Irf1, Fasl, Icam1, Tnf, and Vcam1) related to apoptosis can be lessened by β-carboline (41).
The results showed that the methanolic seed extract of P. harmala could reduce diabetic rats' glucose levels. The effect of the methanolic seed extract of P. harmala has been established in previous research on rats' blood glucose (42). Based on Komeili et al., P. harmala seeds may exert antidiabetic effects due to their antioxidant properties or via enzymatic inhibition or inducing the agonist/antagonistic effects on responsible receptors (43). They also suggested that the hypoglycemia effect of the hydroalcoholic seed extract of P. harmala may be due to the elevated insulin secretion by the remaining pancreatic beta cells in diabetic rats. Therefore, hypoglycemic activity of P. harmala can be attributed to glucose usage or its absorption rather than the pancreas (44).
Overall, as the P. harmala seed extract is rich in nitrogenous compounds, including alkaloids, in some cases, it may increase apoptosis. In other instances, it may significantly be changed the effects of combounds. In fact, these results depend on analyzing nitrogenous compounds interacting with alkaloids in the seeds. According to the significant reduction of apoptosis in the hippocampal region of the seed extract-treated rats compared with the diabetes group, it can be investigated in the future to disclose if this effect is related to the phenolic or β-carboline alkaloids and determine its intracellular mechanisms.
As a limitation, the study only used a single dose of P. harmala seed extract and harmine. Also, it did not assess other gene pathways of apoptosis, possibly affecting the results. Hence, future investigations may examine the expression of other relevant genes to determine the intracellular or extracellular pathways of apoptosis in the hippocampal tissue of diabetic rats.
5.1. Conclusions
The effects of the P. harmala seed extract and its active ingredient (harmine) on rat hippocampal tissue were compared, demonstrating that the seed extract had anti-apoptotic properties by downregulating Bax/Bcl-2 gene expression. However, more research is required to fully elucidate the intracellular signaling cascade of this pathway.
Acknowledgements
References
-
1.
Domon A, Katayama K, Tochigi Y, Suzuki H. Characterization of Novel Nonobese Type 2 Diabetes Rat Model with Enlarged Kidneys. J Diabetes Res. 2019;2019:8153140. [PubMed ID: 31467929]. [PubMed Central ID: PMC6701368]. https://doi.org/10.1155/2019/8153140.
-
2.
Sima AA, Kamiya H, Li ZG. Insulin, C-peptide, hyperglycemia, and central nervous system complications in diabetes. Eur J Pharmacol. 2004;490(1-3):187-97. [PubMed ID: 15094085]. https://doi.org/10.1016/j.ejphar.2004.02.056.
-
3.
Malone JI. Diabetic Central Neuropathy: CNS Damage Related to Hyperglycemia. Diabetes. 2016;65(2):355-7. [PubMed ID: 26798123]. https://doi.org/10.2337/dbi15-0034.
-
4.
Northam EA, Rankins D, Lin A, Wellard RM, Pell GS, Finch SJ, et al. Central nervous system function in youth with type 1 diabetes 12 years after disease onset. Diabetes Care. 2009;32(3):445-50. [PubMed ID: 19151204]. [PubMed Central ID: PMC2646026]. https://doi.org/10.2337/dc08-1657.
-
5.
Vafaei-Nezhad S, Vafaei-Nezhad M, Shadi M, Ezi S. The Impact of Diabetes on Hippocampus. In: Zhang X, editor. Hippocampus - Cytoarchitecture and Diseases. London: IntechOpen; 2021. https://doi.org/10.5772/intechopen.99895.
-
6.
He X, Sun J, Huang X. Expression of caspase-3, Bax and Bcl-2 in hippocampus of rats with diabetes and subarachnoid hemorrhage. Exp Ther Med. 2017;15(1):873-7. https://doi.org/10.3892/etm.2017.5438.
-
7.
Ghafari S, Asadi E, Shabani R, Golalipour MJ. Hippocampal Neuronal Apoptosis in Rat Offspring Due to Gestational Diabetes. Int J Morphol. 2016;34(1):205-11. https://doi.org/10.4067/s0717-95022016000100029.
-
8.
Guo C, Quobatari A, Shangguan Y, Hong S, Wiley JW, Quobatari A. Diabetic autonomic neuropathy: evidence for apoptosis in situ in the rat. Neurogastroenterol Motil. 2004;16(3):335-45. [PubMed ID: 15198656]. https://doi.org/10.1111/j.1365-2982.2004.00524.x.
-
9.
Waldmeier PC, Tatton WG. Interrupting apoptosis in neurodegenerative disease: potential for effective therapy? Drug Discov Today. 2004;9(5):210-8. [PubMed ID: 14980539]. https://doi.org/10.1016/S1359-6446(03)03000-9.
-
10.
Haghir H, Hami J, Lotfi N, Peyvandi M, Ghasemi S, Hosseini M. Expression of apoptosis-regulatory genes in the hippocampus of rat neonates born to mothers with diabetes. Metab Brain Dis. 2017;32(2):617-28. [PubMed ID: 28078553]. https://doi.org/10.1007/s11011-017-9950-2.
-
11.
Jafari Anarkooli I, Sankian M, Ahmadpour S, Haghir H, Bonakdaran S, Varasteh A. [The Study of Effects of Insulin and Ascorbic Acid on Bcl-2 Family Expression in Hippocampus of Streptozotocin -Induced Diabetic Rats]. J Adv Med Biomed Res. 2007;15(60):1-16. Persian.
-
12.
Zhou W, Yao Y, Li J, Wu D, Zhao M, Yan Z, et al. TIGAR Attenuates High Glucose-Induced Neuronal Apoptosis via an Autophagy Pathway. Front Mol Neurosci. 2019;12:193. [PubMed ID: 31456661]. [PubMed Central ID: PMC6700368]. https://doi.org/10.3389/fnmol.2019.00193.
-
13.
Jafari Anarkooli I, Sankian M, Ahmadpour S, Varasteh AR, Haghir H. Evaluation of Bcl-2 family gene expression and Caspase-3 activity in hippocampus STZ-induced diabetic rats. Exp Diabetes Res. 2008;2008:638467. [PubMed ID: 18923682]. [PubMed Central ID: PMC2566751]. https://doi.org/10.1155/2008/638467.
-
14.
Giribabu N, Srinivasarao N, Swapna Rekha S, Muniandy S, Salleh N. Centella asiatica Attenuates Diabetes Induced Hippocampal Changes in Experimental Diabetic Rats. Evid Based Complement Alternat Med. 2014;2014:592062. [PubMed ID: 25161691]. [PubMed Central ID: PMC4139016]. https://doi.org/10.1155/2014/592062.
-
15.
Hayet E, Maha M, Mata M, Mighri Z, Gutman L, Mahjoub A. Biological activities of Peganum harmala leaves. Afr J Biotechnol. 2010;9(48):8199-205. https://doi.org/10.5897/ajb10.564.
-
16.
Moloudizargari M, Mikaili P, Aghajanshakeri S, Asghari MH, Shayegh J. Pharmacological and therapeutic effects of Peganum harmala and its main alkaloids. Pharmacogn Rev. 2013;7(14):199-212. [PubMed ID: 24347928]. [PubMed Central ID: PMC3841998]. https://doi.org/10.4103/0973-7847.120524.
-
17.
Salahshoor MR, Roshankhah S, Motavalian V, Jalili C. Effect of Harmine on Nicotine-Induced Kidney Dysfunction in Male Mice. Int J Prev Med. 2019;10:97. [PubMed ID: 31360344]. [PubMed Central ID: PMC6592107]. https://doi.org/10.4103/ijpvm.IJPVM_85_18.
-
18.
Nafisi S, Asghari MH, Mohammad Nezhady MA, Ekhtiari MS. Possible antidiabetic effect of Peganum harmala on streptozocine-induced mouse. World Appl Sci J. 2011;14(6):822-4.
-
19.
Kajbaf F, Oryan S, Ahmadi R, Eidi A. Assessment of the Anti-apoptotic Effects of Peganum harmala Leaf Extract on Type 2 Diabetes in the Kidney of Male Wistar Rats. Avicenna J Med Biochem. 2020;8(2):74-82. https://doi.org/10.34172/ajmb.2020.11.
-
20.
Safamanesh A, Oryan S, Ahmadi R, Parivar K. Inhibition of Streptozotocin-Induced Apoptosis in Beta Cells by Peganum harmala Seed Extracts in Adult Male Rats. Crescent J Med Biol Sci. 2021;8(4):296-302.
-
21.
Magdy N, Salama M, Alsaedy Y, El-Sayed G. Ameliorative effects of Pergamum harmala seed extract on obese diabetic rats. Mansoura Vet Med J. 2020;21(3):116-20. https://doi.org/10.21608/mvmj.2020.21.319.
-
22.
Salehi H, Karimi M, Rezaie N, Raofie F. Extraction of β-Carboline alkaloids and preparation of extract nanoparticles from Peganum harmala L. capsules using supercritical fluid technique. J Drug Deliv Sci Technol. 2020;56:101515. https://doi.org/10.1016/j.jddst.2020.101515.
-
23.
Fahimi S, Oryan S, Ahmadi R, Eidi A. Evaluation of chemical components, anti-oxidant properties, and lethal toxicity of alkaloids extracted from espand (Peganum harmala). J Appl Biol Sci. 2022;16(2):257-65.
-
24.
Rezzagui A, Merghem M, Derafa I, Dahamna S. Acute and Sub-acute Toxic Effects of Algerian Peganum harmala L. Crud Extract. J Drug Deliv Ther. 2020;10(2):115-21. https://doi.org/10.22270/jddt.v10i2.3920.
-
25.
Fortunato JJ, Reus GZ, Kirsch TR, Stringari RB, Stertz L, Kapczinski F, et al. Acute harmine administration induces antidepressive-like effects and increases BDNF levels in the rat hippocampus. Prog Neuropsychopharmacol Biol Psychiatry. 2009;33(8):1425-30. [PubMed ID: 19632287]. https://doi.org/10.1016/j.pnpbp.2009.07.021.
-
26.
Jafari Anarkooli I, Barzegar Ganji H, Pourheidar M. The protective effects of insulin and natural honey against hippocampal cell death in streptozotocin-induced diabetic rats. J Diabetes Res. 2014;2014:491571. [PubMed ID: 24745031]. [PubMed Central ID: PMC3976855]. https://doi.org/10.1155/2014/491571.
-
27.
Naseer MI, Ullah I, Narasimhan ML, Lee HY, Bressan RA, Yoon GH, et al. Neuroprotective effect of osmotin against ethanol-induced apoptotic neurodegeneration in the developing rat brain. Cell Death Dis. 2014;5(3). e1150. [PubMed ID: 24675468]. [PubMed Central ID: PMC3973231]. https://doi.org/10.1038/cddis.2014.53.
-
28.
Bradford MM. A rapid and sensitive method for the quantitation of microgram quantities of protein utilizing the principle of protein-dye binding. Anal Biochem. 1976;72:248-54. [PubMed ID: 942051]. https://doi.org/10.1006/abio.1976.9999.
-
29.
Cheraghi E, Soleimani Mehranjani M, Shariatzadeh SMA, Nasr Esfahani MH, Alani B. N-Acetylcysteine Compared to Metformin, Improves The Expression Profile of Growth Differentiation Factor-9 and Receptor Tyrosine Kinase c-Kit in The Oocytes of Patients with Polycystic Ovarian Syndrome. Int J Fertil Steril. 2018;11(4):270-8. [PubMed ID: 29043702]. [PubMed Central ID: PMC5641458]. https://doi.org/10.22074/ijfs.2018.5142.
-
30.
Mohd Shafri MA, Mat Jais AM, Md Jaffri J, Kim MK, Ithnin H, Mohamed F. Cresyl violet staining to assess neuroprotective and euroregenerative effects of Haruan traditional extract against eurodegenerative damage of ketamine. Int J Pharm Pharm Sci. 2012;4(4):163-8.
-
31.
Aragno M, Mastrocola R, Brignardello E, Catalano M, Robino G, Manti R, et al. Dehydroepiandrosterone modulates nuclear factor-kappaB activation in hippocampus of diabetic rats. Endocrinology. 2002;143(9):3250-8. [PubMed ID: 12193536]. https://doi.org/10.1210/en.2002-220182.
-
32.
Wang Q, Pfister F, Dorn-Beineke A, vom Hagen F, Lin J, Feng Y, et al. Low-dose erythropoietin inhibits oxidative stress and early vascular changes in the experimental diabetic retina. Diabetologia. 2010;53(6):1227-38. [PubMed ID: 20339831]. https://doi.org/10.1007/s00125-010-1727-7.
-
33.
Kim DH, Jang YY, Han ES, Lee CS. Protective effect of harmaline and harmalol against dopamine- and 6-hydroxydopamine-induced oxidative damage of brain mitochondria and synaptosomes, and viability loss of PC12 cells. Eur J Neurosci. 2001;13(10):1861-72. [PubMed ID: 11403679]. https://doi.org/10.1046/j.0953-816x.2001.01563.x.
-
34.
Lee CS, Han ES, Jang YY, Han JH, Ha HW, Kim DE. Protective effect of harmalol and harmaline on MPTP neurotoxicity in the mouse and dopamine-induced damage of brain mitochondria and PC12 cells. J Neurochem. 2000;75(2):521-31. [PubMed ID: 10899927]. https://doi.org/10.1046/j.1471-4159.2000.0750521.x.
-
35.
Biradar SM, Joshi H, Tarak KC. Cerebroprotective effect of isolated harmine alkaloids extracts of seeds of Peganum harmala L. on sodium nitrite-induced hypoxia and ethanol-induced neurodegeneration in young mice. Pak J Biol Sci. 2013;16(23):1687-97. [PubMed ID: 24506035]. https://doi.org/10.3923/pjbs.2013.1687.1697.
-
36.
Duchen MR. Mitochondria in health and disease: perspectives on a new mitochondrial biology. Mol Aspects Med. 2004;25(4):365-451. [PubMed ID: 15302203]. https://doi.org/10.1016/j.mam.2004.03.001.
-
37.
Saleh RA, Eissa TF, Abdallah DM, Saad MA, El-Abhar HS. Peganum harmala enhanced GLP-1 and restored insulin signaling to alleviate AlCl3-induced Alzheimer-like pathology model. Sci Rep. 2021;11(1):12040. [PubMed ID: 34103557]. [PubMed Central ID: PMC8187628]. https://doi.org/10.1038/s41598-021-90545-4.
-
38.
Das A, Banik NL, Ray SK. Flavonoids activated caspases for apoptosis in human glioblastoma T98G and U87MG cells but not in human normal astrocytes. Cancer. 2010;116(1):164-76. [PubMed ID: 19894226]. [PubMed Central ID: PMC3159962]. https://doi.org/10.1002/cncr.24699.
-
39.
Gurpinar T, Ekerbicer N, Uysal N, Barut T, Tarakci F, Tuglu MI. The effects of the melatonin treatment on the oxidative stress and apoptosis in diabetic eye and brain. ScientificWorldJournal. 2012;2012:498489. [PubMed ID: 22654617]. [PubMed Central ID: PMC3361279]. https://doi.org/10.1100/2012/498489.
-
40.
Zhang XS, Sun JN, Yu HL. [Effects of Total Alkaloids of Harmaline on Learning and Memory in Vascular Dementia Rats]. Zhong Yao Cai. 2015;38(11):2353-7. Chinese. [PubMed ID: 27356391].
-
41.
Hamann J, Wernicke C, Lehmann J, Reichmann H, Rommelspacher H, Gille G. 9-Methyl-beta-carboline up-regulates the appearance of differentiated dopaminergic neurones in primary mesencephalic culture. Neurochem Int. 2008;52(4-5):688-700. [PubMed ID: 17913302]. https://doi.org/10.1016/j.neuint.2007.08.018.
-
42.
Singh AB, Chaturvedi JP, Narender T, Srivastava AK. Preliminary studies on the hypoglycemic effect of Peganum harmala L. Seeds ethanol extract on normal and streptozotocin induced diabetic rats. Indian J Clin Biochem. 2008;23(4):391-3. [PubMed ID: 23105794]. [PubMed Central ID: PMC3453127]. https://doi.org/10.1007/s12291-008-0086-3.
-
43.
Komeili G, Hashemi M, Bameri-Niafar M. Evaluation of Antidiabetic and Antihyperlipidemic Effects of Peganum harmala Seeds in Diabetic Rats. Cholesterol. 2016;2016:7389864. [PubMed ID: 27190643]. [PubMed Central ID: PMC4848417]. https://doi.org/10.1155/2016/7389864.
-
44.
Ae Park S, Choi MS, Cho SY, Seo JS, Jung UJ, Kim MJ, et al. Genistein and daidzein modulate hepatic glucose and lipid regulating enzyme activities in C57BL/KsJ-db/db mice. Life Sci. 2006;79(12):1207-13. [PubMed ID: 16647724]. https://doi.org/10.1016/j.lfs.2006.03.022.