Abstract
Background:
Obsessive-compulsive disorder (OCD) is considered neurodevelopmental disorder that shares the feature of dysfunctional frontal-subcortical circuitry, yet the relationship between structural abnormalities or neurobiochemistrical changes and OCD pathogenesis is still unknown.Objectives:
This study aims to use magnetic resonance spectroscopy (MRS) to discover substance-metabolism abnormalities of brain circuits in patients with first-episode OCD, and to study the mechanism of OCD.Patients and Methods:
Twenty patients with first-episode OCD and twenty gender- and age-matched normal controls were studied. Neurochemical abnormalities, including ratios of N-acetylaspartate (NAA), creatine (Cr), and choline (Cho), were measured in the prefrontal cortex, anterior cingulate cortex, orbitofrontal cortex, caudate nucleus, and thalamus with three-dimensional 1H-proton MRS (3D 1H-MRS). The data of the ratios in the two groups were analyzed with a independent samples t-test.Results:
Cho/Cr ratios in the thalamus were significantly higher in the first-episode OCD patients than in the controls (P < 0.01), and the NAA/Cr ratios in the caudate nucleus and thalamus were significantly lower in the first-episode OCD patients than in the controls (P < 0.01). However, NAA/Cr ratios in the anterior cingulate cortex and orbitofrontal cortex were higher in the first-episode OCD patients than in the controls (P < 0.01).Conclusion:
Distinct neurochemical and histological structure changes (cortico-striatal-thalamic-cortical cycle) exist in patients with first-episode OCD. 1H-MRS is capable of identifying these changes by assessing metabolic abnormalities.Keywords
Obsessive-Compulsive Disorder Brain Magnetic Resonance Spectroscopy
1. Background
Obsessive-compulsive disorder (OCD) comprises a group of neuroses with mainly clinical symptoms of obsession (including obsessive rumination and compulsion). It is a mental disease with 2% - 3% lifetime prevalence and increased rates of disability (1). OCD is a prevalent and clinically significant phenomenon in schizophrenia patients (2), but its etiopathogenesis and pathogenesis are not clear (3). Since both schizophrenia and OCD are considered neurodevelopmental disorders that share the feature of dysfunctional frontal-subcortical circuitry (4), dysfunction of the neuroanatomical circuit in OCD patients has been suggested (5). This circuit consists mainly of the prefrontal lobe, basal ganglia, limbic system, and thalamus (6-9), and the relationship between structural abnormalities or neurobiochemical changes and OCD pathogenesis is still unknown. As the only noninvasive molecular imaging method of detecting neurobiochemical information about living tissues, magnetic resonance spectroscopy (MRS) provides a new means of studying human mental disorders. Volumetric magnetic resonance imaging (MRI) images have shown that OCD patients have increased white matter volume, significantly smaller left and right orbitofrontal cortex volumes, and significantly greater left and right thalamus volumes (10).
It is possible to detect possible neurobiochemical disorders according to the characteristics of substance metabolism in patients with OCD, by using three-dimensional 1-hydrogen MRS (1H-MRS). This technique provides clues about the neurobiological basis of OCD. MR images are obtained from the magnetization of vector signals produced by magnetic nuclei with positive-charge resonance transitions, which are used to identify metabolic abnormalities (11).
In the brain, all of the metabolic compounds that can produce MRS signals are associated with the Krebs cycle and/or energy metabolism, such as creatine (Cr)/phosphocreatine, which is involved in ADP and ATP conversion. A decrease of Cr leads to a lack of energy in glial cells; glutamine (Glx) and N-acetyl aspartate (NAA) are involved in the mitochondrial oxidation-reduction reaction, and Glx is helpful for the recognition of meningioma because it is always increased in that condition. Glu and the signal intensity of NAA reflects the number and functional status of neurons; the cytoplasmic redox lactic acid (Lac) reaction process, choline (Cho) compounds, and myo-inositol (MI) are involved in the redox reaction of the cytoplasm. Lac indicates the metabolism status, Cho has always been considered a brain tumor biomarker, and MI is a glial cell marker. The molecular movements of all of the above compounds produce signals that can be detected by MRS (12).
2. Objectives
This study aims to use IH-MRS to discover substance-metabolism abnormalities of brain circuits in patients with first-episode OCD, and to study the mechanisms of OCD.
3. Patients and Methods
3.1. Subjects
The OCD group comprised patients with first-episode OCD, who presented to the psychological counseling clinic of Hainan Provincial People’s Hospital from January 2010 to December 2012. The inclusion criteria were as follows: 1) age 18 - 60 years; 2) compliance with Chinese classification of mental disorders-III (CCMD-III) and diagnostic and statistical manual of mental disorders-IV (DSM-IV) definitions of OCD; 3) first-episode OCD and no antidepressants or other psychiatric drugs; 4) no structural damage shown on cranial MRI; 5) no other systematic diseases or any family history of mental illness; 6) a score of more than 20 on the Yale-brown obsessive compulsive scale; and 7) right-handedness. Exactly 20 cases met these criteria, including 13 males and 7 females with an age range of 15 - 45 years (28.8 ± 8.3). The length of the patients’ diseases ranged from 0.6 to 8 years (3.66 ± 4.05), education level ranged from 8 to 15 years (10.9 ± 2.2 average), and scores for the Yale-brown obsessive compulsive scale were 25 - 38 (19.2 ± 3.8).
This study was conducted in accordance with the declaration of Helsinki and with approval from the ethics committee of Hainan provincial people’s hospital. Written informed consent was obtained from all participants.
The control group comprised volunteers who underwent health examinations during the same period. The inclusion criteria for this group were as follows: 1) matched to the OCD group for age, sex, and education level; 2) no critical diseases of the heart, liver, lung, kidney, or other organs after comprehensive physical and laboratory examination, and no metabolic diseases such as diabetes or hyperthyroidism; 3) no personal or family history of mental illness, including in first-degree relatives; 4) no structural damage on cranial MRI; and 5) right-handedness. Exactly 20 cases met these criteria, including 12 males and 8 females with an age range of 15 - 46 years (29.2 ± 8.6) and an education level of 8 - 16 years (11.2 ± 2.4).
3.2. Diagnosis and Scale Rating
The patients with OCD were diagnosed by trained attending or higher-level psychiatrists, and the scores for the Yale–Brown obsessive compulsive scale were assessed by psychiatrists.
3.3. Data Collection
The radiologist was not informed of the clinical data of the subjects. All MRS examinations were performed using a 1.5 Tesla scanner with an eight-channel head coil (GE Sigma 1.5 T Twin speed with Excite II, USA). Routine head MRI scans were performed on all subjects using the eight-channel head array coil, including the axial fluid-attenuated inversion recovery (FLAIR) sequence of T1 weighted Imaging (T1WI), the axial sagittal fast-recovery fast spin-echo (FRFSE) sequence, and the FLAIR sequence of T2WI, in order to exclude unknown diseases in the brain and to localize regions for 1H-MRS. Since multi-voxel spectroscopy was obtained during the test, a long time of echo (TE) was determined as 144 ms. The sampling point of 1H-MRS is zygomorphic, measuring about 40 cm3, and the bilateral prefrontal cortices, anterior cingulate cortex, orbitofrontal cortex, head of the caudate nucleus, and thalamus on the conventional T2-weighted images were selected as regions of interest (ROI) with the special head coil, after consultation with two trained attending radiologists. No difference in procedure was observed for the two groups. The scan time was 13 minutes and 24 seconds, and the measuring voxels were 1 cm3. Spectra were obtained with the point-resolved spectroscopy sequence (PRESS). The imaging parameters were 10 emission frequency (Freq), 10 (Phase), 10 mm depth, 10 mm interval, 16 field of view (FOV), 1000 ms/144 ms time of repetition (TR)/TE, and 98.6% average water-suppression rate.
3.4. Data Analysis
The software programs used were FuncTool 2 (GE Company, USA) and ACD 4.0 (SUN Company, USA). The ratios of Cho/Cr and NAA/Cr were determined in the ROIs of the bilateral prefrontal cortices, anterior cingulate cortex, orbitofrontal cortex, head of the caudate nucleus, and thalamus.
3.5. Statistical analysis
Statistical analysis were performed using SPSS for Windows ver. 11.5 (SPSS inc., Chicago, Il, USA). The ratios of Cho/Cr and NAA/Cr were analyzed with two-independent-sample t-tests, and P < 0.05 was considered statistically significant.
4. Results
No significant statistical differences were observed between the two groups in terms of age (t = -0.119, P = 0.897), gender (χ2 = 0.131, P = 0.717), and education level (t =- 0.216, P=0.831).
As the ratios of Cho/Cr and NAA/Cr in the ROIs had no significant differences between the left and right sides, they were combined with the statistical analysis. The Cho/Cr ratios in the thalamus (1.37 ± 0.11) in the OCD group were significantly higher than in the control group (1.17 ± 0.09) (P < 0.01). The Cho/Cr ratios in the prefrontal cortices, anterior cingulate cortex, orbitofrontal cortex, and head of the caudate nucleus showed no statistical differences between the two groups (P > 0.05) (Table 1). The NAA/Cr ratios in the head of the caudate nucleus and thalamus (1.22 ± 0.15 and 1.22 ± 0.11, respectively) in the OCD group were significantly lower than in the control group (1.47 ± 0.17 and 1.35 ± 0.11, respectively) (P < 0.01). The NAA/Cr ratios of the anterior cingulate and orbitofrontal cortices (2.92 ± 0.15 and 2.78 ± 0.23, respectively) in the OCD group were significantly higher than in the control group (2.76 ± 0.21 and 2.57 ± 0.18, respectively) (P < 0.01), but no significant differences were observed in the prefrontal cortices between the two groups (P > 0.05) (Table 2, Figures 1 and 2).
Group | N | Prefrontal Cortices | Anterior Cingulate Cortex | Orbitofrontal Cortex | Head of Caudate Nucleus | Thalamus |
---|---|---|---|---|---|---|
OCD | 18 | 2.25 ± 0.12 | 2.19 ± 0.13 | 2.22 ± 0.39 | 1.13 ± 0.14 | 1.37 ± 0.11 |
Control | 18 | 2.29 ± 0.09 | 2.18 ± 0.10 | 1.92 ± 0.16 | 1.12 ± 0.16 | 1.17 ± 0.09 |
t statistics | -1.105 | 0.175 | 0.894 | 0.112 | 5.810 | |
P Value | 0.277 | 0.862 | 0.378 | 0.911 | 0.000 |
Group | N | Prefrontal Cortices | Anterior Cingulate Cortex | Orbitofrontal Cortex | Head of Caudate Nucleus | Thalamus |
---|---|---|---|---|---|---|
OCD | 18 | 2.75 ± 0.23 | 2.92 ± 0.15 | 2.78 ± 0.23 | 1.22 ± 0.15 | 1.22 ± 0.11 |
Control | 18 | 2.77 ± 0.24 | 2.76 ± 0.21 | 2.57 ± 0.18 | 1.47 ± 0.17 | 1.35 ± 0.11 |
t statistics | -0.250 | 2.672 | 3.080 | -4.629 | -3.770 | |
P Value | 0.804 | 0.011 | 0.004 | 0.000 | 0.001 |
MRS diagrams for the thalamus; A, Healthy control group; B, OCD group; compared with the healthy controls, there was a significant increase in the value of Cho/Cr and a remarkable decrease in NAA/Cr in the OCD group (MRS, magnetic resonance spectroscopy; OCD, obsessive-compulsive disorder; Chol, choline; Cr, creatine; NAA, N-acetyl aspartate).
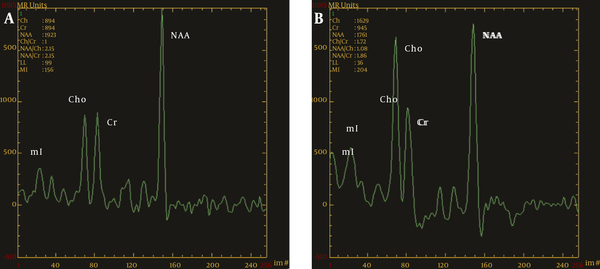
MRS diagrams for the caudate nucleus; A, Healthy control group; B, OCD group; compared with the healthy controls, the Cho/Cr value did not change significantly, and NAA/Cr was significantly decreased in the OCD patients (MRS, magnetic resonance spectroscopy; OCD, obsessive-compulsive disorder; Chol, choline; Cr, creatine; NAA, N-acetyl aspartate).
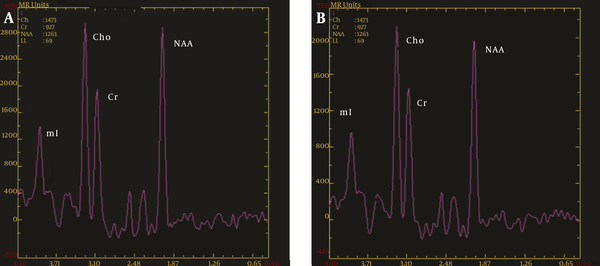
5. Discussion
Brain-circuit abnormalities may induce or directly cause neurosis. These abnormalities may also cause psychological weak points in patients, making them susceptible to neurosis under the influence of external factors. OCD patients are generally considered to have experienced organic brain changes, mainly located in the cortico-striatal-thalamic-cortical circuit (CSTC). This circuit has a direct pathway (cortico-striato-pallidal medial-thalamo-cortical) and an indirect pathway (cortico-striato-pallidal lateral-subthalamic nucleus-thalamo-cortical). The former has alienated function of exercise and the latter can inhibit unwanted movement. Excessive excitement of the direct pathway and relative inhibition of the indirect pathway can cause imbalances and OCD symptoms. When the CSTC direct pathway in refractory OCD patients is damaged surgically, the functions of both the direct pathway and the indirect pathway achieve balance, OCD symptoms are improved, and the high metabolic status of the CSTC circuit is relieved. Previous 1H-MRS studies on OCD used fragmented ROIs in the hippocampus, frontal cortex, and other brain areas, and therefore had divergent findings (13). Only a few studies have performed MRS from the loop point to explore the possible pathogenesis of OCD. This preliminary study involved early-onset OCD patients and used the CSTC loop as the ROI, as it has been implicated in the pathogenesis of OCD.
The ratio of Cho/Cr in the thalami of the OCD group was significantly higher than in the control group, with no significant differences in other parts of the brain that were assayed, suggesting that high intracellular Cho in thalamic neurons may be the pathological phenomenon or the compensatory response to OCD. Cho signaling involves phosphocholine, glycerophosphocholine, phosphatidylcholine, and sphingomyelin, and is a precursor of the neurotransmitter acetylcholine, which is closely related to memory and emotion. Cho signaling is the pathophysiological basis of mood disorders, and is one of the components of nerve envelope phospholipid metabolism. In addition, Cho is involved in the synthesis and degradation processes of cell membranes, and has been shown to reflect metabolic and functional changes in glial cells. Phosphatidylcholine is an important source of second messengers (diglycerides) that are involved in intracellular signal transduction. Therefore, the high Cho peak of the thalamus may be related to nerve cell membrane phospholipid metabolism abnormalities and intracellular signal transduction abnormalities in the area. The striatum (especially the caudate nucleus) may be the idiopathic location of the CSTC circuit in OCD, reducing inhibition to the thalamus because of abnormal striatal function and causing further thalamic gating-function defects. The thalamus facilitates the gating of information, resulting in activation of the orbitofrontal cortex (associated with obsessive thinking) and the cingulate gyrus (associated with nonspecific anxiety), ultimately resulting in compulsive thinking and behaviors. Although this activation partially compensates for the function of the striatum, it drives the thalamus to continue its gating function in order to relieve anxiety and obsessive thinking. These data show that thalamic regions may be the core regions of pathophysiology in OCD. Research results on Cho/Cr metabolic changes in OCD are still controversial (14), which may be related to the choice of ROIs.
The present research results show that the NAA/Cr ratios in the head of the caudate nucleus and the thalamus in OCD patients were significantly lower than those of the control group. A previous study by Bartha et al. (15) showed that the concentration of NAA in the left striatum in patients with OCD was significantly low. A study by Fitzgerald et al. (16) showed that NAA concentration in the medial thalamus in juvenile OCD patients was significantly decreased, and NAA concentration in the left medial thalamus in OCD patients was negatively correlated with the severity of symptoms. These results are similar to those of the present study. NAA is a peak marker of neuronal integrity and function (17). Reduction of NAA levels in the head of the caudate nucleus and thalamus in OCD patients may relate to neuronal and axonal damage from deletion, dysfunction, injury, and so on. This study also shows that the NAA/Cr ratios of the anterior cingulate and orbitofrontal cortices in OCD patients are higher than in the control group, with no significant difference in the other parts of the brain that were assayed. Russell et al. (18) showed that NAA levels in the left dorsolateral prefrontal cortex in pediatric OCD patients were significantly increased. Whiteside et al. (19) showed that NAA concentration in the right orbitofrontal white matter in OCD patients is increased compared to healthy controls. Furthermore, Fan et al. (20) showed that NAA concentration in the medial prefrontal cortex in patients with OCD was significantly increased. Increased metabolic activity in these brain neurons may cause pathological excitement of the cortical and thalamic axes, making patients unable to respond flexibly to important information. This excitement results in the emergence of habituation or ritualization of the circuit. However, not all studies have achieved consistent results. Bedard et al. (21) reported that striatum NAA/Cr ratios were significantly higher in patients with OCD, whereas the thalamus and prefrontal cortex NAA/Cr ratios were reduced. In addition, Starck et al. (22) reported that OCD was present in patients whose striatum NAA/Cr ratios were significantly increased, whereas the lower orbitofrontal and thalamus ratios showed no significant changes between the baseline characteristics of the enrolled populations.
The concentration changes of NAA reflect changes in the number and function of neurons under different pathophysiological conditions. Evidence shows that changes in local NAA concentration are reversible, and the degree of reversibility depends on the degree of neuronal injury. Jang et al. (23) showed that when drug treatment was not carried out in patients with OCD, the NAA levels in the prefrontal cortex, frontal white matter, and anterior cingulate cortex were significantly decreased. However, after 12 weeks of treatment with citalopram, the NAA levels in the prefrontal cortex and frontal white matter of the OCD patients were significantly increased. This result suggests that prefrontal area neuronal activity decreases with OCD, and that this condition is reversible. Another study found that NAA levels in the left head of the caudate nucleus in adults with OCD decreased significantly after effective behavioral therapy, and that such therapy may contribute to strong neuronal activity (3). Some studies have shown that the therapeutic effect of selective serotonin reuptake inhibitor (SSRI) drugs is related to metabolite changes of the thalamus and basal ganglia of the brain (24, 25). However, this study is not dynamically longitudinal.
Previous research on the local metabolism of the brain in patients with OCD has shown abnormalities, especially in the frontal cortex, anterior cingulate cortex, caudate nucleus, and thalamus regions of the brain. However, the differences in sample size, selection of ROI, age, gender, course of disease, administration of medication, and other confounding factors produced inconsistent results across these studies (26). The current study avoided the effects of confounding factors by selecting primary untreated OCD patients as subjects. Our results support more conclusively the hypothesis of CSTC circuit dysfunction in OCD, and show that OCD is not caused by certain types of nerve cells or structural brain lesions, but by abnormalities in different parts of the brain and multiple cerebral lesions. Due to the complexity of this mechanism, further studies on the brains of OCD patients are recommended.
References
-
1.
Menzies L, Chamberlain SR, Laird AR, Thelen SM, Sahakian BJ, Bullmore ET. Integrating evidence from neuroimaging and neuropsychological studies of obsessive-compulsive disorder: the orbitofronto-striatal model revisited. Neurosci Biobehav Rev. 2008;32(3):525-49. [PubMed ID: 18061263]. https://doi.org/10.1016/j.neubiorev.2007.09.005.
-
2.
Hagen K, Hansen B, Joa I, Larsen TK. Prevalence and clinical characteristics of patients with obsessive-compulsive disorder in first-episode psychosis. BMC Psychiatry. 2013;13:156. [PubMed ID: 23721089]. https://doi.org/10.1186/1471-244X-13-156.
-
3.
Whiteside SP, Abramowitz JS, Port JD. Decreased caudate N-acetyl-l-aspartic acid in pediatric obsessive-compulsive disorder and the effects of behavior therapy. Psychiatry Res. 2012;202(1):53-9. [PubMed ID: 22704757]. https://doi.org/10.1016/j.pscychresns.2011.11.010.
-
4.
Poyurovsky M, Faragian S, Pashinian A, Levi A, Viosburd A, Stryjer R, et al. Neurological soft signs in schizophrenia patients with obsessive-compulsive disorder. J Neuropsychiatry Clin Neurosci. 2007;19(2):145-50. [PubMed ID: 17431060]. https://doi.org/10.1176/jnp.2007.19.2.145.
-
5.
Murayama K, Nakao T, Kanba S. [Neuro-pathophysiological hypothesis of obsessive compulsive disorder based on the findings from neuroimaging studies]. Fukuoka Igaku Zasshi. 2013;104(5):81-8. [PubMed ID: 23885392].
-
6.
Cavedini P, Zorzi C, Piccinni M, Cavallini MC, Bellodi L. Executive dysfunctions in obsessive-compulsive patients and unaffected relatives: searching for a new intermediate phenotype. Biol Psychiatry. 2010;67(12):1178-84. [PubMed ID: 20381015]. https://doi.org/10.1016/j.biopsych.2010.02.012.
-
7.
Schiepek G, Tominschek I, Karch S, Mulert C, Pogarell O. [Neuroimaging and the neurobiology of obsessive-compulsive disorder]. Psychother Psychosom Med Psychol. 2007;57(9-10):379-94. [PubMed ID: 17357901]. https://doi.org/10.1055/s-2006-952021.
-
8.
Del Casale A, Kotzalidis GD, Rapinesi C, Serata D, Ambrosi E, Simonetti A, et al. Functional neuroimaging in obsessive-compulsive disorder. Neuropsychobiology. 2011;64(2):61-85. [PubMed ID: 21701225]. https://doi.org/10.1159/000325223.
-
9.
Kwon JS, Jang JH, Choi JS, Kang DH. Neuroimaging in obsessive-compulsive disorder. Expert Rev Neurother. 2009;9(2):255-69. [PubMed ID: 19210199]. https://doi.org/10.1586/14737175.9.2.255.
-
10.
Atmaca M, Yildirim H, Ozdemir H, Tezcan E, Poyraz AK. Volumetric MRI study of key brain regions implicated in obsessive-compulsive disorder. Prog Neuropsychopharmacol Biol Psychiatry. 2007;31(1):46-52. [PubMed ID: 16859819]. https://doi.org/10.1016/j.pnpbp.2006.06.008.
-
11.
Karlas T, Petroff D, Garnov N, Bohm S, Tenckhoff H, Wittekind C, et al. Non-invasive assessment of hepatic steatosis in patients with NAFLD using controlled attenuation parameter and 1H-MR spectroscopy. PLoS One. 2014;9(3):91987. [PubMed ID: 24637477]. https://doi.org/10.1371/journal.pone.0091987.
-
12.
Lapidus KA, Gabbay V, Mao X, Johnson A, Murrough JW, Mathew SJ, et al. In vivo (1)H MRS study of potential associations between glutathione, oxidative stress and anhedonia in major depressive disorder. Neurosci Lett. 2014;569:74-9. [PubMed ID: 24704328]. https://doi.org/10.1016/j.neulet.2014.03.056.
-
13.
Lazaro L, Bargallo N, Andres S, Falcon C, Morer A, Junque C, et al. Proton magnetic resonance spectroscopy in pediatric obsessive-compulsive disorder: longitudinal study before and after treatment. Psychiatry Res. 2012;201(1):17-24. [PubMed ID: 22281202]. https://doi.org/10.1016/j.pscychresns.2011.01.017.
-
14.
O'Neill J, Piacentini JC, Chang S, Levitt JG, Rozenman M, Bergman L, et al. MRSI correlates of cognitive-behavioral therapy in pediatric obsessive-compulsive disorder. Prog Neuropsychopharmacol Biol Psychiatry. 2012;36(1):161-8. [PubMed ID: 21983143]. https://doi.org/10.1016/j.pnpbp.2011.09.007.
-
15.
Bartha R, Stein MB, Williamson PC, Drost DJ, Neufeld RW, Carr TJ, et al. A short echo 1H spectroscopy and volumetric MRI study of the corpus striatum in patients with obsessive-compulsive disorder and comparison subjects. Am J Psychiatry. 1998;155(11):1584-91. [PubMed ID: 9812122]. https://doi.org/10.1176/ajp.155.11.1584.
-
16.
Fitzgerald KD, Moore GJ, Paulson LA, Stewart CM, Rosenberg DR. Proton spectroscopic imaging of the thalamus in treatment-naive pediatric obsessive-compulsive disorder. Biol Psychiatr. 2000;47(3):174-82.
-
17.
Olvera RL, Caetano SC, Stanley JA, Chen HH, Nicoletti M, Hatch JP, et al. Reduced medial prefrontal N-acetyl-aspartate levels in pediatric major depressive disorder: a multi-voxel in vivo(1)H spectroscopy study. Psychiatry Res. 2010;184(2):71-6. [PubMed ID: 20864319]. https://doi.org/10.1016/j.pscychresns.2010.07.008.
-
18.
Russell A, Cortese B, Lorch E, Ivey J, Banerjee SP, Moore GJ, et al. Localized functional neurochemical marker abnormalities in dorsolateral prefrontal cortex in pediatric obsessive-compulsive disorder. J Child Adolesc Psychopharmacol. 2003;13 Suppl 1:31-8. [PubMed ID: 12880498]. https://doi.org/10.1089/104454603322126322.
-
19.
Whiteside SP, Port JD, Deacon BJ, Abramowitz JS. A magnetic resonance spectroscopy investigation of obsessive-compulsive disorder and anxiety. Psychiatry Res. 2006;146(2):137-47. [PubMed ID: 16507346]. https://doi.org/10.1016/j.pscychresns.2005.12.006.
-
20.
Fan Q, Tan L, You C, Wang J, Ross CA, Wang X, et al. Increased N-Acetylaspartate/creatine ratio in the medial prefrontal cortex among unmedicated obsessive-compulsive disorder patients. Psychiatry Clin Neurosci. 2010;64(5):483-90. [PubMed ID: 20923427]. https://doi.org/10.1111/j.1440-1819.2010.02128.x.
-
21.
Bedard MJ, Chantal S. Brain magnetic resonance spectroscopy in obsessive-compulsive disorder: the importance of considering subclinical symptoms of anxiety and depression. Psychiatry Res. 2011;192(1):45-54. [PubMed ID: 21377338]. https://doi.org/10.1016/j.pscychresns.2010.10.008.
-
22.
Starck G, Ljungberg M, Nilsson M, Jonsson L, Lundberg S, Ivarsson T, et al. A 1H magnetic resonance spectroscopy study in adults with obsessive compulsive disorder: relationship between metabolite concentrations and symptom severity. J Neural Transm (Vienna). 2008;115(7):1051-62. [PubMed ID: 18528631]. https://doi.org/10.1007/s00702-008-0045-4.
-
23.
Jang JH, Kwon JS, Jang DP, Moon WJ, Lee JM, Ha TH, et al. A proton MRSI study of brain N-acetylaspartate level after 12 weeks of citalopram treatment in drug-naive patients with obsessive-compulsive disorder. Am J Psychiatry. 2006;163(7):1202-7. [PubMed ID: 16816225]. https://doi.org/10.1176/appi.ajp.163.7.1202.
-
24.
Mohamed MA, Smith MA, Schlund MW, Nestadt G, Barker PB, Hoehn-Saric R. Proton magnetic resonance spectroscopy in obsessive-compulsive disorder: a pilot investigation comparing treatment responders and non-responders. Psychiatry Res. 2007;156(2):175-9. [PubMed ID: 17904826]. https://doi.org/10.1016/j.pscychresns.2007.04.002.
-
25.
Shin LM, Davis FC, Vanelzakker MB, Dahlgren MK, Dubois SJ. Neuroimaging predictors of treatment response in anxiety disorders. Biol Mood Anxiety Disord. 2013;3(1):15. [PubMed ID: 23915782]. https://doi.org/10.1186/2045-5380-3-15.
-
26.
Brennan BP, Rauch SL, Jensen JE, Pope HG. A critical review of magnetic resonance spectroscopy studies of obsessive-compulsive disorder. Biol Psychiatry. 2013;73(1):24-31. [PubMed ID: 22831979]. https://doi.org/10.1016/j.biopsych.2012.06.023.