Abstract
Background:
Impaired wound healing is one of the complications of diabetes. Carvacrol, a natural substance, shows promising results in diabetic wound healing despite the fact that its therapeutic mechanisms are not fully understood.Objectives:
The aim of this study was to investigate the molecular alterations caused by carvacrol intervention in human dermal fibroblasts (HDFs) cultured at a high-glucose condition.Methods:
The HDFs were incubated in different glucose concentrations, and cell viability was assessed. In addition, carvacrol cytotoxicity was determined. Then, the HDFs were incubated in 50 mM glucose prior to treatment. After that, the cells were divided into 4 groups: Controls, high-glucose (50 mM), carvacrol-treated (9 µM), and high-glucose carvacrol-treated for 24 h. Cell proliferation and migration were examined. Furthermore, superoxide dismutase (SOD) production, collagen deposition, and RNA levels of TGFβ1, ACTA2, and miR-155 were investigated.Results:
The in vitro scratch assay revealed that the fibroblast migration, which was reduced at high-glucose concentration, was reversed due to the carvacrol intervention during 12 h and 24 h (P < 0.01 and P < 0.001, respectively). Collagen deposition and SOD synthesis showed an increase in treated cells (P < 0.001). Both TGFβ1 and ACTA2 mRNA expressions were elevated due to the carvacrol treatment, while the miR-155 level decreased (P < 0.001).Conclusions:
A high level of glucose impaired the cellular function of human dermal fibroblast. Carvacrol reversed the adverse effects of high-glucose stress and promoted wound healing through greater cell migration, collagen deposition, and levels of TGFb1 and ACTA2 gene expression. It showed inhibitory effects against miR-155, which is known for its negative role in diabetic wound healing.Keywords
1. Background
Diabetes mellitus (DM) is a health-threatening chronic disorder. Constant high glucose is associated with a wide range of complications, including impaired wound healing, especially in the lower limbs (1). Diabetic wounds are highly susceptible to infection, resistant to treatment, and prone to recurrence (2). A global increase in the number of diabetic patients has led to a higher number of diabetic wound cases, which, in turn, has burdened health systems due to greater costs (1). Hence, discovering an alternative therapy for diabetic wounds is of importance in developing countries to reduce costs.
Since ancient times, medicinal plants have been used to treat wounds. It seems that bioactive compounds from herbs can be considered in this approach (3, 4). Carvacrol (C10H14O) is the main monoterpene of oregano and thyme. It has also been found in essential oils extracted from various plants (5). In addition, carvacrol synthesis by chemical and biotechnological methods is conveniently applicable. Carvacrol exerts its antibacterial potential against both Gram-positive and -negative bacteria involved in diabetic wound infection (6). Besides, some studies have indicated the ameliorating effects of carvacrol on wound healing via antioxidant and anti-inflammatory properties (5, 7).
Wound healing consists of 4 overlapping sequential phases: Hemostasis, inflammation, proliferation, and remodeling (5). In DM patients suffering from ulcers, all of these phases are affected; however, the underlying mechanism of impaired wound repair is not fully understood (8). Human dermal fibroblasts (HDFs) are central players in all the stages of wound healing. When the wound occurs, HDFs migrate to the wound site and proliferate. Some of the migrated HDFs are differentiated to myofibroblasts to aid wound closure by their contractile properties. In the next step, the proliferated fibroblasts produce the extracellular matrix (ECM), which is crucial for granulation and facilitates the migration of immune system cells. Moreover, they secret different types of growth factors and cytokines to modulate signaling interactions, maintain cellular status, and regulate ECM production (9, 10).
Delayed wound healing in DM patients occurs through various processes in which HDFs are involved. For instance, during impaired wound healing in diabetics, the rise of reactive oxygen species (ROS) and the reduction of collagen in ECM are observed (11). Hyperglycemia establishes an oxidizing environment, which impairs wound healing and can decrease the production of transforming growth factor β1 (TGFβ1); TGFβ1 is of importance in the deposition of ECM and recruitment of inflammatory cells. Furthermore, decreasing levels of TGFβ reduce the expression of α-smooth muscle actin (αSMA), which in turn can decelerate fibroblast to myofibroblast differentiation (11, 12). Superoxide dismutases (SODs) are cellular antioxidant enzymes which are the front-line fighters against ROS. It has been revealed that the expression of SOD is declined in diabetic wounds. The decline of SOD leads to the decrease of TGFβ1 and αSMA expressions, as well as the decrease in collagen secretion (13, 14).
It has been documented that hyperglycemia can change the expressive levels of several miRNAs, including miR-155 (15). Increased expression of miR-155 is observed in diabetic skin (16). Wang et al. showed that miR-155 knockout mice's wounds improved more appropriately than their diabetic counterparts (17). Moreover, the inhibition of miR-155 led to improvement in diabetic wound healing (18, 19).
2. Objectives
The HDFs growing under high-glucose stress can be considered an appropriate in vitro model for diabetic wound healing. The current study aimed to evaluate the competence of carvacrol in collagen deposition and wound closure and investigate its ability to modulate miR-155, TGFβ1, and αSMA RNA levels, as well as collagen and SOD proteins of HDFs.
3. Methods
3.1. Cell Culture
Human dermal fibroblasts from adult foreskin were purchased from the Royan Institute for Stem Cell Biology and Technology (Iran). The HDFs were cultured in Dulbecco's Modified Eagle's Medium (DMEM, Gibco, Germany), supplemented with 10% fetal bovine serum (FBS, Gibco, Germany) and 1% penicillin-streptomycin. Then, they were incubated at 37°C in 5% CO2. The cells from 4 - 6 passages were used for further experiments. The control group consisted of HDFs incubated in normal glucose concentrations (5.5 mM). A diabetic wound model was obtained by continuous incubation under hyperglycemic stress (25, 50, and 75 mM/L glucose concentrations) 72 hours prior to analysis, as previously described by Rashnavandi et al. (20). Mannitol was added to the test groups to control the osmotic pressure.
3.2. 3-(4,5-Dimethylthiazol-2-yl)-2,5-Diphenyltetrazolium Bromide Assay
The MTT (3-(4,5-dimethylthiazol-2-yl)-2,5 -diphenyltetrazolium bromide) assay was carried out to examine the cytotoxicity of glucose concentrations. A total of 10000 HDFs were seeded in 200 µL DMEM in 96-well plates for 24 h. Next, the cells were exposed to the different glucose concentrations (5.5, 25, 50, and 75 mM) for 24, 48 and 72 hours. Furthermore, the appropriate carvacrol concentrations were evaluated in 5.5 mM glucose at 24, 48, and 72 h. After the incubation, the supernatant was removed, and wells were washed with Phosphate-buffered saline (PBS). Next, 200 µL MTT (10% in PBS) was added to each well and incubated at 37°C. After 3 hours of incubation, 60 µL DMSO was added for 20 min to solubilize formazan crystals. Finally, the optical density (OD) was recorded at 57 nm with a spectrophotometer (Thermo-Fisher, USA). The half-maximal inhibitory concentration (IC50) of carvacrol was determined in each group, and the 9 µL concentration of carvacrol was chosen as our interventive dose. For further analysis, 4 groups were determined as follows: Control (without any treatment), high-glucose (50 mM glucose), carvacrol (treated with 9 µL), glucose + carvacrol (added 50 mM glucose and 9 µL carvacrol). The test period was adjusted to 24 h.
3.3. Scratch Assay
The in vitro wound model for HDFs was simulated by the scratch assay in triplicate. In brief, 5x104 cells were seeded in 6-well plates filled with DMEM until 80% confluency was obtained. Then, the treated cells were exposed to 50 mM glucose, and 9 µL of carvacrol was used to assess its potential therapeutic ability. The confluent monolayer was scraped by a sterile 200 µL pipette tip to create a cell-free zone surrounded by cells on both sides of the wound. PBS was used to remove cell debris, and a fresh medium was added instantly. Subsequently, the cells were observed through a phase contrast microscope for 24 hours and imaged.
3.4. Hydroxyproline Assay
The collagen quantity was investigated by a hydroxyproline ELISA kit according to the manufacturer's protocol (Kiazist Co., Iran). Hydroxyproline is one of the main components of collagen and the main composition of the ECM. The given amino acid exclusively exists in collagen; hence, the measurement of the hydroxyproline can directly indicate the amount of collagen. The sample was digested by strong acid and subsequently oxidated. The oxidative product reacted to chromogen. The absorbance was measured at 540 - 560 nm. The concentration of collagen was calculated by comparing the obtained data with the standard curve.
3.5. Superoxide Dismutase Assay
In the current study, the SOD activity was determined by a fluorometric ELISA kit (Kiazist Co., Iran). The kit determines the superoxide radicals produced by xanthine oxidase. These particular radicals reduce resazurin to fluorochrome resorufin, which is detectable at 570 nm (Ex/Em = 572.586). Therefore, SOD activity can be estimated as the percentage of reduction of resorufin. The unit of SOD activity is described by the inhibition rate of 50%.
3.6. Gene Expression Assay
Total RNA was isolated from cells (5x106) by RiboEx Total RNA Kit (GENE ALL, South Korea) according to the manufacturer's protocol. The purity and concentration of the extracted RNA were investigated by NanoDrop (Berthold, Germany). Samples with the 260/280 = 1.8 - 2.2 and the 230/260 = 1.7 - 1.9 were considered validated for the cDNA synthesis. The complementary DNA (cDNA) was produced by the Easy cDNA Synthesis Kit (Cat.No.A101161). Three genes (TGFβ2, ACTA2, and miR-155-5p) were designated for amplification. GAPDH and U6 were used as internal control genes for mRNAs and miRNA, respectively. Primers were designed by Gene Runner, and their sensitivity was affirmed by the National Center for Biotechnology Information (NCBI) Primer-BLAST (https://www.ncbi.nlm.nih.gov/tools/primer-blast/) (Table 1). The amplification process consisted of 95°C for 4 min, followed by 40 cycles of 94°C for 30 s, 60°C for 30 s, and 72°C for 30 s, as well as final extension at 72°C for 5 min. The quantitative polymerase chain reaction (PCR) was conducted by Rotor-Gene 6000 (Corbett Research, Australia). 2-ΔΔCt was used to calculate the fold change of gene expression.
Designed Primers Used in This Study
Gene | Forward Primer Sequence (5' - 3') | Reverse Primer Sequence (5' - 3') |
---|---|---|
TGFβ1 | ATTTGGAGCCTGGACACGCA | AGTACACGATGGGCAGCGG |
ACTA2 | CCAACTGGGACGACATGGAA | ATTTTCTCCCGGTTGGCCTT |
GAPDH | CTCATTTCCTGGTATGACAACG | CTTCCTCTTGTGCTCTTGCT |
MiR-155-5p | GCGTCGCTTAATGCTAATCG | GTGCAGGGTCCGAGGTAT |
U6 | CTCGCTTCGGCAGCACA | AACGCTTCACGAATTTGCGT |
3.7. Statistical Analysis
All the experiments were conducted in triplicate. All the data were presented as mean ± standard deviation (SD). The one-way analysis of variance (ANOVA) followed by Tukey's post-hoc test was used to evaluate the differences. Pearson correlation was used to validate the relationships among biological mechanisms. GraphPad Prism v. 8 software performed the statistical analysis. P < 0.05 was considered statistically significant.
4. Results
4.1. Cell Viability and Migration
The cytotoxicity of different glucose and carvacrol concentrations on HDFs was assessed by MTT. The half-maximal inhibitory concentration (IC50) of carvacrol at 24, 48, and 72h was 22.45, 18.49, and 9.34 µM, respectively (Figure 1A). The decrease in cell viability was dose- and time-dependent. Glucose dose-dependently decreased cell proliferation. Regarding time, cells proliferated in an increasing trend after 48 h in glucose-treated groups. This could be due to mechanisms activated to tolerate high-glucose stress (Figure 1B). The change of the cell viability rate according to time was significantly different (P < 0.001) except for the control group and 50 mM glucose at 48 and 72 h. The carvacrol treatment altered the cell proliferation in each treatment group time-dependently (P < 0.0001). The scratch assay was performed to determine carvacrol competence in wound closure. The cell migration analysis demonstrated that the carvacrol treatment could considerably restore the migration ability of HDFs (Figure 1C and D).
A, cell viability of HDFs in different glucose concentrations (mM) and carvacrol intervention in 24, 48, and 72-h culture; B, MTT assay results of HDFs treated with carvacrol; C, the images present the effects of carvacrol intervention at 50 mM glucose concentration on wound healing in 2, 12, and 24 h; D, carvacrol ameliorated wound closure process in high-glucose condition after 24 h (Data are presented as mean ± SD, **P < 0.01, ***P < 0.001). Abbreviations: HDF, human dermal fibroblasts; MTT, (3-(4, 5-dimethylthiazolyl-2)-2, 5-diphenyltetrazolium bromide; SD, standard deviation.
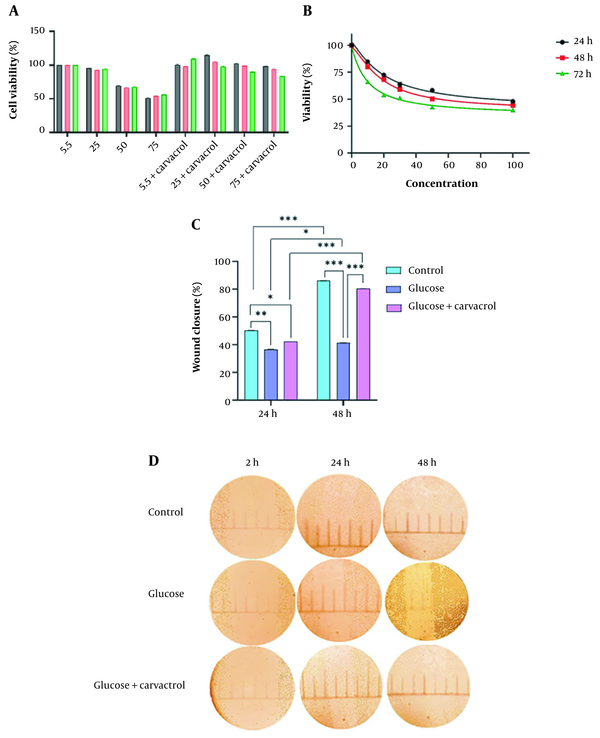
4.2. Collagen Deposition
Collagen deposition was investigated to assess carvacrol's influence on wound repair by producing the ECM. The results indicated that carvacrol ameliorated collagen deposition. The HDFs treated with high glucose showed a decrease in collagen deposition (P < 0.001). Although significantly increased collagen production was observed in intervention cells (in comparison to high-glucose cells, P < 0.001), the collagen produced among these cells was approximately equal to the normal cells (P < 0.661) (Figure 2A).
A, the content of collagen produced by HDFs; B, the SOD activity of HDfs in non-treated, high-glucose stressed, carvacrol-treated, and high-glucose carvacrol-treated groups (ns, nonsignificant; ***P < 0.001). Abbreviations: HDF, human dermal fibroblasts; SOD, superoxide dismutase.
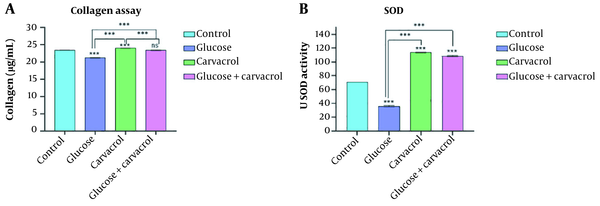
4.3. Superoxide Dismutase Activity
Superoxide dismutase plays an important role in the detoxification of unregulated ROS. In this study, high-glucose stress alleviated the SOD (P < 0.001). The use of carvacrol in both normal and high-glucose environments led to a significant increase in SOD production compared to controls and high-glucose cells (P < 0.001) (Figure 2B).
4.4. Gene Expression
Real-time PCR was carried out to assess the adverse effects of high glucose and carvacrol intervention on TGFβ1, ACTA2, and miR-155 RNA levels. As shown in Figure 3A, TGFβ1 expression nonsignificantly decreased in the high-glucose condition, while experiencing carvacrol intervention increased its levels dramatically (P < 0.001). ACTA2 expression alleviated drastically in the high-glucose condition (P < 0.001). Carvacrol treatment raised ACTA2 levels (P < 0.001) in different conditions; however, the increased level was more noticeable in normal-treated cells (P < 0.001) (Figure 3B). Somewhat like its controversial role in wound healing, the miR-155 expression pattern was intriguing. As presented in Figure 3C, there was a significant enhancement of miR-155 in the high-glucose condition and normal cells treated with carvacrol (P < 0.001) compared to the controls. However, the results indicated that the simultaneous effects of glucose and carvacrol did not change the miR-155 levels compared to the controls. In our study, the expression of ACTA2 was significantly correlated to the collagen deposition (r = 0.9695, P = 0.0153). On the other hand, the altered mRNA levels of TGFβ1 and ACTA2 were correlated to SOD production (r = 0.9526, P = 0.0237; r = 0.9218, P = 0.0391, respectively).
Relative gene expression of (A) TGFβ1, (B) ACTA2, and (C) miR-155, measured by real-time PCR in non-treated, grown at the high-glucose environment, carvacrol-treated and high-glucose-grown carvacrol-treated groups after 24 h (ns, nonsignificant; ***P < 0.001). Abbreviation: PCR, polymerase chain reaction.
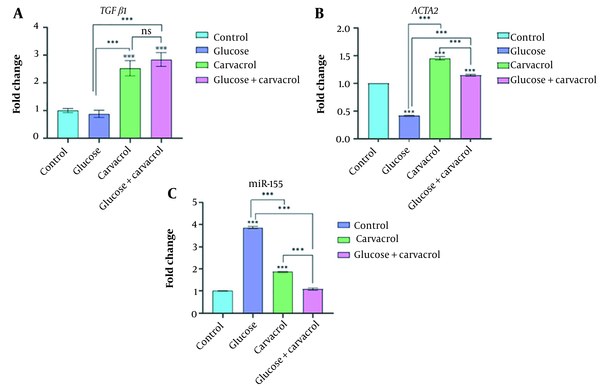
5. Discussion
Numerous researchers have demonstrated the impaired wound-healing process in diabetic patients (1, 2). Although fibroblasts showed high resistance against elevated levels of glucose (21), their dysfunction has been documented in the diabetic environment (11). Natural products are considered an alternative therapeutic policy for dermal repair. We used carvacrol as a novel approach for the treatment of hyperglycemic wounds. The antibacterial and anti-inflammatory properties of carvacrol make it a potential therapeutic candidate for diabetic ulcers. This study aimed to clarify the possible role of carvacrol on the major markers of the wound healing process.
The cytotoxic effects of carvacrol on fibroblasts were determined through colorimetric MTT assay. Our findings suggested that the carvacrol might be noncytotoxic against fibroblasts and potentially induce cell proliferation at a dose of 9 µM. On the other hand, concurrent with our results, a systematic review by Costa et al. about the effects of carvacrol essential oil on wound healing revealed its ability to upregulate the growth of fibroblasts (5).
Cell migration in the current study was investigated by the scratch assay. Our results showed a diminished cell migration in 50 mM glucose. This observation, besides the lower cell proliferation in hyperglycemic conditions, is in line with previous studies; this finding suggests that high glucose doses alleviate cell proliferation and migration in fibroblasts obtained in diabetic patients (22). Following the carvacrol administration, HDFs showed migration acceleration. Several studies have reported decreased cell proliferation and migration when carvacrol was used against cancerous cells (23-25). The reason for this disagreement can be that normal human fibroblasts were used in the current study, while other studies were designed to evaluate carvacrol antioxidant properties against cancer cells. The role of ROS in cell life is controversial.
Collagen deposition by HDFs is required for inflammation modulation, angiogenesis, and remodeling during wound healing (26). Our results confirmed that carvacrol might ameliorate the dermal healing process by collagen production. The cells growing in hyperglycemic conditions deposited collagen approximately equivalent to their normal counterparts after treatment with carvacrol. In addition, HDFs treated with carvacrol in the normal condition produced significantly more collagen in comparison to normal cells. This observation suggests that carvacrol is a beneficial agent for treating normal wounds. In a study conducted by Lee et al., the influence of carvacrol on collagen deposition was traced. They reported that carvacrol activated COL1A2 promoter and type 1 collagen synthesis. This occurs via the transcriptional induction of AP-1 and phosphorylation of JNK and ERK1/2. Besides, carvacrol stimulates intracellular mobilization of Ca2+ and PLCγ1 (27).
To maintain the stability of tissues during cell migration, the ECM must be produced constantly. SOD, an antioxidant enzyme, is a factor that plays a role in the induction of collagen production (28). The inadequate expression of SOD in diabetic wounds is a mediator of impaired wound healing. Moreover, the hyperglycemic environment induces the oxidative stress (29). Superoxide dismutase secretion was evaluated by a fluorometric ELISA kit. The hyperglycemic conditions lead to a reduction in SOD expression, which, in turn, attenuates the wound-healing process. Our findings showed that carvacrol intervention significantly ameliorates skin repair processes in both normal and hyperglycemic conditions. In line with our results, several studies have confirmed the role of carvacrol in improving redox balance by increasing the expression of SOD (5, 30).
Our study demonstrated that the treated HDFs had significantly higher mRNA levels of TGFβ1 and ACTA2, while the expression of miR-155 decreased significantly compared to untreated HDFs in hyperglycemic conditions. Our results indicated the relationship between SOD and TGFβ1 mRNA expression. TGFβ1, secreted by fibroblasts, is associated with proliferation, collagen synthesis and deposition, and angiogenesis. TGFβ1 indirectly induces the expression of collagen and αSMA via various signaling pathways, including Smad, ERK/MAPK, and PI3-Akt-mTOR (31, 32). αSMA is a marker of myofibroblasts and is involved in inflammatory response, collagen deposition, and wound contraction (12). These findings indicated a significant correlation between SOD production, collagen deposition, and ACTA2 expression, which is consistent with other studies (14, 33).
In the inflammatory phase, miR-155 expression is induced to perform its regulatory role in immune responses. It is estimated that miR-155 contributes to collagen deposition and myofibroblast differentiation. However, it is believed that in the remodeling phase, its expression negatively affects re-epithelization and wound closure; along with increasing inflammation, it decelerates the wound-healing process (15, 16, 34). Eissa and Artlett concluded that miR-155 was related to lung fibrosis by promoting the expression of COL1A1 and αSMA (35). Furthermore, Yang et al. showed that the overexpression of miR-155 could accelerate wound healing (36). On the other hand, some studies on diabetic wound healing have revealed the adverse aspects of miR-155 high expression (16, 19). Our results supported the latter reports, which demonstrated that the decrease of miR-155 under the influence of carvacrol was associated with healing. It shows the controversial role of miR-155 in wound healing, particularly in diabetic patients. Further studies are needed to determine the role of miR-155 and its proper levels in skin repair.
One of the limitations of the current study was that αSMA and TGFβ1 were not investigated at the protein level. In addition, the assessment of carvacrol treatment on the studied factors in the current study must be confirmed in animal models. Although we tried to provide new information to elaborate on the potency of carvacrol in diabetic wound healing, more detailed studies and molecular techniques, such as RNA-seq, are needed to clarify its role in this issue.
5.1. Conclusions
In conclusion, carvacrol ameliorated collagen deposition and wound closure in HDFs. Increased mRNA levels of TGFβ1 and ACTA2 and decreased levels of miR-155 were observed at the 9 µM concentration of carvacrol. Besides its antimicrobial effects, its anti-inflammatory, pro-proliferative, and remodeling properties in wound healing were shown. These results can be considered biological evidence for further investigation regarding carvacrol as an inexpensive natural product. Further studies are required to precisely understand carvacrol's potential in diabetic wound healing.
References
-
1.
Hagde P, Pingle P, Mourya A, Katta CB, Srivastava S, Sharma R, et al. Therapeutic potential of quercetin in diabetic foot ulcer: Mechanistic insight, challenges, nanotechnology driven strategies and future prospects. J Drug Deliv Sci Technol. 2022;74. https://doi.org/10.1016/j.jddst.2022.103575.
-
2.
Liu Y, Liu Y, Deng J, Li W, Nie X. Fibroblast growth factor in diabetic foot ulcer: Progress and therapeutic prospects. Front Endocrinol (Lausanne). 2021;12:744868. [PubMed ID: 34721299]. [PubMed Central ID: PMC8551859]. https://doi.org/10.3389/fendo.2021.744868.
-
3.
Herman A, Herman AP. Herbal products and their active constituents for diabetic wound healing-preclinical and clinical studies: A systematic review. Pharmaceutics. 2023;15(1). [PubMed ID: 36678910]. [PubMed Central ID: PMC9865817]. https://doi.org/10.3390/pharmaceutics15010281.
-
4.
Xu Z, Dong M, Yin S, Dong J, Zhang M, Tian R, et al. Why traditional herbal medicine promotes wound healing: Research from immune response, wound microbiome to controlled delivery. Adv Drug Deliv Rev. 2023;195:114764. [PubMed ID: 36841332]. https://doi.org/10.1016/j.addr.2023.114764.
-
5.
Costa MF, Durco AO, Rabelo TK, Barreto RSS, Guimaraes AG. Effects of carvacrol, thymol and essential oils containing such monoterpenes on wound healing: A systematic review. J Pharm Pharmacol. 2019;71(2):141-55. [PubMed ID: 30537169]. https://doi.org/10.1111/jphp.13054.
-
6.
Marinelli L, Di Stefano A, Cacciatore I. Carvacrol and its derivatives as antibacterial agents. Phytochem Rev. 2018;17(4):903-21. https://doi.org/10.1007/s11101-018-9569-x.
-
7.
Tabibzadeh Dezfuli SA, Ehsani M, Lakzaei Azar O. Carvacrol alleivated negative effects of diabetes on inflammation and oxidation by modulation in gene expression of inflammatory and antioxidant system in diabetic rat model. GMJ Medical. 2017:15-20. https://doi.org/10.29088/gmjm.2017.15.
-
8.
Lerman OZ, Galiano RD, Armour M, Levine JP, Gurtner GC. Cellular dysfunction in the diabetic fibroblast: impairment in migration, vascular endothelial growth factor production, and response to hypoxia. Am J Pathol. 2003;162(1):303-12. [PubMed ID: 12507913]. [PubMed Central ID: PMC1851127]. https://doi.org/10.1016/S0002-9440(10)63821-7.
-
9.
Al-Rikabi AHA, Tobin DJ, Riches-Suman K, Thornton MJ. Dermal fibroblasts cultured from donors with type 2 diabetes mellitus retain an epigenetic memory associated with poor wound healing responses. Sci Rep. 2021;11(1):1474. [PubMed ID: 33446687]. [PubMed Central ID: PMC7809350]. https://doi.org/10.1038/s41598-020-80072-z.
-
10.
Du J, Liu X, Wong CWY, Wong KKY, Yuan Z. Direct cellular reprogramming and transdifferentiation of fibroblasts on wound healing-Fantasy or reality? Chronic Dis Transl Med. 2023;9(3):191-9. [PubMed ID: 37711868]. [PubMed Central ID: PMC10497843]. https://doi.org/10.1002/cdt3.77.
-
11.
Moura LI, Dias AM, Suesca E, Casadiegos S, Leal EC, Fontanilla MR, et al. Neurotensin-loaded collagen dressings reduce inflammation and improve wound healing in diabetic mice. Biochim Biophys Acta. 2014;1842(1):32-43. [PubMed ID: 24161538]. https://doi.org/10.1016/j.bbadis.2013.10.009.
-
12.
Levinson H. A paradigm of fibroblast activation and dermal wound contraction to guide the development of therapies for chronic wounds and pathologic scars. Adv Wound Care (New Rochelle). 2013;2(4):149-59. [PubMed ID: 24527338]. [PubMed Central ID: PMC3840547]. https://doi.org/10.1089/wound.2012.0389.
-
13.
Younus H. Therapeutic potentials of superoxide dismutase. Int J Health Sci (Qassim). 2018;12(3):88-93. [PubMed ID: 29896077]. [PubMed Central ID: PMC5969776].
-
14.
Setyawati A, Wahyuningsih MSH, Nugrahaningsih DAA, Effendy C, Fneish F, Fortwengel G. Piper crocatum Ruiz & Pav. ameliorates wound healing through p53, E-cadherin and SOD1 pathways on wounded hyperglycemia fibroblasts. Saudi J Biol Sci. 2021;28(12):7257-68. [PubMed ID: 34867030]. [PubMed Central ID: PMC8626332]. https://doi.org/10.1016/j.sjbs.2021.08.039.
-
15.
Jankauskas SS, Gambardella J, Sardu C, Lombardi A, Santulli G. Functional role of miR-155 in the pathogenesis of diabetes mellitus and its complications. Noncoding RNA. 2021;7(3). [PubMed ID: 34287359]. [PubMed Central ID: PMC8293470]. https://doi.org/10.3390/ncrna7030039.
-
16.
Xu M, Li Y, Tang Y, Zhao X, Xie D, Chen M. Increased expression of miR-155 in peripheral blood and wound margin tissue of type 2 diabetes mellitus patients associated with diabetic foot ulcer. Diabetes Metab Syndr Obes. 2022;15:3415-28. [PubMed ID: 36353665]. [PubMed Central ID: PMC9639392]. https://doi.org/10.2147/DMSO.S376292.
-
17.
Wang CR, Zhu HF, Zhu Y. Knockout of MicroRNA-155 ameliorates the Th17/Th9 immune response and promotes wound healing. Curr Med Sci. 2019;39(6):954-64. [PubMed ID: 31845227]. https://doi.org/10.1007/s11596-019-2128-x.
-
18.
Gondaliya P, Sayyed AA, Bhat P, Mali M, Arya N, Khairnar A, et al. Mesenchymal stem cell-derived exosomes loaded with miR-155 inhibitor ameliorate diabetic wound healing. Mol Pharm. 2022;19(5):1294-308. [PubMed ID: 35294195]. https://doi.org/10.1021/acs.molpharmaceut.1c00669.
-
19.
Ye J, Kang Y, Sun X, Ni P, Wu M, Lu S. MicroRNA-155 inhibition promoted wound healing in diabetic rats. Int J Low Extrem Wounds. 2017;16(2):74-84. [PubMed ID: 28682732]. https://doi.org/10.1177/1534734617706636.
-
20.
Rashnavadi MH, Tahmasebi Z, Piravar Z, Gholi AM. N-Acetyl-L-cysteine effects on oxidative stress-induced high glucose-cultured human dermal fibroblasts. Gene Reports. 2022;26. https://doi.org/10.1016/j.genrep.2022.101522.
-
21.
Yu J, Nam D, Park KS. Substance P enhances cellular migration and inhibits senescence in human dermal fibroblasts under hyperglycemic conditions. Biochem Biophys Res Commun. 2020;522(4):917-23. [PubMed ID: 31806373]. https://doi.org/10.1016/j.bbrc.2019.11.172.
-
22.
Karlowicz-Bodalska K, Han S, Freier J, Smolenski M, Bodalska A. Curcuma longa as medicinal herb in the treatment of diabet- IC complications. Acta Pol Pharm. 2017;74(2):605-10. [PubMed ID: 29624265].
-
23.
Arunasree KM. Anti-proliferative effects of carvacrol on a human metastatic breast cancer cell line, MDA-MB 231. Phytomedicine. 2010;17(8-9):581-8. [PubMed ID: 20096548]. https://doi.org/10.1016/j.phymed.2009.12.008.
-
24.
Jung CY, Kim SY, Lee C. Carvacrol targets AXL to inhibit cell proliferation and migration in non-small cell lung cancer cells. Anticancer Res. 2018;38(1):279-86. [PubMed ID: 29277784]. https://doi.org/10.21873/anticanres.12219.
-
25.
Fatima K, Luqman S, Meena A. Carvacrol arrests the proliferation of hypopharyngeal carcinoma cells by suppressing ornithine decarboxylase and hyaluronidase activities. Front Nutr. 2022;9:857256. [PubMed ID: 35464036]. [PubMed Central ID: PMC9028219]. https://doi.org/10.3389/fnut.2022.857256.
-
26.
Mathew-Steiner SS, Roy S, Sen CK. Collagen in wound healing. Bioengineering (Basel). 2021;8(5). [PubMed ID: 34064689]. [PubMed Central ID: PMC8151502]. https://doi.org/10.3390/bioengineering8050063.
-
27.
Lee J, Jung E, Yu H, Kim Y, Ha J, Kim YS, et al. Mechanisms of carvacrol-induced expression of type I collagen gene. J Dermatol Sci. 2008;52(3):160-9. [PubMed ID: 18701258]. https://doi.org/10.1016/j.jdermsci.2008.06.007.
-
28.
Lee MJ, Agrahari G, Kim HY, An EJ, Chun KH, Kang H, et al. Extracellular superoxide dismutase prevents skin aging by promoting collagen production through the activation of AMPK and Nrf2/HO-1 cascades. J Invest Dermatol. 2021;141(10):2344-2353 e7. [PubMed ID: 33836179]. https://doi.org/10.1016/j.jid.2021.02.757.
-
29.
Zhang W, Chen L, Xiong Y, Panayi AC, Abududilibaier A, Hu Y, et al. Antioxidant therapy and antioxidant-related bionanomaterials in diabetic wound healing. Front Bioeng Biotechnol. 2021;9:707479. [PubMed ID: 34249895]. [PubMed Central ID: PMC8264455]. https://doi.org/10.3389/fbioe.2021.707479.
-
30.
Samarghandian S, Farkhondeh T, Samini F, Borji A. Protective effects of carvacrol against oxidative stress induced by chronic stress in rat's brain, liver, and kidney. Biochem Res Int. 2016;2016:2645237. [PubMed ID: 26904286]. [PubMed Central ID: PMC4745576]. https://doi.org/10.1155/2016/2645237.
-
31.
Zhou Z, Liang S, Zhou Z, Liu J, Zhang J, Meng X, et al. TGF-beta1 promotes SCD1 expression via the PI3K-Akt-mTOR-SREBP1 signaling pathway in lung fibroblasts. Respir Res. 2023;24(1):8. [PubMed ID: 36627645]. [PubMed Central ID: PMC9832654]. https://doi.org/10.1186/s12931-023-02313-9.
-
32.
Pan X, Chen Z, Huang R, Yao Y, Ma G. Transforming growth factor beta1 induces the expression of collagen type I by DNA methylation in cardiac fibroblasts. PLoS One. 2013;8(4). e60335. [PubMed ID: 23560091]. [PubMed Central ID: PMC3613378]. https://doi.org/10.1371/journal.pone.0060335.
-
33.
de Haan JJ, Bosch L, Borgman A, Bastemeijer M, Brans MAD, van de Weg SM, et al. Complement 5a receptor deficiency does not influence adverse cardiac remodeling after pressure-overload in mice. Sci Rep. 2017;7(1):17045. [PubMed ID: 29213128]. [PubMed Central ID: PMC5719022]. https://doi.org/10.1038/s41598-017-16957-3.
-
34.
Mashima R. Physiological roles of miR-155. Immunology. 2015;145(3):323-33. [PubMed ID: 25829072]. [PubMed Central ID: PMC4479532]. https://doi.org/10.1111/imm.12468.
-
35.
Eissa MG, Artlett CM. The MicroRNA MiR-155 is Essential in Fibrosis. Noncoding RNA. 2019;5(1). [PubMed ID: 30871125]. [PubMed Central ID: PMC6468348]. https://doi.org/10.3390/ncrna5010023.
-
36.
Yang L, Zheng Z, Zhou Q, Bai X, Fan L, Yang C, et al. miR-155 promotes cutaneous wound healing through enhanced keratinocytes migration by MMP-2. J Mol Histol. 2017;48(2):147-55. [PubMed ID: 28247149]. https://doi.org/10.1007/s10735-017-9713-8.