Abstract
Background:
Dendrimers are a new class of polymeric materials with the well-defined structure, composition and architecture. Owing to antibacterial properties of dendrimers, they can be used as alternative water and wastewater disinfection with the minimum adverse side effects.Objectives:
The aim of the present study was to evaluate the antibacterial activity of second generation poly propylene imine (PPI- G2) dendrimer on predominant bacteria in drinking water resources.Materials and Methods:
In this qualitative and interventional study, a total of 764 water samples were collected from rural areas in Qom Province, Iran, and bacteria were isolated and identified using standard procedures. Minimum inhibitory concentration (MIC) and minimum bactericidal concentration (MBC) against gram-positive and gram-negative bacteria were determined according to clinical and laboratory standards institute (CLSI) guideline. Standard discs were prepared using different concentrations of dendrimer on Mueller-Hinton agar plates.Results:
The most important isolated bacteria were Escherichia coli 37 (48.7%), Klebsiella oxytoca 12 (15.8%), Proteus mirabilis 3 (4%), Entrobacter aerogenes 8 (10.5%), Citrobacter freundii 4 (5.2%), Pseudomonas aeruginosa 5 (6.6%), Staphylococcus aureus 2 (2.6%), and Bacillus subtilis 5 (6.6%). Moreover, it was found that PPI-G2 showed more potent activity towards the gram-positive bacteria than the gram-negative ones.Conclusions:
The PPI-G2 dendrimer with end amine groups exhibited a positive impact on the removal of dominant bacterial isolated strains. Therefore, it is possible to use these nanodendrimers as a safe and effective material for water disinfection in the future.Keywords
1. Background
Removal of harmful microorganisms such as bacteria, fungi, yeasts and viruses have always been one of the main concerns for human health. Due to accelerating growth and global expansion of antimicrobial resistance, there is a need to develop new antimicrobial compounds and strategies (1). In this regards many natural and artificial compounds are used to kill microorganisms or inhibit their growth (2-4). With the advent of new technology, including nanotechnology, in health and environmental science, application of nanostructure materials to fight against harmful biological agents is growing rapidly (1). In recent years, nanoparticles of zinc, silver, titanium and dendrimers have been widely used in health and environmental application due to the antimicrobial properties of these nanoparticles (5). Methods of evaluating antimicrobial activity of nanostructure materials can be divided into quantitative and qualitative methods. Microorganisms are often evaluated in the laboratory using the methods such as Minimum Inhibitory Concentration (MIC), Minimum Bactericidal Concentration (MBC), disc diffusion, colony counting, turbidity assay and dilution methods (6-12).
Dendrimers are macromolecules of well-defined structure and nano scale size characterized by a branched structure from the center to the periphery. Polypropylene imine is one of the oldest known dendrimer types developed initially by Jevprasesphant et al. (13) These dendrimers are made of central core and multiple activated agents.
Dendrimers have unique structural properties influenced by highly branched structure, multiple surface functional groups and the empty spaces between branches. These empty spaces are capable of absorbing guest molecules and encapsulating different-sized particles. For this reason, in recent years, scientists have studied application of dendrimers in the field of medicine, biology and pharmacy (14).
If the surface groups of dendrimers are functionalized with antimicrobial agents, they can be used for microbial deactivation. Lopez et al showed that Polyamidoamine (PAMAM) dendrimers with amino surface groups possess antibacterial activity against Escherichia coli, Pseudomonas aeroginosa and Staphylococcus aureus (15, 16). Influence of quaternary ammonium compounds in destruction and degradation of the cell wall of bacteria has been reported (17, 18). Most dendrimer antimicrobial agents are derived from activated surface groups with quaternary ammonium salts synthesis (19). Polypropylene imine dendrimers are constructed from D-amino butane core, internal divisions of triple propylene amine units and end amine groups (20). Therefore, the end amino group in their structure is the cause of their microbicidal properties (20). Antimicrobial activity of dendrimers is related to some key factors such as dendrimer core type, dendrimer surface charge, their functional groups, dendrimer three-dimensional structure and its size (21). However, it is recognized that if site effects of dendrimers are cell walls or cell membranes, the anti-life stabilized on the dendrimers can be more effective (16).
In these studies, the target cell for antimicrobial agent should be chosen carefully because the voluminous dendrimers cannot penetrate cell membrane barrier and cannot reach to site effect for antimicrobial activity (22). For example, Lopez et al. declared that the antimicrobial properties of Poly (amidoamine) dendrimer generation 3 is much greater than Poly (amidoamine) dendrimer generation 5 (16). However, mostly dendrimers antimicrobial activity is on the pathogenic microorganisms such as E. coli, S. aureus, P. aeroginosa, Listeria monocytogenes, and Streptococcus mutans. These microorganisms are responsible for various infections in humans and a major threat for public health (1). Thus, with attention to antimicrobial properties of dendrimers, the aim of this study was to evaluate the antimicrobial properties of PPI-G2 using disk diffusion, minimum inhibitory concentration (MIC) and minimum bactericidal concentration (MBC) methods and ultimately to use that as a disinfectant for removing isolated bacteria from contaminated rural drinking water samples in Qom province.
2. Objectives
With regard to the production of hazardous byproducts resulting from the indiscriminate use of disinfectants in drinking water treatment, the aim of this study was to evaluate the antibacterial properties of dendrimers in removing bacteria from drinking water.
3. Materials and Methods
In this qualitative and interventional study, sampling was performed during the period of April 2012 to March 2013 from different rural areas of Qom Province. Qom Province is one of the 31 provinces of Iran with 11,526 km², covering 0.7% of the total area in Iran. It is approximately in the center of the country, and west of Namak Lake. A total of 764 water samples from wells and springs at 116 different resources from different rural areas on different seasons were prepared. To increase the precision and accuracy of testing, sampling from some sources was repeated several times. Water samples were collected in 250 mL sterile glass bottles and immediately transferred in a cold box at 4°C to the laboratory. To neutralize residual chlorine in water 0.1 mL of a 10% solution of sodium thiosulphate was added to water. Microbiological analyses were carried out within 24 hours of sampling. Microbiological testing was performed using the membrane filter technique and bacteria were isolated and identified based on standard procedures (23).
3.1. Preparation of Antimicrobial Agents and Target Bacteria
Antimicrobial agent used for this study was the PPI-G2 dendrimer. This nanopolymer was purchased from SyMO-Chem Company, Netherlands. Table 1 shows the characteristics of this dendrimer.
Chemical Properties of Polypropylene Imine-G2 (PPI-G2) Dendrimer
Total Number of Amine Groups | Number of Amine End Groups | Density, g/cm3 | Molecular Weight, g/mol | Dendrimer Generation | Molecular Formula |
---|---|---|---|---|---|
14 | 08 | 0.98 | 770 | 02 | C40H94N14 |
Before use, all bacteria were incubated in nutrient broth culture under aerobic conditions at 37°C for 24 hours. In subsequent tests, optimal bacteria concentrations were adjusted according to 0.5 McFarland standards.
3.2. Determination of Antimicrobial Activity of Dendrimer
In order to determine the antimicrobial activity of dendrimer, we made serial dilution (500, 50, 5, 0.5, and 0.05 µg/mL) of antimicrobial agent using sterile distilled water. Also, Minimum Inhibitory Concentration, and Minimum Bactericidal Concentration of dendrimers against the target culture bacteria were determined using the disc diffusion method according to Clinical and Laboratory Standards Institute guideline (24).
Briefly, the antimicrobial activity of dendrimer was carried out using the disc diffusion method (25). In this method, 25 µL of antibacterial agent in different concentrations was added to standard blank disc (6 mm in diameter) and placed on the Mueller Hinton agar seeded with the target culture and incubated at 37°C for 24 hours.
3.3. Determination of Minimum Inhibitory Concentration and Minimum Bactericidal Concentration
In this investigation, we measured MIC and MBC using the method recommended by CLSI (24). Tubes containing 10 mL of nutrient broth (consisting of 108 CFU/mL of bacteria and dilution 0, 500, 50, 5, 0.5, and 0.05 µg/mL of dendrimer) incubated at 37°C for 24 hour. A Tube with the lowest concentration of antimicrobial agent and no bacterial growth was reported as MIC. To determine MBC, a loop of each tube with no growth transferred to Nutrient Agar plates and incubated at 37°C for 24 hours. A plate with no bacterial growth was reported as MBC. All experiments were repeated three times.
3.4. Evaluation of the Antimicrobial Activity of Dendrimer Against Bacteria in Water Samples
First of all, water was sterilized in an autoclave at 121°C for 15 minutes. Next, bacterial culture (103 CFU/mL) was diluted by adding 250 mL of sterile water. Different dilutions of antimicrobial agent were added to the water sample, and then bacteria were cultured in Nutrient Agar to observe the effects of the antimicrobial agents in various times. After 24 hours incubation at 37°C, colonies were counted.
4. Results
The data in Table 2 demonstrates the type and frequency of isolated bacteria. From a total of 764 water samples tested, 76 samples had bacterial contamination and 693 samples had no contamination. Of contaminated samples only 37 (48.7%) samples were found thermotolerant coliform bacteria. Other types of bacteria isolated were Klebsiella oxytoca 12 (15.8%), Proteus mirabilis 3 (4%), Entrobacter aerogenes 8 (10.5%), Citrobacter freundii 4 (5.2%), P. aeruginosa 5 (6.6%), S. aureus 2 (2.6%), Bacillus subtilis 5 (6.6%).
Frequency of Species Isolated From Water Sources
Isolated Species | ||||||||
---|---|---|---|---|---|---|---|---|
E. coli | B. subtilis | S. aureus | P. mirabilis | K. oxytoca | E. aerogenes | C. freundii | P. aeruginosa | |
Frequency, No. | 37 | 05 | 02 | 03 | 12 | 08 | 04 | 05 |
Scanning electron microscopy image of PPI-G2 and Fourier Transform Infrared Spectroscopy (FTIR) are presented in Figure 1 and Figure 2, respectively. The data in Table 3 shows the corresponding functional groups of the wavelengths indicated on Figure 2.
Scanning of Electron Microscopy Image of the Polypropylene Imine-G2 (PPI-G2)
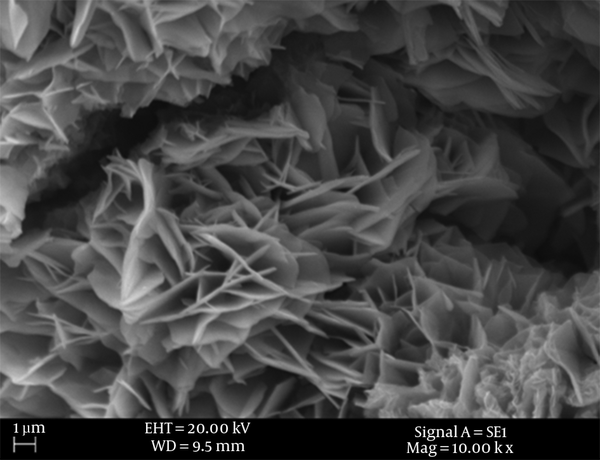
Fourier Transforms Infrared Spectroscopy Fourier Transforms Infrared Spectroscopy (FTIR) of Polypropylene Imine-G2 (PPI-G2)
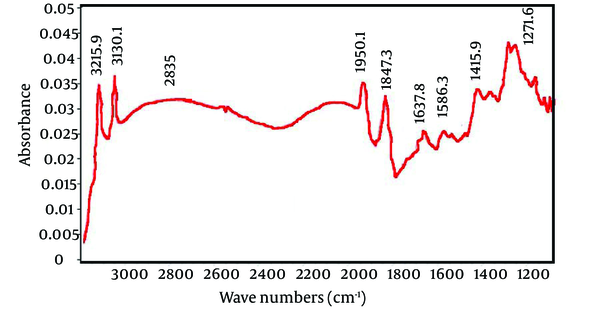
Polypropylene Imine-G2 (PPI-G2) Functionalized Groups
Wave Numbers, cm-1 | Functional Groups |
---|---|
3215 and 3130 | N-H stretching vibrations |
2835 | Amid I vibrations |
1950 and 1847 | Amid two vibrations |
1637 and 1586 | C-H stretching vibrations |
1415 and 1271 | C-H twisting vibrations |
At this stage, antimicrobial activity of PPI-G2 was evaluated. Table 4 shows the antibacterial effect of PPI-G2. Moreover, PPI-G2 showed a potent activity against gram-positive bacteria particularly B. subtilis.
The Mean Diameter of Inhibition Zone in Disk Diffusion Method Against Different Concentrations of Polypropylene Imine-G2 (PPI-G2) Dendrimer
Dendrimer concentration, µg/ml | Zone of inhibition, mm | |||
---|---|---|---|---|
E. coli | P. mirabilis | S. aureus | B. subtilis | |
500 | 20 | 18 | 25 | 28 |
50 | 12 | 11 | 17 | 19 |
05 | 08 | 08 | 10 | 12 |
0.5 | 0.0 | 0.0 | 0.0 | 08 |
0.05 | 0.0 | 0.0 | 0.0 | 0.0 |
Table 5 compares MIC and MBC values of Polypropylene Imine-G2 in different bacteria. As can be seen from this table, MIC value for E. coli and P. mirabilis was 50 µg/mL, and for B. subtilis and S. aureus was 5 µg/mL. Moreover, MBC value for E. coli and P. mirabilis was 500 µg/mL, and for B. subtilis and S. aureus was 50 µg/mL. Table 6 shows the antimicrobial activity (concentrations 500-0.5 µg/mL) of the PPI-G2 dendrimer in aqueous area on different isolated species.
Minimum Inhibitory Concentration and Minimum Bactericidal Concentration of the Polypropylene Imine-G2 (PPI-G2) Dendrimer
Dendrimer concentration, µg/ml | Type of Effect | |||
---|---|---|---|---|
Escherichia coli | Proteus mirabilis | Staphylococcus aureus | Bacillus subtilis | |
500 | MBC | MBC | MBC | MBC |
50 | MIC | MIC | MBC | MBC |
5 | Growth | Growth | MIC | MIC |
0.5 | Growth | Growth | Growth | Growth |
0.05 | Growth | Growth | Growth | Growth |
Changes in the Number of Bacteria Exposed to Different Concentrations of the Polypropylene Imine-G2 (PPI-G2) Dendrimer in Aquatic Environment
Type of Bacteria and Dendrimer Concentration, μg/mL | Exposure Time, min | ||||||
---|---|---|---|---|---|---|---|
0 | 10 | 20 | 30 | 40 | 50 | 60 | |
Proteus mirabilis | |||||||
500 | 1000 | 250 | 100 | 0 | 0 | 0 | 0 |
50 | 1000 | 280 | 100 | 50 | 0 | 0 | 0 |
5 | 1000 | 750 | 600 | 250 | 250 | 250 | 250 |
0.5 | 1000 | 1000 | 1000 | 1000 | 1000 | 1000 | 1000 |
Escherichia coli | |||||||
500 | 1000 | 180 | 50 | 0 | 0 | 0 | 0 |
50 | 1000 | 250 | 100 | 30 | 0 | 0 | 0 |
5 | 1000 | 700 | 600 | 250 | 200 | 200 | 200 |
0.5 | 1000 | 1000 | 1000 | 1000 | 1000 | 1000 | 1000 |
Staphylococcus aureus | |||||||
500 | 1000 | 100 | 0 | 0 | 0 | 0 | 0 |
50 | 1000 | 180 | 50 | 0 | 0 | 0 | 0 |
5 | 1000 | 680 | 500 | 200 | 50 | 0 | 0 |
0.5 | 1000 | 1000 | 1000 | 900 | 750 | 620 | 620 |
Bacillus subtilis | |||||||
500 | 1000 | 70 | 0 | 0 | 0 | 0 | 0 |
50 | 1000 | 150 | 50 | 0 | 0 | 0 | 0 |
5 | 1000 | 650 | 450 | 180 | 50 | 0 | 0 |
0.5 | 1000 | 1000 | 1000 | 800 | 700 | 500 | 500 |
As seen in Table 6, PPI-G2 has better antibiotic activity against Gram-positive than against Gram-negative bacteria. In addition, data obtained from the antimicrobial activity of the PPI-G2 dendrimer in aqueous area on different isolated species was evaluated in terms of reaction kinetic with the first degree equation kinetic (Figure 3). Table 7 presents the reaction kinetic for different types of bacteria tested.
First Reaction Rate Constants and Coefficients of Determination Disinfection Process Using the Polypropylene Imine-G2 (PPI-G2) Dendrimer
Type of Bacteria | ||||
---|---|---|---|---|
P. mirabilis | E. coli | S. aureus | B. subtilis | |
Coefficient of determination Reaction rate constant (min-1) | 0.98 | 0.99 | 0.90 | 0.93 |
Reaction rate constant, min-1 | 0.100 | 0.114 | 0.072 | 0.073 |
First Order Kinetic of Disinfection Process Using Polypropylene Imine-G2 (PPI-G2) Dendrimer
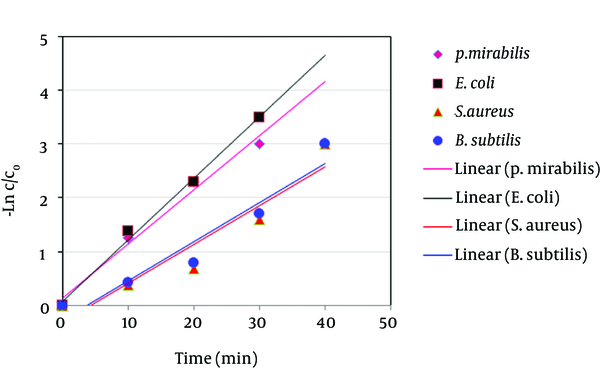
5. Discussion
As mentioned above, a total of 764 samples were tested, 71 samples had coliform (9.3%) and 693 (90.7%) samples had no coliform contamination. Positive samples in probability stage were transferred to the confirmatory stage. Only 37 (5%) samples tested for thermotolerant coliform bacteria were contaminated and other samples were not contaminated. Similar results were observed by Bay et al. (26); they reported that from a total of 598 water samples tested, 92.2% were not contaminated with bacteria, 7.8% were contaminated with coliform and 2.7% had thermotolerant coliform bacteria. According to Table 2, the frequency of E. coli, due to its intestinal source, is indicative of the extent of dispersion of those bacteria in the environment. Enterobacteriaceae family living naturally in the intestines of humans and animals, B. subtilis which are abundant in the soil and other saprophytic heterotrophic bacteria among isolates indicate that the water may be contaminated with different sources.
Figure 1 shows a scanning electron microscopy image of dendrimer. Because of their multi-layered structures with high purity, they can trap and absorb microbial agents. Dendritic structures known as dendriform with progressive structure are illustrated in this figure. Too many branches of this dendriform lead to increase the dendrimer specific surface and therefore they absorb microbes on their surface. On the other hand, nano holes created between branches trap biological agents and destroy them.
Figure 2 shows the FTIR spectroscopy of dendrimers. As seen, 5 peaks are detectable at 1271 cm-1, 1415 cm-1, 1586 cm-1, 1637 cm-1, 1847 cm-1, 1950 cm-1, 2835 cm-1 3130 cm-1 and 3215 cm-1 which the last peak is related to N-H stretching vibration. Other functional groups, based on wavelength, are presented in Table 3.
The size of the inhibition zone clearly shows that with increasing concentration of the antimicrobial agent, the zone surrounding the disks is expanded. This situation indicates the power of antimicrobial agent in higher concentrations. It can be deduced from the results that the size of the inhibition zone varies in different concentrations of antimicrobial agent. The least concentration of dendrimer that is bacteriostatic or bacteriocide (Table 5) suggested that PPI-G2 has antimicrobial properties and can be used as an antimicrobial agent. Previous studies have shown that antimicrobial agents damage bacteria by membrane damage, spatial deformation, degradation of bacterial enzymes, damage of chromosome and bacteria cell wall damage (27).
This character refers to end amine groups in dendrimer structure which interact with negative charge of membrane or cytoplasm microorganism causes bacterial cell wall damage and finally, inhabitation of bacterial activity (21). However, the PPI-G2 dendrimer is an efficient antibacterial agent against both Gram-negative and Gram-positive bacteria. Our results are also consistent with Chen et al. study. They observed the antimicrobial effect of polypropylene imine dendrimer modified with quaternary ammonium groups on Gram-positive and Gram-negative bacteria (22). It is obvious that Gram-positive bacteria show greater sensitivity than Gram-negative bacteria in exposure to antimicrobial properties of dendrimers. For example, according to Table 5, the MIC of PPI-G2 related to gram-positive bacteria (B. subtilis and S. aureus) is 5µg/ml and the MIC of PPI-G2 related to gram-negative bacteria (P. mirabilis and E. coli) is 50 µg/ml. The results of this study showed that with increasing concentration of the PPI-G2, an inhibition zone diameter was increased.
The major difference between Gram-positive and Gram-negative bacteria is in cell walls and amount of peptidoglycan in them. Because of peptidoglycan in Gram-positive bacteria is thicker than Gram-negative bacteria (23), it can be expected that they were more resistant to antimicrobial agents (20). Our results indicated that Gram-positive bacteria are more susceptible that Gram-negative ones. Salimpour et al. declared that PPI dendrimers have a good antimicrobial activity against microorganisms with no extracellular fatty acid (19). Selahattin et al. findings revealed that Gram-positive bacteria are more sensitive than Gram-negative ones because of differences in the cell wall structure, cell physiology and cell metabolism between the two groups (28). Neu declared that antimicrobial activity of dendrimers is because of their ability to increase membrane permeability, which eventually higher concentrations of dendrimers lead to complete breakdown of membrane and death of bacteria. Additionally, the binding between dendrimer and surface charge of bacterial cell is induced by electrostatic bonds between bacterial negative surface charge and dendrimer positive charge (14).
In Table 6, the removal of bacteria in expose to different concentrations of dendrimers is compared. As seen in Table 6, by increasing the time in all amounts of dendrimers, removing bacteria shows an upward trend. What can be inferred from the results of inhibition zone, MIC and MBC is that sensitivity of gram-positive bacteria is greater than gram-negative bacteria. In the lowest concentration of the PPI-G2 (0.5 µg/mL) even after one hour of exposure, no decline in the number of E. coli and P. mirabilis bacteria was found. But at the same conditions, after an hour of exposure, S. aureus and B. subtilis declined by 38% and 50%, respectively. In other concentrations of dendrimers, the PPI-G2 reduced the number of bacteria so that in concentration of 500µg/ml, after ten minutes of contact, the efficiency rates for reducing the number of E. coli bacteria, P. mirabilis, S. aureus and B. subtilis were 75%, 82%, 90% and 93%, respectively.
Figure 3 was obtained based on data derived from the effect of PPI-G2 on E. coli, P. mirabilis, S. aureus and B. subtilis. As Figure 3 indicates, the ratio of C/C0 (C = remaining bacteria, C0 = initial number of bacteria) plotted on a logarithmic scale against time give approximately a straight line graph. As indicated in Table 7, coefficient of determination (R2) for removal of E. coli, P. mirabilis, S. aureus and B. subtilis is 0.99, 0.98, 0.90, and 0.90, respectively. Thus, we can conclude that removal rates of bacteria by dendrimer fit linear equation. These results are consistent with other researchers’ findings. Alikhani et al. observed first order kinetics for removal of E. coli from water by ultraviolet radiation (29). Also, Miranzadeh et al. showed that coliform bacteria removal from polluted water by silver nanoparticles can be modeled as a first-order reaction (30).
Acknowledgements
References
-
1.
Moritz M, Geszke-Moritz M. The newest achievements in synthesis, immobilization and practical applications of antibacterial nanoparticles. Chem Eng J. 2013;228:596-613. https://doi.org/10.1016/j.cej.2013.05.046.
-
2.
Drakopoulou S, Terzakis S, Fountoulakis MS, Mantzavinos D, Manios T. Ultrasound-induced inactivation of gram-negative and gram-positive bacteria in secondary treated municipal wastewater. Ultrason Sonochem. 2009;16(5):629-34. [PubMed ID: 19131265]. https://doi.org/10.1016/j.ultsonch.2008.11.011.
-
3.
Alp S. [Bacterial resistance to antiseptics and disinfectants]. Mikrobiyol Bul. 2007;41(1):155-61. [PubMed ID: 17427567].
-
4.
Cerf O, Carpentier B, Sanders P. Tests for determining in-use concentrations of antibiotics and disinfectants are based on entirely different concepts: "resistance" has different meanings. Int J Food Microbiol. 2010;136(3):247-54. [PubMed ID: 19853944]. https://doi.org/10.1016/j.ijfoodmicro.2009.10.002.
-
5.
Albrecht MA, Evans CW, Raston CL. Green chemistry and the health implications of nanoparticles. Green Chem. 2006;8(5):417. https://doi.org/10.1039/b517131h.
-
6.
Wang XF, Zhang SL, Zhu LY, Xie SY, Dong Z, Wang Y, et al. Enhancement of antibacterial activity of tilmicosin against Staphylococcus aureus by solid lipid nanoparticles in vitro and in vivo. Vet J. 2012;191(1):115-20. [PubMed ID: 21900026]. https://doi.org/10.1016/j.tvjl.2010.11.019.
-
7.
Abbasi AR, Akhbari K, Morsali A. Dense coating of surface mounted CuBTC Metal-Organic Framework nanostructures on silk fibers, prepared by layer-by-layer method under ultrasound irradiation with antibacterial activity. Ultrason Sonochem. 2012;19(4):846-52. [PubMed ID: 22204978]. https://doi.org/10.1016/j.ultsonch.2011.11.016.
-
8.
Tayel AA, El-Tras WF, Moussa S, El-Baz AF, Mahrous H, Salem MF, et al. Antibacterial Action of Zinc Oxide Nanoparticles against Foodborne Pathogens. J Food Saf. 2011;31(2):211-8. https://doi.org/10.1111/j.1745-4565.2010.00287.x.
-
9.
Nair MG, Nirmala M, Rekha K, Anukaliani A. Structural, optical, photo catalytic and antibacterial activity of ZnO and Co doped ZnO nanoparticles. Mater Lett. 2011;65(12):1797-800. https://doi.org/10.1016/j.matlet.2011.03.079.
-
10.
Pinto RJ, Fernandes SC, Freire CS, Sadocco P, Causio J, Neto CP, et al. Antibacterial activity of optically transparent nanocomposite films based on chitosan or its derivatives and silver nanoparticles. Carbohydr Res. 2012;348:77-83. [PubMed ID: 22154478]. https://doi.org/10.1016/j.carres.2011.11.009.
-
11.
Raghupathi KR, Koodali RT, Manna AC. Size-dependent bacterial growth inhibition and mechanism of antibacterial activity of zinc oxide nanoparticles. Langmuir. 2011;27(7):4020-8. [PubMed ID: 21401066]. https://doi.org/10.1021/la104825u.
-
12.
Kalantar E, Motlagh M, Lordnejad H, Beiranvand S. The prevalence of bacteria isolated from blood cultures of iranian children and study of their antimicrobial susceptibilities. Jundishapur J Nat Pharm Prod. 2008;3(1):1-7.
-
13.
Jevprasesphant R. The influence of surface modification on the cytotoxicity of PAMAM dendrimers. Int J Pharm. 2003;252(1-2):263-6. https://doi.org/10.1016/s0378-5173(02)00623-3.
-
14.
Neu HC. The crisis in antibiotic resistance. Science. 1992;257(5073):1064-73. [PubMed ID: 1509257].
-
15.
Calabretta MK, Kumar A, McDermott AM, Cai C. Antibacterial activities of poly(amidoamine) dendrimers terminated with amino and poly(ethylene glycol) groups. Biomacromolecules. 2007;8(6):1807-11. [PubMed ID: 17511499]. https://doi.org/10.1021/bm0701088.
-
16.
Lopez AI, Reins RY, McDermott AM, Trautner BW, Cai C. Antibacterial activity and cytotoxicity of PEGylated poly(amidoamine) dendrimers. Mol Biosyst. 2009;5(10):1148-56. [PubMed ID: 19756304]. https://doi.org/10.1039/b904746h.
-
17.
McBain AJ, Ledder RG, Moore LE, Catrenich CE, Gilbert P. Effects of quaternary-ammonium-based formulations on bacterial community dynamics and antimicrobial susceptibility. Appl Environ Microbiol. 2004;70(6):3449-56. [PubMed ID: 15184143]. https://doi.org/10.1128/AEM.70.6.3449-3456.2004.
-
18.
Kourai H, Horie T, Takeichi K, Shibasaki I. The antimicrobial characteristics of quaternary ammonium salts and their alkyl chain length. J Antibacterial Antifungal Agents. 1980;8(5):9-17.
-
19.
Chen CZ, Beck-Tan NC, Dhurjati P, van Dyk TK, LaRossa RA, Cooper SL. Quaternary ammonium functionalized poly(propylene imine) dendrimers as effective antimicrobials: structure-activity studies. Biomacromolecules. 2000;1(3):473-80. [PubMed ID: 11710139].
-
20.
Salimpour Abkenar S, Malek RMA, Taher S. Effect of poly (propylene imine) dendrimer nano structure on antimicrobial property of cotton fabric. Pejouhesh. 2012;36(1):11-8.
-
21.
Wang B, Navath RS, Menjoge AR, Balakrishnan B, Bellair R, Dai H, et al. Inhibition of bacterial growth and intramniotic infection in a guinea pig model of chorioamnionitis using PAMAM dendrimers. Int J Pharm. 2010;395(1-2):298-308. [PubMed ID: 20580797]. https://doi.org/10.1016/j.ijpharm.2010.05.030.
-
22.
Chen CZ, Cooper SL. Recent advances in antimicrobial dendrimers. Adv Mater. 2000;12(11):843-6. https://doi.org/10.1002/(SICI)1521-4095(200006)12:11<843::AID-ADMA843>3.3.CO;2-K.
-
23.
Murray P, Rosenthal K PM. Med Microbiol. Philadelphia: Mosby Elsevier; 2009.
-
24.
Wikler MA. Performance Standards for Antimicrobial Susceptibility Testing: Twentieth Informational Supplement. Clinical and Laboratory Standards Institute; 2010.
-
25.
Dabiri H, Aghamohammad S, Goudarzi H, Noori M, Hedayati MA, Ghoreyshiamiri SM. Prevalence and Antibiotic Susceptibility of Campylobacter species Isolated From Chicken and Beef Meat. Int J Enteric Pathog. 2014;2(2). eee17087.
-
26.
Bay A, Poorshamsian K, Karimi K, Hashemi M, Maghsodlo B. Determination of Bacteriological and Physiochemical Parameters of Drinking Water of Gorgan city, Iran. Med Lab J. 2011;5(1):13-7.
-
27.
Hebeish A, Hashem M, Abdel-Rahman A, El-Hilw ZH. Improving easy care nonformaldehyde finishing performance using polycarboxylic acids via precationization of cotton fabric. J Appl Polym Sci. 2006;100(4):2697-704. https://doi.org/10.1002/app.22916.
-
28.
Selahattin A, Kadri. G, Ramazan C. Effect of zinc on microbial growth. Tr J Med Sci. 1998;28:595-7.
-
29.
Alikhani MY, Khorasani M, Piri Dogahe H, Shirzad Siboni M. Investigation of Efficiency Ultra Violet Radiation in Disinfection of Escherichia coli in Aquatic Environments: Kinetic Study. J Ardabil Univ Med Sci. 2011;11(2):158-65.
-
30.
Miranzadeh MB, Rabbani D, Naseri S, Nabizadeh R, Mousavi SGA, Ghadami F. Coliform bacteria removal from contaminated water using nanosilver. Feyz J Kashan Univ Med Sci. 2012;16(1).