Abstract
Background:
Hymenodictyon excelsum is a medicinal plant traditionally used for tumor treatment as it contains phytochemicals of anthraquinone and coumarin class.Objectives:
The aim of the present study was to unfold the therapeutic value of selected phytocompounds of Hymenodictyon excelsum in prostate cancer.Materials and Methods:
Eight phytochemicals were selected based on the literature search including anthragallol, damnacanthal, esculin, lucidin, morindone, nordamnacanthal, rubiadin, and soranjidiol while dihydrotestosterone was considered as the control. Human androgen receptor (AR) ligand binding domain (PDB id: 1e3g) was selected as the receptor for subsequent computational docking study. First, the selected phytocompounds were screened for their drug likeness and safety profile. Molegro Virtual Docker (MVD) was subjected only to drug-like and safe phytocompounds for the computational docking study.Results:
Except for anthragallol, nordamnacanthal and rubiadin, all the ligands were drug-like and safe. Results of the docking study suggest a favorable binding of esculin to the receptor with respect to dihydrotestosterone. Analysis of docking pattern revealed a nearly similar ligand-receptor interaction for both dihydrotestosterone and esculin.Conclusions:
The anthraquinone and coumarin principles of H. excelsum have an anti-prostate cancer effect that has been proposed to be exerted by antagonistic effects on AR.Keywords
1. Background
Androgens are a class of natural or synthetic steroid hormone compounds such as testosterone, dihydrotestosterone and androstenedione (1). Physiological androgens play vital roles in the regulation of anabolic metabolism and expression of male phenotype. They are actually eminent for regulation of male development particularly the male reproductive system (2). The diverse effects of androgens are mediated by complex cell type-specific signaling pathways that always involve the androgen receptor (AR) (3). The androgen receptor (AR) is a type of nuclear receptor that acts as a DNA-binding transcription factor to regulate gene expression (4). Dysregulation of androgen/AR signaling perturbs normal reproductive development and accounts for a wide range of pathological conditions such as prostate cancer (2). Recent experimental findings conform the expression of AR in androgen-dependent, androgen-independent or hormone refractory prostate cancers, where AR expression is maintained throughout prostate cancer progression (5). Interestingly, a number of clinical studies suggest that prostate cancer initiation and progression is also uniquely dependent on AR expression (6). This pathogenic role of AR has made it a major therapeutic target in aggressive prostate cancer (7). Therefore, searching for AR inhibitors is clearly an important issue for biological research.
Hymenodictyon excelsum is a medium-sized deciduous tree of the Rubiaceae family. It is locally known as “Phuti-kadam” and has an abundant distribution in Bangladesh and India. In traditional medicinal practice the bark of H. excelsumis used as an astringent and febrifuge and for treatment of fever and tumors, while the leaves are used to treat ulcers, sialitis, sore throat, tonsillitis and inflammatory conditions (8). The stem bark of H. excelsum contains tannin, toxic alkaloid, hymenodictine, esculin, an apioglucoside of scopoletin andhymexelsin (9). Anthraquinones, rubiadin and its methyl ether, lucidin, nordamnacanthal, damnacanthal, 2-benzylzanthopurpurin, anthragallol, soranjidiol and morindone have also been isolated from roots (10). So far, little is known about the pharmacological activity of this plant. The plant has been reported to have antioxidant and anti-inflammatory properties (11).
2. Objectives
The present study aimed to investigate H. excelsum’s phytochemicals with anti-prostate cancer activity and to predict their mechanisms of action using a computational molecular simulation study.
3. Materials and Methods
3.1. Selection of Receptor and Ligands
The therapeutic effects of a medicinal plant are attributed to the phytochemical constituents of that plant. Therefore, it is feasible to study individual phytochemicals present in plants when investigating the therapeutic effects of medicinal plants. From the literature search, eight phytochemicals were taken into consideration for the molecular simulation study including anthragallol, damnacanthal, esculin, lucidin, morindone, nordamnacanthal, rubiadin and soranjidiol. Besides, dihydrotestosterone was considered as the control. Canonical SMILES file of these selected ligand molecules were retrieved from the PubChem Compound server (https://pubchem.ncbi.nlm.nih.gov/). Canonical SMILES files were entered in to the Corina online demo to generate 3D Protein Data Bank (PDB) files. The human androgen receptor was the receptor used in the present study. Protein Data Bank (PDB) file of human AR ligand binding domain (PDB id: 1e3g) was downloaded from RCSB PDB database (http://www.rcsb.org/pdb/home/home.do).
3.2. Drug likeness and Toxicity Evaluation of the Ligands
Prior to molecular simulation study the drug likeness and safety of the chosen ligands were evolved by empirical computational tools. The Molinspiration cheminformatics server was exploited to calculate the molecular properties associated with drug-likeness and to predict bioactivity of components including the nuclear receptor ligand and enzyme inhibitor (12). The OSIRIS property explorer was used to evolve the toxicity of the selected ligands in term of mutagenic, tumorigenic and irritant properties (13). Only safe drug-like phytochemicals were subjected to the molecular simulation study.
3.3. Computational Simulation Study
3.3.1. Binding Site Prediction
The site of the receptor that interacted with the ligands was predicted by the Molegro Virtual Docker (MDV) inbuilt cavity detection algorithm and the Pocket-Finder server (14). The outputs from both of the computational tools were synchronized to predict the ultimate ligand binding site and exploited in the subsequent docking study.
3.3.2. Computational Simulation Tools and Algorithm
Molegro Virtual Docker (MVD) was used for the computer simulated docking study (15). This tool performs flexible ligand docking with the optimization of ligand geometry during docking. Molegro Virtual Docker includes MolDock and PLANTS score for evaluating docking solutions followed by a rank for the best conformations. The MVD uses a differential evolution algorithm. The solution to the function is the sum of intermolecular interaction energy between protein and ligand with the intra-molecular interaction energy of the ligand. The docking energy scoring function is based on a modified piecewise linear potential (PLP) with new hydrogen bonding and electrostatics terms included. A lower score always indicates a higher affinity.
3.3.3. Molecular Docking Study
Before initiation of the docking operation, both protein and ligands were prepared by MVD through assigning bonds, bond orders, explicit hydrogens, charges and flexible torsions at the missing region only. Potential binding sites (cavities or active sites) were identified using the built-in cavity detection algorithm of MVD with a grid resolution (Å) of 0.30. Internal electrostatic interactions, internal hydrogen bonds, and Sp2-Sp2 torsions were selected as ligand evaluation terms. The “MolDock SE” was set as the searching algorithm for ten runs using a maximum of 1500 iterations with a total population size of 50. Energy minimization and optimized H-bonds were enabled as post-docking steps for more accurate refinement of the docking results. Multiple poses were clustered based on the RMSD threshold of 1.0, ignoring similar poses with RMSD threshold of 1.0.
4. Results
4.1 Result of Drug Likeness and Toxicity Evaluation of the Ligands
Result of the drug likeness and toxicity evaluation of the ligand have been summarized in Table 1. The results from the Molinspiration cheminformatics server showed that all the selected ligands met the Lipinski's rule of drug likeness without violation of any condition. Additionally, all the ligands fitted more or less with nuclear receptor ligands and enzyme inhibitors. The output from the OSIRIS property explorer suggested the esculin, lucidin, morindone and soranjidiol were safe ligands with no mutagenic or tumorigenic effects. Thus, the probable phytochemicals were filtered, as they were more potent physiologically active molecules without any harmless effects.
Drug Likeness and Toxicity Evaluation of the Selected Ligands a
Ligand | miLogP | TPSA | NV | NRL | EI | Mutagenic | Tumorigenic | Irritant |
---|---|---|---|---|---|---|---|---|
Anthragallol | 2.61 | 94.83 | 0 | 0.01 | 0.29 | Y | N | N |
Damnacanthal | 3.34 | 80.68 | 0 | 0.10 | 0.14 | N | N | Y |
Esculin | 3.34 | 80.68 | 0 | 0.10 | 0.14 | N | N | Y |
Lucidin | 2.61 | 94.83 | 0 | 0.19 | 0.38 | N | N | N |
Morindone | 3.04 | 94.83 | 0 | 0.07 | 0.20 | N | N | N |
Nordamnacanthal | 3.06 | 91.67 | 0 | 0.15 | 0.22 | Y | N | Y |
Rubiadin | 3.72 | 74.60 | 0 | 0.07 | 0.24 | Y | N | N |
Soranjidiol | 3.30 | 74.60 | 0 | 0.11 | 0.20 | N | N | N |
4.2. Computational Simulation Study
4.2.1. Binding Site Prediction
Initial prediction of the probable ligand-binding site was performed by the Pocket-Finder and MDV Cavity Search. The prediction showed an active site of 409 cubic angstroms with a maximum coordination of 8 × 42 × 12 from the Pocket-Finder output (Figure 1). The cavity searching algorithm built in the MVD tool fetched a cavity of 86.02 cubic angstroms with a maximum coordination of 0.41 × 31.67 × 4.44 and surface of 204.8 square angstroms. Output of the Pocket-Finder was synchronized with the MDV Cavity Search output for screening the prediction with higher degree of accuracy. As, these two outputs overlapped and the selected molecule had a surface of ~95 square angstroms at best, the cavity coordination of 0.41 × 31.67 × 4.44 was set as the desired ligand interaction site or binding site.
Predicted Binding Site by the Pocket-Finder
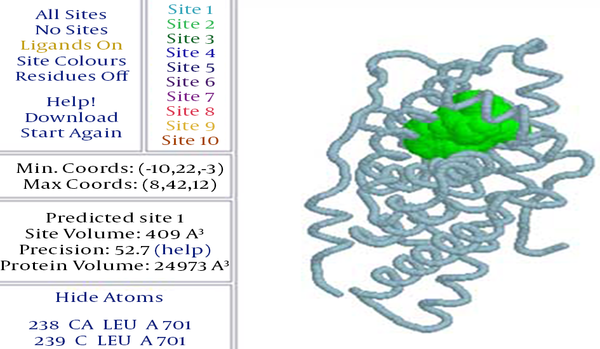
4.2.2. Computational Molecular Docking
The computational docking study of the ligand with the receptor (coordinate: 0.41 × 31.67 × 4.44) by MVD was evolved in terms of MolDock Score for best pose. Dihydrotestosterone exhibited the lowest docking score of -109.81. This observation is concordant with previous study with R 18-100 that has inhibitory effect on the AR. Among the studied ligands, esculin exhibited the most favorable binding to the receptor followed by morindone, anthragallol, soranjidiol, lucidin and damnacanthal. The MolDock Score also revealed that esculin’s interaction with the receptor is likely to be more energetically economic than that of the dihydrotestosterone (Table 2).
During dihydrotestosterone interaction with the receptor, the amino acid residues, including Gln 711, Arg 752 and Thr 877, were involved in hydrogen bonding while Gln 711, Met 745 and Phn 764 were involved in steric interaction (Figure 2A).
The amino acid residues Leu 704, Asn 705, Arg 752 and Thr 877 were involved in hydrogen bonding in case of esculin’s interaction receptor. Esculin exploited Leu 704, Gln 711, Leu 873, Phe 876, Met 787 and Met 895 residues at the binding site to maintain a steric interaction with the receptor (Figure 2 B). Thus, the amino acid residues Arg 752 and Thr 877 were the common residues involved in the hydrogen bonding of the receptor with esculin and dihydrotestosterone.
Molecular Docking Results from the Molegro Vertual Docker (MVD) for Ligands Bound to the Androgen Receptor
Ligands | MolDock Score (kcal/mol) | H-Bond (kcal/mol) | Steric Energy (kcal/mol) |
---|---|---|---|
Damnacanthal | -97.28 | -3.09 | -123.05 |
Lucidin | -98.13 | -3.62 | -113.96 |
Dihydrotestosterone | -109.81 | -4.62 | -115.65 |
Anthragallol | -99.35 | -3.61 | -106.28 |
Soranjidiol | -98.14 | -6.75 | -109.83 |
Morindone | -105.49 | -7.98 | -111.53 |
Esculin | -146.55 | -9.06 | -134.47 |
Docking Poses of the Ligand-Androgen Receptor
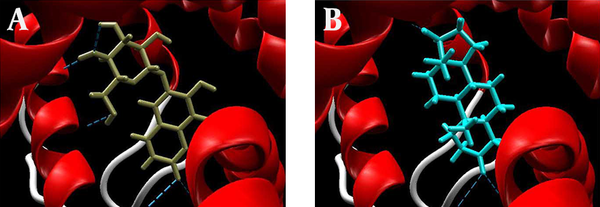
5. Discussion
The purpose of the present study was to evaluate potential phytochemicals with anti-prostate cancer activity from H. excelsum with prediction of mechanism of action using a computational molecular simulation study. This study firstly investigated the anti-prostate cancer effect of H. excelsum phytochemicals with a mechanistic insight.
From the literature search, eight phytochemicals were selected as the subject ligands including anthragallol, damnacanthal, esculin, lucidin, morindone, nordamnacanthal, rubiadin, and soranjidiol while dihydrotestosterone was considered as the control. Besides, human androgen receptor (AR) ligand binding domain was selected as the receptor for the subsequent computational docking study. All the ligands were screened for drug-likeness rules, biological activity and probable toxicity. Esculin, lucidin, morindone and soranjidiol were found as more potent physiologically active molecules without any harmless effects (Table 1).
Upon the generation of the 3D PDB structure, the ligands were docked to the AR ligand binding residues by Molegro Virtual Docker (MVD) tools. Results of the docking study suggest a favorable binding of esculin to the receptor with respect to dihydrotestosterone (Table 2). The literature search revealed that previous studies agree with the present findings. Several experimental data suggest the likelihood of esculin’s anti-cancer effects. The coumarin glycoside esculin also scavenges superoxide radicals and decreases lipid peroxidation that is considered as a common strategy of a broad range on anti-cancer agents (16). Cichorium intybus, an esculin containing plant, has been reported to have a modest inhibitory effects on the proliferation of prostate, breast and colorectal cells (17). Analysis of amino acid residues involved in docking, hydrogen bonding energy contribution to docking score and steric interaction revealed a nearly similar ligand-receptor interaction for both of dihydrotestosterone and esculin. Still, from the present molecular simulation study it was profound that the contribution of hydrogen bonding to MolDock score was higher for esculin with respect to dihydrotestosterone (Table 2). Thus, hydrogen bonding and steric interaction patterns of esculin and dihydrotestosterone suggested a nearly similar ligand-receptor interaction pattern. Therefore, esculin has the potency to act like an antagonist of dihydrotestosterone, while it also has a potential AR inhibitory effect.
References
-
1.
Sriram D, Yogeeswari P. Medicinal Chemistry. 2nd ed. New Delhi, India: Pearson Education; 2010.
-
2.
Matsumoto T, Sakari M, Okada M, Yokoyama A, Takahashi S, Kouzmenko A, et al. The androgen receptor in health and disease. Annu Rev Physiol. 2013;75:201-24. [PubMed ID: 23157556]. https://doi.org/10.1146/annurev-physiol-030212-183656.
-
3.
Gelmann EP. Molecular biology of the androgen receptor. J Clin Oncol. 2002;20(13):3001-15. [PubMed ID: 12089231].
-
4.
Heinlein CA, Chang C. The roles of androgen receptors and androgen-binding proteins in nongenomic androgen actions. Mol Endocrinol. 2002;16(10):2181-7. [PubMed ID: 12351684]. https://doi.org/10.1210/me.2002-0070.
-
5.
Heinlein CA, Chang C. Androgen receptor in prostate cancer. Endocr Rev. 2004;25(2):276-308. [PubMed ID: 15082523]. https://doi.org/10.1210/er.2002-0032.
-
6.
Lonergan PE, Tindall DJ. Androgen receptor signaling in prostate cancer development and progression. J Carcinog. 2011;10:20. [PubMed ID: 21886458]. https://doi.org/10.4103/1477-3163.83937.
-
7.
Lee E, Madar A, David G, Garabedian MJ, Dasgupta R, Logan SK. Inhibition of androgen receptor and beta-catenin activity in prostate cancer. Proc Natl Acad Sci U S A. 2013;110(39):15710-5. [PubMed ID: 24019458]. https://doi.org/10.1073/pnas.1218168110.
-
8.
Nareeboon P, Komkhunthot W, Lekcharoen D, Wetprasit N, Piriyapolsart C, Sutthivaiyakit S. Acetylenic fatty acids, triglyceride and triterpenes from the leaves of Hymenodictyon excelsum. Chem Pharm Bull (Tokyo). 2009;57(8):860-2. [PubMed ID: 19652413].
-
9.
Rao PS, Asheervadam Y, Khaleelullah M, Rao NS, Murray RD. Hymexelsin, an Apiose-Containing Scopoletin Glycoside from the Stem Bark of Hymenodictyon excelsum. J Nat Prod. 1988;51(5):959-61. [PubMed ID: 21401191]. https://doi.org/10.1021/np50059a024.
-
10.
Rastogi RP, Mehrotra BN. Compendium of Indian Medicinal Plants. 1st ed. New Delhi: Lucknow and Publicatios & Information Directorate;
-
11.
Kar B, Nepal A, Kumar RBS, Dolai N, Bhattacharya S, Mazumder UK, et al. Antioxidant and anti-inflammatory properties Hymenodictyon excelsum bark. Orient Pharm Exp Med. 2013;13(2):103–111.
-
12.
Available from: http://www.molinspiration.com/cgi-bin/properties.
-
13.
Available from: http://www.organic-chemistry.org/prog/peo/.
-
14.
Available from: http://www.modelling.leeds.ac.uk/pocketfinder/.
-
15.
Thomsen R, Christensen MH. MolDock: a new technique for high-accuracy molecular docking. J Med Chem. 2006;49(11):3315-21. [PubMed ID: 16722650]. https://doi.org/10.1021/jm051197e.
-
16.
Biljali S, Hadjimitova VA, Topashka-Ancheva MN, Momekova DB, Traykov TT, Karaivanova MH. Antioxidant and antiradical properties of esculin, and its effect in a model of epirubicin-induced bone marrow toxicity. Folia Med (Plovdiv). 2012;54(3):42-9. [PubMed ID: 23270206].
-
17.
Nawab A, Yunus M, Mahdi AA, Gupta S. Evaluation of anticancer properties of medicinal plants from the Indian sub-continent. Mol Cellular Pharmacol. 2011;3(1):21-9.