Abstract
Keywords
1. Background
Insulin instability has been regarded as a significant obstacle to developing an insulin oral dosage device to attain optimal diabetic control. Interest in using natural materials as a part of drug delivery protocols has increased in the past two decades (1). This characteristic has been used to produce sustained-release particulate systems for a variety of medications, proteins, and even cells (2, 3). The aim of this study was to prepare and characterize an insulin-loaded TMC/tragacanth polymeric delivery system. A drug carrier for insulin should provide a stable and biocompatible environment to ensure that the main fraction of the therapeutic protein will be biologically active following encapsulation (4).
Chitosan is a natural cationic polysaccharide macromolecule obtained by the alkaline deacetylation of the natural polysaccharide chitin (5). This compound is a hydrophilic, biocompatible, and biodegradable macromolecule, which is the most abundant polymer after cellulose. The interaction between this cationic compound and the anionic components of the glycoproteins on the epithelial cell surface on one side and the changes it induces in the cytoskeletal F-actin on another side can open the tight junction of the intestinal epithelium and increase the permeability of this delivered protein (6). Biopolymers, such as tragacanth, an anionic polysaccharide gum, can be alternative polymeric carriers for physiologically important peptides and proteins. Therefore, the characterization of tragacanth is essential for providing a foundation for possible applications.
The peptide/protein delivery mechanism of tragacanth can be achieved through two types of mechanisms: polyelectrolyte complexes (PECs) and entrapment through hydrogel (7, 8). Cell toxicity was tested using the L-929 cell line. Finally, orally administered NPs elicited a hypoglycemic response and increased serum insulin levels in diabetic rats (9).
2. Methods
2.1. Materials
Insulin (Isophane regular suspension USP recombinant DNA origin one vial of 10 mL, 100 units per mL) was purchased from Vitane Pharmed pharmaceutical company. Hydrochloric acid (HCl, 37%), Na2HPO4, KH2PO4, and H3PO4 were bought from Merck chemical company. Tragacanth was purchased from Tehran's local market. Chitosan powder (MW = 100,000 - 300,000 deacetylation degree ≥ 90%) was obtained from Acros Organics. An insulin enzyme-linked immunoassay (ELISA) kit was purchased from Monobind Inc (USA).
2.2. Synthesis of Trimethyl Chitosan/Insulin/Tragacanth
In order to prepare the composite of TMC/insulin/tragacanth, which contained 0.9% of insulin, the calculated amount of insulin (1 mL) was first added to 20 mL of TMC solution (1% wt. in acetic acid). Next, the solution was sonicated at ambient temperature for 30 min, followed by the addition of tragacanth (20 mL) and another 30 min of ultrasonic irradiation (10).
2.3. Synthesis of Insulin/Tragacanth
To prepare the composite of insulin/tragacanth with 1.65% of insulin, 1 mL of insulin was added to 20 mL of tragacanth solution (1% wt.), and this solution underwent 30 minutes of ultra-sonication.
2.4. Synthesis of Insulin/Trimethyl Chitosan
To prepare the composite of insulin/TMC, including 1.7% of insulin, 1 mL of insulin was added to 20 mL of TMC solution (1% wt), followed by 30 min of ultra-sonication.
3. Results
3.1. Morphology and Size of Particles
The physical properties of the microparticles were characterized by N2 sorption at -196°C using a TriStar 3000 gas sorption apparatus. The specific surface area was calculated from the isotherms (11) using the Brunauer-Emmet-Teller theory (BET) and the pore volume from the total adsorbed amount at a relative pressure of p/p0 0.97. The average pore diameter was estimated using the obtained values for the surface area and the total pore volume assuming the pores are cylindrical.
Dynamic light scattering analysis and zeta potential were conducted at neutral pH to measure the size and zeta potential of TMC/insulin/tragacanth and TMC/tragacanth composites (12). The measured size for TMC/insulin/tragacanth was 602 nm, while the size was 438 nm for the TMC/tragacanth composite. The size of the composite was considered to be suitable for oral delivery. Zeta potential values (Table 1) were calculated to be 34.7 and 6.28 mv for TMC/insulin/tragacanth and TMC/tragacanth, respectively, proving the remarkable increase in stability following drug loading. The composite surface charge was positive, both with and without the drug.
Textural Properties of CS/WST and CS/In/WST 0.86%
Sample | SBET [m2 g-1] | Vp [cm3 g-1] | Dp [nm] |
---|---|---|---|
CS/In/WST | 75.8736 | 0.062334 | 3.36 |
CS/WST | 69.8378 | 0.059483 | 3.44 |
3.2. In Vitro Release
An insulin ELISA kit was used to evaluate the entrapment efficiency (EE%) of loaded insulin, calculated to be 100%. Moreover, the ELISA results revealed that insulin, during the ELISA experiment, will be released from TMC/insulin/tragacanth binding to the insulin receptor (13). It is evident from the release data that both TMC and tragacanth tend to make strong interactions with insulin. Therefore, there is negligible release at both pH = 1.2 and 7.4. However, the comparison between release profiles of TMC/insulin/tragacanth, tragacanth/insulin, and TMC/insulin showed a considerable difference at pH = 7.4. The percentage of release was high as 90% over 12 h for TMC/insulin/tragacanth at pH = 7.4. Figure 1 can be explained by the deprotonation of both carboxyl groups available in tragacanth and TMC, and the repulsion between the polymer chains makes the release of insulin from TMC/insulin/tragacanth possible. Consequently, this system is applicable inside the body because when pH changes from 2 (stomach pH) to 7 (intestine pH), the insulin release will start (14, 15).
Insulin release profile from TMC/tragacanth in pH = 1.2 and 7.4
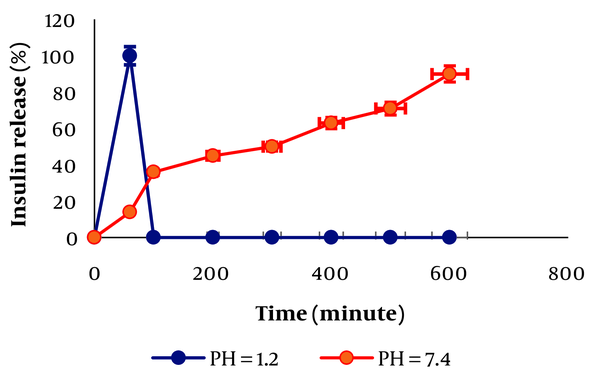
3.3. Real Amount of Insulin in Synthesized Samples
The insulin quantification test was performed using the Accubind ELISA kit (16) according to the protocol of the company (Monobind Inc, USA). In this kit, the absorbance was read at 450 nm, with 650 nm as a reference. The level of insulin (µIU/mL) uptake in the synthesized samples was measured in terms of percentage by concentrate (%w/w) of insulin loaded (Figure 2). These experiments were performed in duplicate, and the results were reported as mean ± standard deviation.
Insulin levels in each sample were measured using ELISA and were compared by
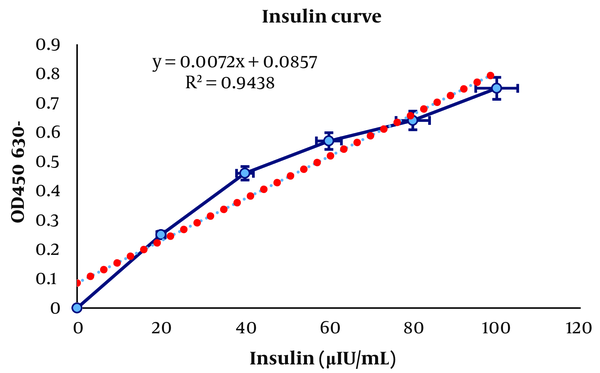
3.4. Cell Cytotoxicity and Cellular Uptake
In the toxicity test (17), the samples were made with different insulin doses (0.2, 0.4, 0.6, and 1 %w/w) and added to the medium in three volumes of 5, 10, and 20 µL. The number of cells per well was 10000, and the toxicity effects of the synthesized samples were compared in 24 and 48 h with those of the drug-free composite. As the diagrams in Figure 3A-B show, increasing the insulin dose from 0.2 to 1 raised the absorption rate, indicating that an increase in insulin concentration had no toxic effect on the cells implanted in the medium and their growth. Take a closer look at the lowest insulin concentration of 0.2, which shows that the composite has a very low toxic effect on the cells, offset by the high insulin dose in other samples.
MTT assay of L-929 cells with the blank composite (TMC/tragacanth) in different concentrations; A: 24 h; B: 48 h
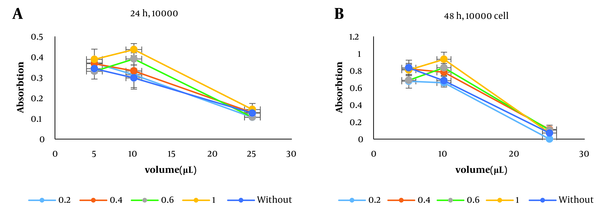
3.5. In Vivo Hypoglycemic Effect and Pharmacokinetics
In an animal study, 40 Wistar rats were selected, and after weighting, female mice were injected with diabetic mice (18). The mice were divided into 8 groups. The effects of the oral and parenteral administration of the synthesized sample compared to insulin solutions have been noted.
As shown in Figure 4, the synthesized sample of Ins-TMC/tragacanth had a slower release than the other samples, and its release results are almost in line with the results obtained from in vitro studies. It is well established that when the synthesized sample is administered orally, after about 10 h in the intestine, it can release insulin at a slower rate than other samples and causes lower blood glucose over a continuous period, making it less necessary to use insulin to lower blood glucose. The amount of insulin was also measured in the blood serum of the animals, with no corresponding curve. The highest level of insulin in the bloodstream is almost obtained when the blood glucose reaches the lowest level (Figure 4A and B).
Blood glucose levels in diabetic rats after the oral administration of Ins-TMC/tragacanth, insulin solution, and saline (A). Plasma insulin level in diabetic rats after the oral administration of Ins-TMC/tragacanth, insulin solution, and saline (B).
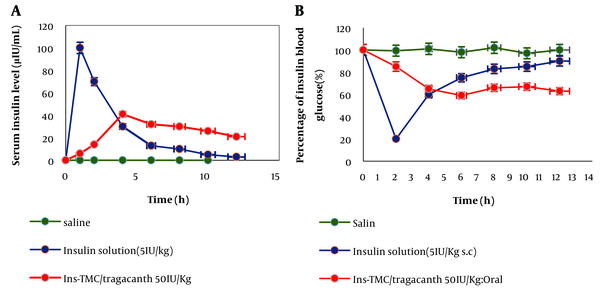
4. Discussion
The method presented in this research is a cheap and facile approach to preparing a composite applicable to oral and subcutaneous insulin delivery. Water-soluble tragacanth (WST), TMC, and insulin were well mixed by ultrasonication. It was revealed by the in vitro release studies that the TMC/insulin/WST composite 0.86% released around 10% of insulin in the colon. The quantitative ELISA showed that insulin was 100% available in TMC/insulin/WST composite. In vivo testing, using the streptozotocin-induced diabetic rat model, demonstrated that the oral administration of the composite loaded with insulin at a 30 IU/kg dose contributed to nearly 2 h of lowering blood glucose levels to about 40% of the basal glucose. However, the same sample injected subcutaneously decreased blood glucose levels to about 60% of the basal glucose within around 10 h, and the blood glucose level rose after 18 h and reached above 80%. The cytotoxicity effect was also tested using the L-929 cell.
4.1. Conclusions
In summary, TMC/tragacanth micro-carrier have been prepared for overcoming multiple barriers to insulin oral delivery. TMC/tragacanth has a high percentage of insulin and is very stable. Insulin sits on TMC, and tragacanth acts as a hydrogel and protects insulin against stomach acid. Cytotoxicity studies have shown that TMC/tragacanth exhibits low toxicity and higher stability. We see no adverse effects with the increased insulin concentration loaded on them. According to the in vivo studies, following the oral administration of TMC/tragacanth with insulin, mice could experience long-term hypoglycemia. We also expect this method to have more significant potential for research and development so that it can even pass human clinical phases in the future and enter the industrial formulation and pharmaceuticals field. This compound promises to be particles based on chitosan derivatives and hydrogels as drug carriers for the oral administration of insulin, other peptides, and even other biomacromolecules.
References
-
1.
Elsayed A, Al-Remawi M, Jaber N, Abu-Salah KM. Advances in buccal and oral delivery of insulin. Int J Pharm. 2023;633:122623. [PubMed ID: 36681204]. https://doi.org/10.1016/j.ijpharm.2023.122623.
-
2.
Mor N, Raghav N. Design and development of carboxymethylcellulose ester of curcumin as sustained release delivery system in liver. Int J Biol Macromol. 2023;231:123296. [PubMed ID: 36649863]. https://doi.org/10.1016/j.ijbiomac.2023.123296.
-
3.
Jaya Prakash N, Shanmugarajan D, Kandasubramanian B, Khot P, Kodam K. Biodegradable silk-curcumin composite for sustained drug release and visual wound monitoring. Mater Today Chem. 2023;27:101289. https://doi.org/10.1016/j.mtchem.2022.101289.
-
4.
Nezamdoost-Sani N, Khaledabad MA, Amiri S, Mousavi Khaneghah A. Alginate and derivatives hydrogels in encapsulation of probiotic bacteria: An updated review. Food Biosci. 2023;52:102433. https://doi.org/10.1016/j.fbio.2023.102433.
-
5.
Huang KX, Zhou LY, Chen JQ, Peng N, Chen HX, Gu HZ, et al. Applications and perspectives of quaternized cellulose, chitin and chitosan: A review. Int J Biol Macromol. 2023;242(Pt 3):124990. [PubMed ID: 37211070]. https://doi.org/10.1016/j.ijbiomac.2023.124990.
-
6.
Ejazi SA, Louisthelmy R, Maisel K. Mechanisms of Nanoparticle Transport across Intestinal Tissue: An Oral Delivery Perspective. ACS Nano. 2023;17(14):13044-61. [PubMed ID: 37410891]. https://doi.org/10.1021/acsnano.3c02403.
-
7.
Chen H, Zhuang J, Wu X, Shen X, Zhang Q, Zhang W. Preparation of the Chitosan/Poly-gamma-Glutamic Acid/Glabrid in Hybrid Nanoparticles and Study on its Releasing Property. Curr Drug Deliv. 2023;20(8):1195-205. [PubMed ID: 35570557]. https://doi.org/10.2174/1567201819666220513122319.
-
8.
Siafaka PI, Gundogdu EA, Caglar ES, Ozgenc E, Gonzalez-Alvarez M, Gonzalez-Alvarez I, et al. Polymer based Gels: Recent and Future Applications in Drug Delivery Field. Curr Drug Deliv. 2023;20(9):1288-313. [PubMed ID: 36082850]. https://doi.org/10.2174/1567201819666220907124040.
-
9.
Schwartz MW, Krinsley JS, Faber CL, Hirsch IB, Brownlee M. Brain Glucose Sensing and the Problem of Relative Hypoglycemia. Diabetes Care. 2023;46(2):237-44. [PubMed ID: 36701597]. [PubMed Central ID: PMC9887623]. https://doi.org/10.2337/dc22-1445.
-
10.
Chandrakar K, Patel JL, Vedula RR, Virendra RK, Mahapatra SP, Penta S, et al. Recent Developments in Multi-component Synthesis of Lawsone Derivatives. Curr Org Synth. 2023;20(3):278-307. [PubMed ID: 35366777]. https://doi.org/10.2174/1570179419666220401121714.
-
11.
Hamrang R, Moniri E, Heydarinasab A, Safaeijavan R. In vitro evaluation of copper sulfide nanoparticles decorated with folic acid/chitosan as a novel pH-sensitive nanocarrier for the efficient controlled targeted delivery of cytarabine as an anticancer drug. Biotechnol Appl Biochem. 2023;70(1):330-43. [PubMed ID: 35561253]. https://doi.org/10.1002/bab.2355.
-
12.
Zeng L, Lee J, Jo Y, Choi M. Effects of micro- and nano-sized emulsions on physicochemical properties of emulsion–gelatin composite gels. Food Hydrocolloids. 2023;139:108537. https://doi.org/10.1016/j.foodhyd.2023.108537.
-
13.
Feng Y, Ge J, Zhang D, Bai X, Fang H, Zhou Y, et al. Development a novel drug delivery formulation targeting to esophageal squamous cell carcinoma. Mater Today Adv. 2023;19:100407. https://doi.org/10.1016/j.mtadv.2023.100407.
-
14.
Zhu C, Zhang X, Gan J, Geng D, Bian X, Cheng Y, et al. A pH-sensitive hydrogel based on carboxymethylated konjac glucomannan crosslinked by sodium trimetaphosphate: Synthesis, characterization, swelling behavior and controlled drug release. Int J Biol Macromol. 2023;232:123392. [PubMed ID: 36702219]. https://doi.org/10.1016/j.ijbiomac.2023.123392.
-
15.
Kamath PP, Rajeevan R, Maity S, Nayak Y, Narayan R, Mehta CH, et al. Development of nanostructured lipid carriers loaded caffeic acid topical cream for prevention of inflammation in wistar rat model. J Appl Pharm Sci. 2023;13(1):64-75. https://doi.org/10.7324/JAPS.2023.130106-1.
-
16.
Abdel Mageed SS, Doghish AS, Ismail A, El-Husseiny AA, Fawzi SF, Mahmoud AMA, et al. The role of miRNAs in insulin resistance and diabetic macrovascular complications - A review. Int J Biol Macromol. 2023;230:123189. [PubMed ID: 36623613]. https://doi.org/10.1016/j.ijbiomac.2023.123189.
-
17.
Rajendran AP, Ogundana O, Morales LC, Meenakshi Sundaram DN, Kucharski C, Kc R, et al. Transfection Efficacy and Cellular Uptake of Lipid-Modified Polyethyleneimine Derivatives for Anionic Nanoparticles as Gene Delivery Vectors. ACS Appl Bio Mater. 2023;6(3):1105-21. [PubMed ID: 36853230]. https://doi.org/10.1021/acsabm.2c00978.
-
18.
Ma Z, Yeoh HH, Lim L. Formulation pH modulates the interaction of insulin with chitosan nanoparticles. J Pharm Sci. 2002;91(6):1396-404. https://doi.org/10.1002/jps.10149.