Abstract
Background:
Neurotoxicity is one of the most recognized effects of acrylamide (ACR) in humans and animals. Oxidative stress is an important mechanism in ACR-induced neurotoxicity.Objectives:
In this research, the effect of silymarin as a potent antioxidant was evaluated against ACR-induced toxicity in both in vitro and in vivo models.Methods:
For the in vitro assay, PC12 cells were exposed to different concentrations (2.5 - 100 µM) of silymarin for 24 hours. ACR at a final concentration of 5 mM was added and cell viability was determined using the MTT assay. For the in vivo study, neurotoxicity was induced using intraperitoneal (IP) administration of ACR (50 mg/kg) for 11 days. The effects of different doses of silymarin (40, 80, and 160 mg/kg IP, respectively) were evaluated in ACR-induced neurotoxicity in Wistar rats based on gait scores.Results:
Exposure to ACR reduced cell viability in PC12 cells. Pre-treatment of cells with silymarin (100 µM) significantly reduced ACR-induced toxicity. In addition, administration of ACR induced severe toxicity in Wistar rats, while silymarin at a dose of 160 mg/kg could improve the rats’ gait abnormalities.Conclusions:
With regard to the potent antioxidant properties of silymarin, the neuroprotective effects of this natural compound suggested in the current study may in part be mediated through inhibition of oxidative stress.Keywords
1. Background
Acrylamide (ACR, 2-propenamide, C3H5NO) is a colorless, odorless crystalline produced by the hydration of acrylonitrile. It is soluble in polar solvents, such as water and alcohol (1, 2). ACR is used in the production of polymers for applications in various chemical industries, such as cosmetics, water and wastewater management, road construction, and papermaking. It also has an important application in laboratory research for the gel electrophoretic separation of molecules. Because of its wide application, people experience high levels of exposure (3). Additionally, ACR has been found in various thermally processed foods, such as potato chips, biscuits, and coffee. The average daily ACR intake has been calculated at 34.03 μg/person/day, which is equivalent to 0.57 μg/kg body weight/day (based on a body weight of 60 kg) (4).
Neurotoxicity is a major consequence of exposure to ACR, and considerable attention has been drawn to this area of investigation over the past 40 years. Symptoms include ataxia, skeletal muscle weakness, weight loss, and sensory and motor dysfunction (5, 6).
It is suggested that oxidative stress plays a major role in the progression of ACR neurotoxicity (7). ACR is conjugated with reduced glutathione (GSH) and the resulting complex is metabolized by cytochrome P450 (subtype CYP 2E1) to form glycidamide. This increases lipid peroxidation and reduces antioxidant capacity in nervous tissue and the sciatic nerve (8). Exposure to ACR elevates the generation of reactive oxygen species and induces apoptosis in PC12 cells (9). Also, in rodent models, it has caused lipid peroxidation, depletion in GSH levels, and alterations in apoptotic markers (8, 10-13).
Several studies have demonstrated that natural compounds with antioxidant properties could be important tools for decreasing the progression of neurodegenerative disease in animal models, as well as in humans (14-19). Silybum marianum (milk thistle) has been used as a hepatoprotective compound and a liver detoxifier and for the treatment of bile duct and gallbladder disorders (20). The renal protection effects of silymarin have been reported (21). Silymarin is a lipophilic extract from the seeds (fruit) of the milk thistle plant (20), which is composed of a mixture of lignan-derived flavonols containing mostly silibinin (silybin), silydianin, silychristin, and isosilybin (22, 23). The potent antioxidant properties of silymarin (24) and its ability to penetrate the blood–brain barrier support the use of this natural compound as a possible neuroprotective agent (25). Silymarin has been shown to significantly reduce lipid peroxidation (26) and to improve psychomotor and cognitive abnormalities in animal models (27).
One study showed that the neuroprotective effects of silymarin on cerebral ischemic damage in middle cerebral artery occlusion (MCAO)-reperfusion rats was probably mediated by the inhibition of neurological deficits and oxidative damage, followed by the inhibition of apoptosis responses. These observations suggest that silymarin may be a potential neuroprotective agent against a variety of conditions in which cellular damage is a consequence of oxidative stress (28).
2. Objectives
In this study, the possible neuroprotective effect of silymarin, a well-known antioxidant, on ACR-induced neurotoxicity was evaluated using both in vitro and in vivo models.
3. Methods
3.1. Chemicals
RPMI 1640 and fetal bovine serum (FBS) were acquired from Gibco, while the 3-(4, 5-Dimethylthiazol-2-yl)-2, 5-diphenyl tetrazolium bromide (MTT) and silymarin were obtained from Sigma. Acrylamide was purchased from Merck.
3.2. Cell Culture
PC12 cells, the rat adrenal pheochromocytoma cell line, were obtained from the Pasteur institute (Tehran, Iran). The cells were cultured as follows: 37°C with 5% CO2 and a humidified atmosphere (90%). The cells were cultured in RPMI 1640 medium supplemented with 10% (v/v) heat-inactivated FBS, 100 U/mL of penicillin, and 100 mg/ml of streptomycin.
3.3. Cell Viability
Cell viability was determined using the MTT assay (9). PC12 cells were cultured in a 96-well microtiter plate at a density of 5,000 cells/well. After pretreatment with silymarin (1, 2.5, 5, 10, 20, 50, and 100 mM) for 24 hours, ACR at a final concentration of 5 mM was added to each well. After incubation for 24 hours, the cells were exposed to MTT solution (final concentration 0.5 mg/mL) for 3 hours at 37°C. Finally, the insoluble formazan was solubilized in dimethyl sulfoxide (DMSO). The absorbance was determined at 545 nm (630 nm as a reference) in an ELISA reader (Start Fax-2100, UK).
3.4. Experimental Animals
Animals were used according to the ethical committee acts and guidelines of Mashhad University of Medical Sciences committee. Male Wistar rats weighing 230 - 250 g were obtained from the Veterinary and Animal Sciences University, Mashhad, Iran. They were housed in bracket cages under standard conditions of 12 hours light/dark cycles at 21 ± 2°C and were fed commercial pellet food and water ad libitum during the dosing period.
3.5. Experimental Design
Neurotoxicity was induced using ACR at a dose of 50 mg/kg/day intraperitoneally (IP) for 11 days (5). This daily dose and corresponding route have been well-characterized with respect to neuropathological expression and neurological deficits. For our study, the rats were divided randomly into 7 groups (6 rats each) and treatment was given as follows:
1) Control, normal saline,
2) ACR, 50 mg/kg IP for 11 days,
3) ACR, 50 mg/kg IP for 11 days + silymarin 40 mg/kg,
4) ACR, 50 mg/kg IP for 11 days + silymarin 80 mg/ kg,
5) ACR, 50 mg/kg IP for 11 days + silymarin 160 mg/kg (29),
6) ACR, 50 mg/kg IP for 11 days + vitamin E 200 mg/ kg IP (30),
7) Silymarin 160 mg/kg.
It should be mentioned that the silymarin was given IP during the treatment with ACR.
3.6. Behavioral Index Examination (Gait Score)
After finishing the treatment protocol, gait scores were examined according to the methods described by LoPachin et al. (31). The rats were placed in a clear plexiglass box and observed for 3 minutes. Following the observation, a gait score was determined on a scale of 1 to 4, where 1 = normal, unaffected gait; 2 = slightly affected gait (foot splay, slight hind limb weakness, and spread); 3 = moderately affected gait (foot splay, moderate hind limb weakness, and moderate limb spread during ambulation,) and 4 = severely affected gait (foot splay, severe hind limb weakness, dragging hind limbs, and inability to rear).
3.7. Statistical Analysis
For the in vitro assay, results are expressed as mean ± SD. Statistical analyses were performed with ANOVA followed by the Tukey-Kramer test to compare the differences between means. For the in vivo assay, the results are expressed as median with range, and the Kruskal-Wallis nonparametric test was used for statistical analysis. Differences were considered statistically significant when P < 0.05.
4. Results
4.1. Effect of ACR on PC12 Cells
Exposure of PC12 cells to ACR significantly reduced cell viability in a dose-dependent manner. The IC50 was calculated at 5 mM (Figure 1).
Cell Viability of PC12 Cells After Exposure to ACR for 24 Hours
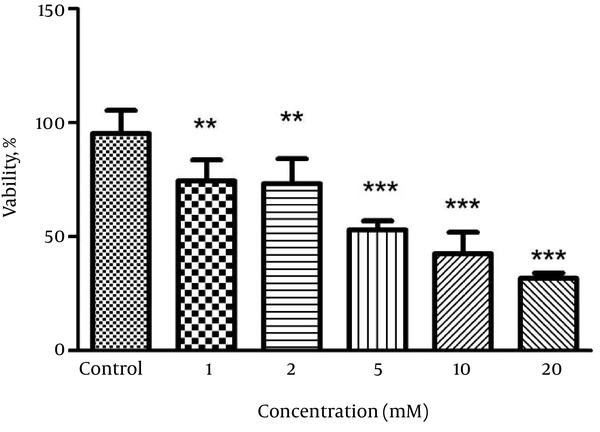
4.2. Effect of Silymarin on PC12 Cells
The PC12 cells were exposed to different concentrations of silymarin (1, 2.5, 5, 10, 20, 50, and 100 μM) for 24 and 48 hours to determine the non-toxic concentration. Silymarin at all concentrations did not alter cell viability compared to the control treated cells. The results are shown in Figure 2.
Cell Viability of PC12 Cells After Exposure to Silymarin for 24 and 48 hours
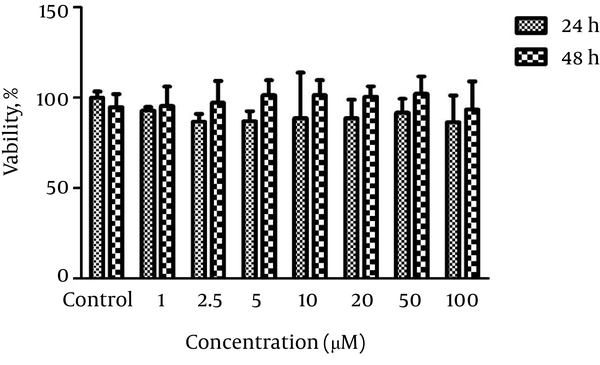
4.3. Effect of Silymarin on ACR-Induced Cytotoxicity in PC12 Cells
At the next step, to assay the protective effect of silymarin, the cells were treated with different concentrations of silymarin (2.5, 5, 10, 20, 50, and 100 μM) for 24 hours. ACR at a concentration of 5 mM was added to the cells and after 24 hours of exposure, cell viability was evaluated using the MTT assay. Treatment of cells with the 100 mM concentration of silymarin for 24 hours significantly increased cell viability compared to ACR (P < 0.001) (Figure 3).
Effect of Silymarin on ACR-Induced Cytotoxicity in PC12 Cells
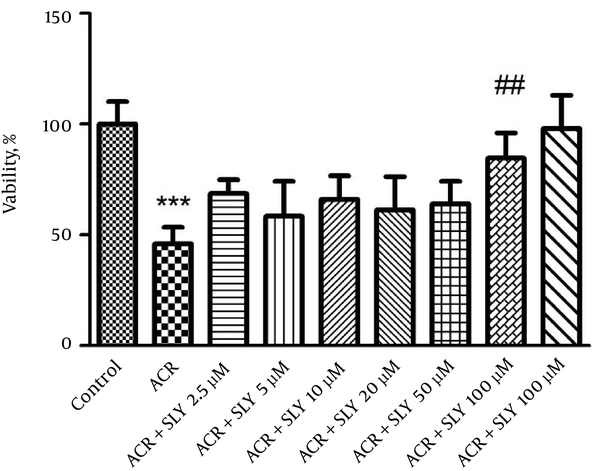
4.4. Effect of ACR on Gait Scores in Rats and the Neuroprotective Effect of Silymarin
Exposure to ACR (50 mg/kg IP) for 11 days induced progressive gait abnormalities in the rats, as shown in Figure 4. Simultaneous treatment of animals with silymarin and ACR (50 mg/kg, IP) significantly reduced ACR-induced gait abnormalities in a dose-dependent manner (P < 0.05), but the greatest neuroprotective effect of silymarin was observed at the dose of 160 mg/kg (Figure 4).
Effects of Silymarin on Behavioral Index (Gait Scores) in Rats During Treatment With ACR (50 mg/kg, IP) for 11 Days
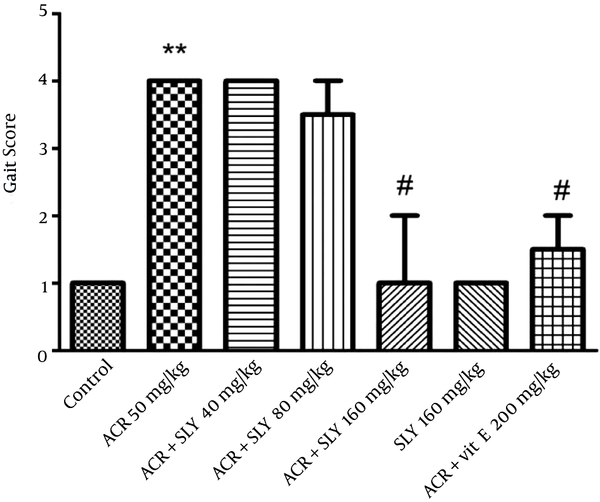
5. Discussion
According to our results, ACR induced toxicity in both in vitro and in vivo models. In addition, silymarin inhibited ACR-induced toxicity in PC12 cells. Treatment of animals with silymarin significantly reduced gait abnormalities, especially at a dose of 160 mg/kg, when the animals received it simultaneously with ACR.
Exposure to ACR could damage both the central and the peripheral nervous systems, manifesting as weight loss, ataxia, and skeletal muscle weakness (5, 32). Several mechanisms are involved in ACR-induced neurotoxicity, including the induction of oxidative stress (8, 30, 33), the apoptosis pathway (9, 34), and modulation of neurotransmitter release. ACR induces apoptosis in neurons and astrocytes in a time- and dose-dependent manner (35).
Recent evidence suggests that ACR forms adducts within presynaptic proteins, resulting in altered neurotransmission and inactivation of enzymes involved in neuronal energy production (36, 37). ACR conjugates with reduced glutathione (GSH) as the main antioxidant for detoxification. The ability of ACR to decrease antioxidant levels could be related, directly or indirectly, to its free-radical-production ability, thus leading to oxidative stress (3).
With regard to the important role of oxidative stress in ACR-induced toxicity in both in vitro and in vivo models, antioxidant agents are noted as potential factors in the inhibition of ACR toxicity. Several studies have shown neuroprotective effects of silymarin using in vitro and in vivo models. Administration of silymarin (200 mg/kg) for 2 weeks could diminish 6-hydroxydopamine–induced Parkinson’s in a rat model (38). The effects of silymarin in this model were mediated through inhibition of oxidative stress. Malondialdehyde content as a marker of lipid peroxidation significantly attenuated in the midbrain following exposure to silymarin. In another study, silymarin (200 and 400 mg/kg doses) inhibited oxidative stress in aged and young rat brains. Silymarin significantly decreased protein oxidation in the hippocampus and cortex tissues of animals (29). In the cerebral global ischemic rat model, exposure to silymarin decreased cognitive and motor function and doses of 50 - 100 µM significantly reduced amyloid β-induced toxicity in PC12 cells (39). Silymarin (20 - 80 µM) protected dopaminergic neurons against lipopolysaccharide‐induced neurotoxicity by inhibiting microglia activation (25).
The fact that the neuroprotective properties of silymarin are in part modulated through potent antioxidant activity suggests that silymarin’s effects on ACR-induced toxicity in PC12 cells and in rats may be due its antioxidant activity.
In the present study, vitamin E was used as a positive control for protection against ACR-induced neurotoxicity. The results showed no difference between the effects of vitamin E and silymarin (160 mg/kg) on the inhibition of animal gait abnormalities. Therefore, the findings suggest that the protective effect of silymarin against ACR-induced toxicity in PC12 cells and Wistar rats may be partly due to the potent antioxidant properties of this natural component.
Acknowledgements
References
-
1.
Hu Q, Xu X, Fu Y, Li Y. Rapid methods for detecting acrylamide in thermally processed foods: A review. Food Control. 2015;56:135-46.
-
2.
Friedman M. Chemistry, biochemistry, and safety of acrylamide. A review. J Agric Food Chem. 2003;51(16):4504-26. [PubMed ID: 14705871]. https://doi.org/10.1021/jf030204+.
-
3.
Adewale OO, Brimson JM, Odunola OA, Gbadegesin MA, Owumi SE, Isidoro C, et al. The Potential for Plant Derivatives against Acrylamide Neurotoxicity. Phytother Res. 2015;29(7):978-85. [PubMed ID: 25886076]. https://doi.org/10.1002/ptr.5353.
-
4.
S Tawfik M, G El-Ziney M. Acrylamide levels in selected foods in Saudi Arabia with reference to health-risk assessment of dietary acrylamide intake. Am J Food Technol. 2008;3:347-53.
-
5.
LoPachin RM. Acrylamide neurotoxicity: neurological, morhological and molecular endpoints in animal models. Adv Exp Med Biol. 2005;561:21-37. [PubMed ID: 16438286]. https://doi.org/10.1007/0-387-24980-X_2.
-
6.
El-Tantavi HGM. The protective role of ginger (Zingiber officinale) against acrylamide induced neurotoxicity in mice. Egypt J Histol. 2007;30:325-36.
-
7.
Mehri S, Karami HV, Hassani FV, Hosseinzadeh H. Chrysin reduced acrylamide-induced neurotoxicity in both in vitro and in vivo assessments. Iran Biomed J. 2014;18(2):101-6. [PubMed ID: 24518551].
-
8.
Zhu YJ, Zeng T, Zhu YB, Yu SF, Wang QS, Zhang LP, et al. Effects of acrylamide on the nervous tissue antioxidant system and sciatic nerve electrophysiology in the rat. Neurochem Res. 2008;33(11):2310-7. [PubMed ID: 18470611]. https://doi.org/10.1007/s11064-008-9730-9.
-
9.
Mehri S, Abnous K, Mousavi SH, Shariaty VM, Hosseinzadeh H. Neuroprotective effect of crocin on acrylamide-induced cytotoxicity in PC12 cells. Cell Mol Neurobiol. 2012;32(2):227-35. [PubMed ID: 21901509]. https://doi.org/10.1007/s10571-011-9752-8.
-
10.
Prasad SN. Neuroprotective efficacy of eugenol and isoeugenol in acrylamide-induced neuropathy in rats: behavioral and biochemical evidence. Neurochem Res. 2013;38(2):330-45. [PubMed ID: 23161090]. https://doi.org/10.1007/s11064-012-0924-9.
-
11.
Ghareeb DA, Khalil AA, Elbassoumy AM, Hussien HM, Abo-Sraiaa MM. Ameliorated effects of garlic (Allium sativum) on biomarkers of subchronic acrylamide hepatotoxicity and brain toxicity in rats. Toxicol Environ Chem. 2010;92(7):1357-72.
-
12.
Mehri S, Abnous K, Khooei A, Mousavi SH, Shariaty VM, Hosseinzadeh H. Crocin reduced acrylamide-induced neurotoxicity in Wistar rat through inhibition of oxidative stress. Iran J Basic Med Sci. 2015;18(9):902-8. [PubMed ID: 26523222].
-
13.
Mehri S, Shahi M, Razavi BM, Hassani FV, Hosseinzadeh H. Neuroprotective effect of thymoquinone in acrylamide-induced neurotoxicity in Wistar rats. Iran J Basic Med Sci. 2014;17(12):1007-11. [PubMed ID: 25859305].
-
14.
Busanello A, Barbosa NB, Peroza LR, Farias LE, Burger ME, Barreto KP, et al. Resveratrol protects against a model of vacuous chewing movements induced by reserpine in mice. Behav Pharmacol. 2011;22(1):71-5. [PubMed ID: 21127417]. https://doi.org/10.1097/FBP.0b013e328341e9b4.
-
15.
Busanello A, Peroza LR, Wagner C, Sudati JH, Pereira RP, Prestes Ade S, et al. Resveratrol reduces vacuous chewing movements induced by acute treatment with fluphenazine. Pharmacol Biochem Behav. 2012;101(2):307-10. [PubMed ID: 22266770]. https://doi.org/10.1016/j.pbb.2012.01.007.
-
16.
Lu P, Mamiya T, Lu LL, Mouri A, Zou L, Nagai T, et al. Silibinin prevents amyloid beta peptide-induced memory impairment and oxidative stress in mice. Br J Pharmacol. 2009;157(7):1270-7. [PubMed ID: 19552690]. https://doi.org/10.1111/j.1476-5381.2009.00295.x.
-
17.
Peroza LR, Busanello A, Leal CQ, Ropke J, Boligon AA, Meinerz D, et al. Bauhinia forficata prevents vacuous chewing movements induced by haloperidol in rats and has antioxidant potential in vitro. Neurochem Res. 2013;38(4):789-96. [PubMed ID: 23377855]. https://doi.org/10.1007/s11064-013-0981-8.
-
18.
Rasool M, Malik A, Qureshi MS, Manan A, Pushparaj PN, Asif M, et al. Recent updates in the treatment of neurodegenerative disorders using natural compounds. Evid Based Complement Alternat Med. 2014;2014:979730. [PubMed ID: 24864161]. https://doi.org/10.1155/2014/979730.
-
19.
Reckziegel P, Peroza LR, Schaffer LF, Ferrari MC, de Freitas CM, Burger ME, et al. Gallic acid decreases vacuous chewing movements induced by reserpine in rats. Pharmacol Biochem Behav. 2013;104:132-7. [PubMed ID: 23313549]. https://doi.org/10.1016/j.pbb.2013.01.001.
-
20.
Mastron JK, Siveen KS, Sethi G, Bishayee A. Silymarin and hepatocellular carcinoma: a systematic, comprehensive, and critical review. Anticancer Drugs. 2015;26(5):475-86. [PubMed ID: 25603021]. https://doi.org/10.1097/CAD.0000000000000211.
-
21.
Karimi G, Ramezani M, Tahoonian Z. Cisplatin nephrotoxicity and protection by milk thistle extract in rats. Evid Based Complement Alternat Med. 2005;2(3):383-6.
-
22.
Kvasnicka F, Biba B, Sevcik R, Voldrich M, Kratka J. Analysis of the active components of silymarin. J Chromatogr A. 2003;990(1-2):239-45. [PubMed ID: 12685603].
-
23.
Karimi G, Vahabzadeh M, Lari P, Rashedinia M, Moshiri M. "Silymarin", a promising pharmacological agent for treatment of diseases. Iran J Basic Med Sci. 2011;14(4):308-17. [PubMed ID: 23492971].
-
24.
Karimi G, Hassanzadeh M, Mehri S. Protective effects of Silymarin against free radical-induced erythrocyte lysis. J Complement Integrat Med. 2006;3(1).
-
25.
Wang MJ, Lin WW, Chen HL, Chang YH, Ou HC, Kuo JS, et al. Silymarin protects dopaminergic neurons against lipopolysaccharide-induced neurotoxicity by inhibiting microglia activation. Eur J Neurosci. 2002;16(11):2103-12. [PubMed ID: 12473078].
-
26.
de Oliveira D, Busanello A, Barbosa CP, Peroza LR, de Freitas CM, Krum BN, et al. Silymarin has antioxidant potential and changes the activity of Na+/K+-ATPase and monoamine oxidase in vitro. Indust Crops Prod. 2015;70:347-55.
-
27.
Lu P, Mamiya T, Lu L, Mouri A, Niwa M, Kim HC, et al. Silibinin attenuates cognitive deficits and decreases of dopamine and serotonin induced by repeated methamphetamine treatment. Behav Brain Res. 2010;207(2):387-93. [PubMed ID: 19857526]. https://doi.org/10.1016/j.bbr.2009.10.024.
-
28.
Raza SS, Khan MM, Ashafaq M, Ahmad A, Khuwaja G, Khan A, et al. Silymarin protects neurons from oxidative stress associated damages in focal cerebral ischemia: a behavioral, biochemical and immunohistological study in Wistar rats. J Neurol Sci. 2011;309(1-2):45-54. [PubMed ID: 21840019]. https://doi.org/10.1016/j.jns.2011.07.035.
-
29.
Galhardi F, Mesquita K, Monserrat JM, Barros DM. Effect of silymarin on biochemical parameters of oxidative stress in aged and young rat brain. Food Chem Toxicol. 2009;47(10):2655-60. [PubMed ID: 19647779]. https://doi.org/10.1016/j.fct.2009.07.030.
-
30.
Mehri S, Meshki MA, Hosseinzadeh H. Linalool as a neuroprotective agent against acrylamide-induced neurotoxicity in Wistar rats. Drug Chem Toxicol. 2015;38(2):162-6.
-
31.
LoPachin RM, Ross JF, Reid ML, Das S, Mansukhani S, Lehning EJ. Neurological evaluation of toxic axonopathies in rats: acrylamide and 2,5-hexanedione. Neurotoxicology. 2002;23(1):95-110. [PubMed ID: 12164553].
-
32.
Esmaeelpanah E, Rahmatkhah A, Poormahmood N, Razavi BM, Hasani F, Hosseinzadeh H. Protective Effect of Green Tea Aqueous Extract on Acrylamide Induced Neurotoxicity. Jundishapur J Nat Pharm Prod. 2015;10(2).
-
33.
Motamedshariaty VS, Amel Farzad S, Nassiri-Asl M, Hosseinzadeh H. Effects of rutin on acrylamide-induced neurotoxicity. Daru. 2014;22(1):27. [PubMed ID: 24524427]. https://doi.org/10.1186/2008-2231-22-27.
-
34.
Alturfan AA, Tozan-Beceren A, Sehirli AO, Demiralp E, Sener G, Omurtag GZ. Resveratrol ameliorates oxidative DNA damage and protects against acrylamide-induced oxidative stress in rats. Mol Biol Rep. 2012;39(4):4589-96. [PubMed ID: 21947844]. https://doi.org/10.1007/s11033-011-1249-5.
-
35.
Chen JH, Wu KY, Chiu IM, Tsou TC, Chou CC. Acrylamide-induced astrogliotic and apoptotic responses in human astrocytoma cells. Toxicol In Vitro. 2009;23(5):855-61. [PubMed ID: 19426797]. https://doi.org/10.1016/j.tiv.2009.04.015.
-
36.
LoPachin RM, Gavin T. Molecular mechanism of acrylamide neurotoxicity: lessons learned from organic chemistry. Environ Health Perspect. 2012;120(12):1650-7. [PubMed ID: 23060388]. https://doi.org/10.1289/ehp.1205432.
-
37.
Martyniuk CJ, Feswick A, Fang B, Koomen JM, Barber DS, Gavin T, et al. Protein targets of acrylamide adduct formation in cultured rat dopaminergic cells. Toxicol Lett. 2013;219(3):279-87. [PubMed ID: 23566896]. https://doi.org/10.1016/j.toxlet.2013.03.031.
-
38.
Baluchnejadmojarad T, Roghani M, Mafakheri M. Neuroprotective effect of silymarin in 6-hydroxydopamine hemi-parkinsonian rat: involvement of estrogen receptors and oxidative stress. Neurosci Lett. 2010;480(3):206-10. [PubMed ID: 20600617]. https://doi.org/10.1016/j.neulet.2010.06.038.
-
39.
Murata N, Murakami K, Ozawa Y, Kinoshita N, Irie K, Shirasawa T, et al. Silymarin attenuated the amyloid beta plaque burden and improved behavioral abnormalities in an Alzheimer's disease mouse model. Biosci Biotechnol Biochem. 2010;74(11):2299-306. [PubMed ID: 21071836]. https://doi.org/10.1271/bbb.100524.