Abstract
Keywords
Naproxen Microemulsion Transdermal Drug Delivery Skin Permeation
1. Background
As one of the popular NSAIDs, NPX has been widely used to treat pain, arthritis, and inflammatory disorders. However, side effects of traditional administration of NSAIDs, particularly NPX, including poor aqueous solubility and permeability properties, might limit their clinical applications (1-3). Many studies have focused on addressing these problems through the development of novel, highly efficient vehicles for optimized NSAIDs delivery. Hence, to overcome the aforementioned drawbacks, drug delivery nanocarriers such as MEs, lipid-based, polymeric-based materials, and others prove to be effective solutions. These carriers can address various pharmaceutical limitations, such as solubility, permeability, bioavailability, and reduced side effects, which are among the main challenges of NSAIDs (4, 5).
One of the most recent attempts in NPX delivery is the development of transdermal-based formulations to optimize its solubility and skin permeation, which may influence clinical translation. Moreover, transdermally administered NPX, compared to other routes, remarkably improves therapeutic effects (2, 3, 6). Overall, transdermal delivery of drugs, compared to oral and intravenous routes, offers several benefits, including efficient penetration into deeper layers of the skin, non-invasive application, reduced systemic side effects (such as gastric disorders), avoidance of pre-systemic metabolism in the liver, targeted delivery, prolonged drug release profile, and ease of use. Nevertheless, the permeability issue of drug molecules through the skin barrier poses a significant challenge (4, 5, 7).
ME-based carriers have recently attracted attention in preparing transdermal formulations for drug delivery due to their tunable physicochemical properties, such as droplet size and morphology, homogeneity, surface area, stability, and permeability. MEs, thermodynamically stable liquids, are composed of aqueous nanodroplets encapsulated within a mixture of surfactant and co-surfactant, dispersed in an oil phase. Additionally, by adjusting the concentration and nature of the organic and aqueous phases, the physicochemical properties of the droplets can be easily modified. In this context, MEs are recognized as promising materials for application in the fabrication of nanocarriers, particularly for skin vehicles, due to their unique structural properties. Moreover, the relatively straightforward preparation and scalability of MEs make them attractive for biomedical applications (8-11).
MEs have great potential to be used as transdermal carriers to increase the solubility of both hydrophobic and hydrophilic drugs, while simultaneously enhancing skin permeability (12-14). The main advantages of ME-based transdermal carriers, compared to other conventional formulations of NSAIDs, include local and controllable drug release, as well as sufficient distribution of drugs into various layers of the skin, resulting in reduced side effects. Hence, MEs, as transdermal carriers, can offer favorable physicochemical properties, especially in terms of skin permeability (15, 16). However, the use of large amounts of S/CS, phase inversion, and the selection of safe excipients for pharmaceutical application pose major disadvantages from both formulation and biocompatibility perspectives (17). Therefore, new assessments are necessary for MEs to guide their fabrication towards optimal formulations in pharmaceutical applications, particularly in transdermal formulations.
There are very few studies on ME formulation for NPX. In prior studies, Salimi et al. characterized and evaluated the skin penetration of NPX on various components (e.g., Tween 80, Span 80, PG, TRC-P, and +LabrafacTM PG) of prepared MEs.
2. Objectives
Herein, we prepared a series of MEs (W/O) containing NPX, which could improve the permeability of the corresponding NPX in a rat skin model (18-20). Meanwhile, the permeation parameters such as steady-state flux (Jss), permeability coefficient (P), lag time (Tlag), apparent diffusion coefficient (Dapp), and enhancement ratio (ERflux, ERD, and ERP) of NPX permeated were investigated. The permeation analysis was evaluated using a Franz diffusion cell set with rat skin. Furthermore, the skin permeability potential of MEs was evaluated with different enhancers including EU oil, OLA, and TRC-P.
3. Methods
3.1. Materials
Naproxen (w ≥ 99%) was purchased from Pars Darou Co, IRAN. Span 80, Tween 80, Oleic acid, and Propylene glycol (PG) were supplied by Merck Co, Germany. Propylene glycol dicaprylocaprate (LabrafacTM PG) and Diethylene glycol monoethyl ether (Transcutol P) were received as gift samples from GATTEFOSSE, France. EU oil was purchased from Barij Essence Co, IRAN. All materials were of reagent grade and applied without further purification.
3.2. Animals
The skin permeation of NPX-MEs was investigated on Wistar rats. The male rats weighing 200-250 g (8 - 10 weeks old) were supplied by the Animals Laboratory, Ahvaz Jundishapur University of Medical Sciences. The experimental protocol was approved by the Animal Ethics Committee of Jundishapur University of Medical Sciences, Faculty of Pharmacy (Approval No. N 75).
3.3. MEs Region Identification
The concentration of the different constituents in the MEs can be chosen according to the water titration method using pseudo-ternary phase diagrams. Here, two phase diagrams were first prepared by accurately weighing Span 80 / Tween 80 (1.1) as surfactant and PG as co-surfactant. One contained a 4: 1 weight ratio, and the second contained a 6: 1 weight ratio of S/C mixture. EU oil, OLA, and TRC-P (+ LabrafacTM PG) (1.10) were selected as oil phases and were accurately weighed in the vial. For the preparation of each phase diagram, the mixture of S/C was then added to the oil phase at weight ratios of 1: 9, 2: 8, 3: 7, 4:6, 5: 5, 6:4, 7: 3, 8: 2, and 9: 1. The resulting S/C-oil mixture was homogeneously mixed, and then deionized water was added under continuous titration until a transparent dispersion was formed. The transparent monophasic liquids were classified as MEs. The MEs were formed spontaneously at room temperature. Pseudo-ternary phase diagrams were investigated to study the region of MEs existence as well as the quaternary system of water/ LabrafacTM PG, Transcutol P (10: 1)/ Span 80-Tween 80/ PG. These diagrams were previously reported in our studies, and the results are shown in Figure 1 (21).
The pseudo-ternary phase diagrams of the water-oil-S/C mixture system at the 4: 1 and 6: 1 weight ratio of Span 80 /Tween 80/ PEG at ambient temperature, dark area show MEs zone
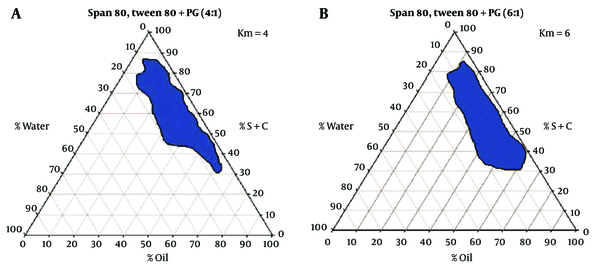
3.4. Preparation of NPX-MEs
NPX-MEs were constructed following the same formulations used in our previous works (21). A full factorial design, as a convenient method to prepare MEs, was used with three variables at two levels. The percentages of oil and water phases and S/C ratio have been proposed as the main variables, and eight different formulations of MEs were selected from the phase diagrams with low and high levels of oil (20% and 40%), water (5%, 10%), and S/C ratio (4: 1, 6: 1). NPX-MEs formulations were prepared as follows in Table 1. The oil phase contains 1% of NPX, and the S/C mixture was separately prepared and then mixed. Afterward, a certain amount of deionized water was added dropwise into the previous mixture under stirring at room temperature until a uniform phase was formed.
Components of the Selected NPX-MEs
Batch No. | Factorial | S/C | % Oil | % (S + C) | % Water |
---|---|---|---|---|---|
ME-1 | +++ | 6: 1 | 40 | 50 | 10 |
ME-2 | ++- | 6: 1 | 40 | 55 | 5 |
ME-3 | +-+ | 6: 1 | 20 | 70 | 10 |
ME-4 | +-- | 6: 1 | 20 | 74 | 5 |
ME-5 | --- | 4: 1 | 20 | 75 | 5 |
ME-6 | --+ | 4: 1 | 20 | 70 | 10 |
ME-7 | -+- | 4: 1 | 40 | 55 | 5 |
ME-8 | -++ | 4: 1 | 40 | 50 | 10 |
3.5. Particle Size
All prepared NPX-MEs were characterized by dynamic light scattering (DLS) using SCATTER SCOPE 1 QUIDIX (South Korea) to measure the particle size of droplets.
3.6. Refractive Index
The refractive index measurements were conducted using a refractometer instrument (Quarts, Ceti, Belgium).
3.7. Viscosity
The viscosity of NPX-MEs was measured using a Brookfield viscometer (DV-II + Pro, Brookfield, USA). For the viscosity evaluation of ME-NPX formulations, 10 mL of sample was poured into a beaker, and the viscosity was estimated using Spindle number 34 at room temperature with a shear rate of 100 rpm.
3.8. Analytical Method of NPX
A UV assay method for NPX was validated, and the quantitative analysis of NPX was estimated using a UV-Visible spectrophotometer (BioWaveII, WPA, England) at λmax = 271 nm.
3.9. Ex vivo Permeation Study
Skin samples were obtained from male rats. Immediately after shaving the hair, abdominal skin was excised, and the subcutaneous fatty tissue was separated. The hairless skin was then cut into smaller pieces, wrapped in aluminum foil, and stored at -20°C until use. All permeation experiments were performed using Franz cells with a diffusional area of 4.9 cm2. All skin samples were hydrated in distilled water before permeation studies and checked for integrity. The thickness of skin samples was 0.055 ± 0.005 cm. Phosphate buffer saline (PBS; pH 7.4) was used as the receptor medium. Each skin sample was placed between the donor and receptor chambers so that the stratum corneum of the skin surface was exposed to the donor solution. Then, 23 mL of PBS was poured into the receptor chamber. The donor chamber was filled with the MEs formulation or a saturated solution of NPX as the control sample. The Franz diffusion cell was kept in an oil bath setup at 37°C under stirring. At specific interval times, 2 mL of samples were extracted from the receptor chamber up to 56 h for UV assay analysis at 271 nm. To maintain sink conditions after every sample withdrawal, the receptor chamber was filled with 2 mL of fresh PBS. Additionally, EU oil, OLA, and TRC-P were used as enhancers to increase skin permeability. The effect of these enhancers was also evaluated through rat skin. For this purpose, the hydrated skin samples were treated with 1 mL of each enhancer in the donor chamber before the addition of the MEs formulation. After 2 h, both the donor and acceptor chambers were washed with distilled water, and the experiment was performed following the same procedure as described above.
3.10. Statistical Data Analysis
To calculate the permeation parameters of NPX, the cumulative mass of released NPX per unit surface area of the rat skin versus time was first plotted. The Jss (mg/cm2.h) value through the rat skin into the acceptor medium was determined by the slope of the linear portion of the mentioned plot. The permeability coefficient includes P (cm/h), Dapp (cm2/h), and ER parameters (denoted as the enhancer effect on the enhancement of NPX permeation) were calculated using the following Equations ((22, 23):
Where C, Tlag, and h were the total amount of permeated drug in the donor phase, the needed time to reach a steady state, and the length of the diffusion pathway, respectively.
All data are reported as the mean ± standard deviation (SD) from triplicate independent experiments for each sample. The statistical differences between experimental formulations were obtained by Analysis of Variance (ANOVA) using GraphPad Software. P-values less than < 0.05 were considered significant.
4. Results and Discussion
4.1. Characterization Studies
The results of the characterization of MEs formulations are listed in Table 2. The findings show that the average particle size values of the NPX-MEs range from 7.05 ± 0.03 to 79.56 ± 0.58 nm, which were in the nanoscale range. Also, the refractive index for the NPX-MEs was about 1.45, near to the oil phase, suggesting water-in-oil structures of formulations. The obtained viscosity values were in the range of 222.4 ± 0.87 to 681.13 ± 1.97 cp. Moreover, the correlation between the independent variables such as %w, % oil, and S/C, and particle size, refractive index, and viscosity values for NPX-MEs were analyzed and expressed using Equations 4 (Table 3).
Batch No. | Particle Size, nm | Refractive Index | Viscosity, cp |
---|---|---|---|
ME-1 | 7.05 ± 0.03 | 1.445 ± 0.14 | 251.3 ± 1.91 |
ME-2 | 79.56 ± 0.58 | 1.451 ± 0.15 | 222.4 ± 0.87 |
ME-3 | 53.63 ± 0.42 | 1.453 ± 0.2 | 652.63 ± 1.22 |
ME-4 | 10.26 ± 0.15 | 1.452 ± 0.18 | 681.13 ± 1.97 |
ME-5 | 21.96 ± 0.31 | 1.456 ± 0.16 | 319.4 ± 1.37 |
ME-6 | 34.4 ± 0.41 | 1.452 ± 0.21 | 341.8 ± 0.61 |
ME-7 | 10.43 ± 0.12 | 1.451 ± 0.19 | 364.16 ± 1.60 |
ME-8 | 11.6 ± 0.3 | 1.444 ± 0.17 | 475.9 ± 1.83 |
The Statistical Relationship Between Independent Variables with Particle Size, Refractive Index and Viscosity
Dependent Variables | Independent Variable | P-Value | Significant |
---|---|---|---|
Particle Size | % oil | 0.880 | No |
% w | 0.770 | No | |
S/C | 0.697 | No | |
Refractive Index | % oil | 0.970 | No |
% w | 0.001 | Yes | |
S/C | 0.001 | Yes | |
Viscosity | % oil | 0.210 | No |
% w | 0.009 | Yes | |
S/C | 0.576 | No |
According to Equation 4, there were no significant differences between the independent variables and average particle size values of the NPX-MEs. In the case of refractive index, both the S/C ratio and % w phase indicated a significant effect (P-value = 0.001); thereby, the refractive index of NPX-MEs decreases with the increase in % water phase or S/C ratio. The viscosity is affected by % w phase (P-value = 0.009), so the viscosity increases with the decrease in % w phase. Through the change of various parameters, including the oil phase, S/CS mixture, and weight ratio of excipients, MEs with different physicochemical properties can be formulated. In this context, Salimi et al. (24) prepared MEs formulations via a pseudo-ternary phase diagram-assisted full factorial method, using Tween-80 and Span 20 as S, PG as CS, TRC-P, and OLA as the oil phase to enhance transdermal delivery of meloxicam. In this study, the droplet size (5.24 to 16.75 nm) and viscosity (132 to 418 cp) of MEs were smaller compared to our study. They seemed to be influenced by the oil phase type (mixture of TRC-P/OLA) and the ratio of S/CS (1: 1 and 3: 1). Additionally, they found that the particle size was not dependent on the independent variables, coherent with the present study. For another example, Moghimipour et al. (21) fabricated quercetin-loaded MEs composed of Tween-80, Span 20, PG, TRC-P, and OLA with a size range of 5.31 - 26.07 nm and viscosity of 115 - 361 cp for transcorneal delivery application. It seems that the decreased particle size resulted in increased surface area due to the different weight ratio of MEs components. Similarly, the relationship between the droplet size and independent variables was not significant. In another study, Salimi et al. (25) developed an ME formulation for transfollicular delivery of adapalene. MEs were fabricated using TRC-P, OLA, PG, Tween-80, and Span 20. These MEs had a droplet size of 13.86 to 56.16 nm, and the viscosity ranged from 117 to 240 cp. Furthermore, statistical differences were observed between the viscosity parameter and % w, S/CS, and oil%. These findings suggest that the physicochemical properties of MEs can be affected by constituents and their weight ratio in the ME (26).
4.2. Ex-vivo Permeation Studies of NPX-MEs
Permeation of NPX from MEs carriers containing 1% NPX was evaluated using Franz diffusion cells with rat full-thickness skin. A saturated NPX solution served as the control sample. The permeation parameters and enhancement ratios of pre-prepared NPX-MEs and the control are shown in Table 4. Transdermal delivery revealed a significant increase in permeated NPX with all MEs carriers. Among the various MEs, MEs-3, composed of 20% oil, 10% water phases, and 70% S/C ratio, exhibited the highest permeation rate. The initial time point of NPX detection ranged from 11.72 ± 1.532 to 20.42 ± 15.521 hours across different MEs formulations. In ME-3 formulation, ERD (527.989 ± 313.627) notably increased compared to the control sample. These findings suggest that the higher percentage of S and C components in the prepared MEs may contribute to the increased permeation rate of NPX on the rat skin model. Surfactants, particularly, may enhance drug penetration into the intercellular lipid matrix of the stratum corneum, thereby enhancing skin permeation. Additionally, the use of TRC-P and LabrafacTM PG can modify MEs formulations. Similar results were observed in previous studies on transdermal drug delivery of NSAIDs based on MEs carriers. Ustundag Okur et al. demonstrated that incorporating different components like Labrafil, Tween 80, and Transcutol P favored the formation of MEs as carriers for drug delivery of NSAIDs such as NPX, promoting skin permeation (19). Hu et al. reported MEs-based vehicles for the transdermal delivery of ibuprofen, which also increased ibuprofen permeation through rat skin without associated skin irritation risk (13). Overall, the observed results in skin permeation studies could be attributed to various effects: (1) enhanced local NPX availability incorporated into the hydrophobic domain of MEs carriers; (2) increased distribution of drug molecules into skin layers; (3) the nonionic and hydrophobic components of MEs interacting with lipidic components and disrupting the skin structure, particularly the stratum corneum layer (20, 27, 28). Additionally, ex vivo skin permeation profiles of NPX from ME formulations are shown in Figure 2. We observed that NPX release from all MEs was approximately 6-fold higher compared to the control sample after 56 hours. NPX, being a hydrophobic drug, benefits from the nanocarriers of developed MEs, which promote controlled local release into skin layers, providing wide drug distribution and increased solubility (29-31).
Batch No. | Jss, mg/cm2.h | Dapp, cm2/h | P, cm/h | Tlag, h | ERfflux | ERD | ERP |
---|---|---|---|---|---|---|---|
Control | 0.0033 ± 0.00011 | 0.0019 ± 0.00001 | 0.0032 ± 0.0061 | 17.96 ± 0.831 | - | - | - |
ME-1 | 0.011 ± 0.002 | 0.006 ± 0.002 | 0.011 ± 0.054 | 10.99 ± 0.368 | 3.293 ± 0.671 | 2.829 ± 0.118 | 3.325 ± 0.703 |
ME-2 | 0.016 ± 0.007 | 0.009 ± 0.007 | 0.016 ± 0.001 | 20.42 ± 0.521 | 4.758 ± 0.167 | 4.538 ± 0.371 | 4.763 ± 0.175 |
ME-3 | 0.018 ± 004 | 1.36 ± 0.616 | 0.017 ± 0.002 | 1.72 ± 0.532 | 5.414 ± 0.094 | 527.989 ± 0.627 | 5.425 ± 0.089 |
ME-4 | 0.017 ± 0.008 | 0.043 ± 0.032 | 0.017 ± 0.008 | 1.87 ± 0.243 | 5.030 ± 0.273 | 21.923 ± 0.149 | 5.035 ± 0.277 |
ME-5 | 0.010 ± 0.002 | 0.021 ± 0.012 | 0.01 ± 0.002 | 3.10 ± 0.691 | 2.909 ± 0.652 | 10.632 ± 0.117 | 2.912 ± 0.653 |
ME-6 | 0.017 ± 0.002 | 0.013 ± 0.0007 | 0.017 ± 0.0007 | 3.50 ± 0.194 | 5.071 ± 0.513 | 6.720 ± 0.378 | 5.075 ± 0.513 |
ME-7 | 0.008 ± 0.0008 | 0.113 ± 0.08 | 0.007 ± 0.0008 | 2.21 ± 0.809 | 2.346 ± 0.237 | 40.790 ± 0.749 | 2.271 ± 0.227 |
ME-8 | 0.017 ± 0.004 | 0.013 ± 0.004 | 0.017 ± 0.002 | 4.88 ± 0.380 | 5.232 ± 0.353 | 6.372 ± 0.906 | 5.234 ± 0.355 |
The graph of the cumulative amount of NPX passed through the surface unit in the whole abdominal skin of the rat by pharmaceutical MEs (mean ± SD) (n = 3).
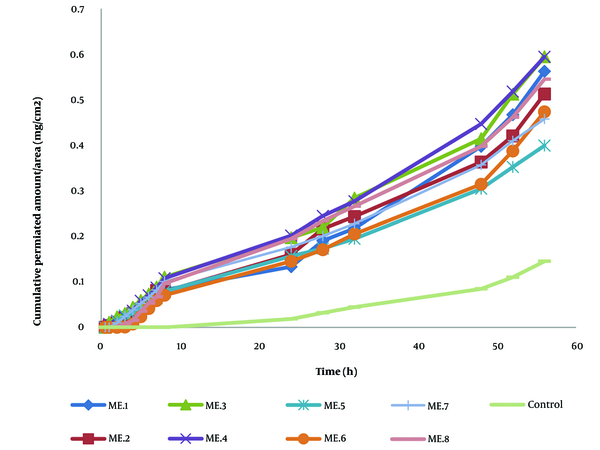
4.3. Evaluation of the Main Variables' Effects on Permeation
In this section, the independent variables of oil (TRC-P + LabrafacTM PG), water phases, and S/C ratio (Span 80/Tween 80 and PEG-400) were investigated, and their effects on the permeation parameters of MEs are discussed. Linear equations 7 - 10 indicated the impact of these variables on the amount of permeated NPX. Regression analysis of these equations revealed no significant differences between JSS, Dapp, and P values for the main variables (P > 0.05) after ANOVA analyses. However, a statistically significant difference (P < 0.05) was found between the Tlag value and water phase, while other variables showed no significant difference with the Tlag value (Table 5).
The Statistical Relationship between Independent Variables with Jss, Tlag, Dapp and P
Depended Variables | Independent Variable | P-Value | Significant |
---|---|---|---|
Jss | % oil | 0.168 | No |
% w | 0.350 | No | |
S/C | 0.077 | No | |
Tlag | % oil | 0.073 | No |
% w | 0.021 | Yes | |
S/C | 0.574 | No | |
Dapp | % oil | 0.381 | No |
% w | 0.345 | No | |
S/C | 0.424 | No | |
P | % oil | 0.611 | No |
% w | 0.591 | No | |
S/C | 0.722 | No |
4.4. Effect of Penetration Enhancers on the Skin Permeation of NPX-MEs
The ex vivo permeation of NPX was evaluated using EU oil, OLA, and TRC-P treated rat skin samples in a Franz diffusion cell, and the results are presented in Tables 6 and 7. All enhancer-treated skins exhibited better permeation compared to samples without any treatment. Among the enhancers, OLA demonstrated faster permeation than others (ERflux = 6.193 ± 0.587 and ERDapp = 75.5 ± 33.53). This increase in the skin permeation of NPX could be attributed to OLA and EU serving as active vehicles for the preparation of MEs, as reported by Abd et al. (27). Other studies have shown that the higher permeation from OLA is likely due to its attractive features, including a cis isomer structure, unsaturated bond, and solubility ability in the skin lipid bilayers, leading to disruption in the lipidic structure of the outermost layer of skin and changes in skin functions, thereby favoring enhanced drug permeation (32-35).
Batch No. | Jss, mg/cm2.h | Dapp, cm2/h | Tlag, h | ||||||
---|---|---|---|---|---|---|---|---|---|
EU Oil | TRC-P | OLA | Eu Oil | TRC-P | OLA | Eu Oil | TRC-P | OLA | |
ME-1 | 0.04 ± 0.0022 | 0.039 ± 0.003 | 0.021 ± 0.0013 | 0.071 ± 0.0029 | 0.075 ± 0.0007 | 0.372 ± 0.006 | 1.209 ± 1.2 | 0.523 ± 0.0003 | 0.083 ± 0.11 |
ME-2 | 0.036 ± 0.001 | 0.035 ± 0.001 | 0.030 ± 0.005 | 0.113 ± 0.0016 | 0.092 ± 0.01 | 0.011 ± 0.0018 | 1.064 ± 1.002 | 0.692 ± 0.0002 | 2.431 ± 1.56 |
ME-3 | 0.028 ± 0.003 | 0.038 ± 0.002 | 0.023 ± 0.001 | 0.076 ± 0.0024 | 0.064 ± 0.0031 | 0.005 ± 0.0021 | 0.812 ± 0.49 | 0.504 ± 0.027 | 3.5 ± 1.1 |
ME-4 | 0.042 ± 008 | 0.044 ± 0022 | 0.043 ± 011 | 0.016 ± 001 | 0.031 ± 0002 | 0.023 ± 0012 | 2.316 ± 0.54 | 1.691 ± 0.062 | 1.81 ± 0.57 |
ME-5 | 0.027 ± 0.0001 | 0.019 ± 0.0016 | 0.022 ± 0.0014 | 0.089 ± 0.0019 | 0.055 ± 0.0021 | 0.096 ± 0.003 | 1.026 ± 0.51 | 0.792 ± 0.41 | 0.507 ± 0.24 |
ME-6 | 0.036 ± 0.0002 | 0.017 ± 0.0012 | 0.021 ± 0.0011 | 0.423 ± 0.00023 | 0.074 ± 0.0011 | 0.029 ± 0.0011 | 0.132 ± 0.12 | 0.292 ± 0.12 | 0.736 ± 0.0125 |
ME-7 | 0.029 ± 0.001 | 0.019 ± 0.0013 | 0.048 ± 0.0021 | 0.311 ± 0.19 | 0.011 ± 0.0018 | 0.021 ± 0.001 | 0.326 ± 0.005 | 4.165 ± 1.6 | 2.029 ± 0.55 |
ME-8 | 0.03 ± 0.005 | 0.024 ± 0.0016 | 0.019 ± 0.0016 | 0.107 ± 0.072 | 0.017 ± 0.025 | 0.034 ± 0.0014 | 0.563 ± 0.125 | 2.393 ± 1.52 | 0.819 ± 0.0032 |
Batch No. | EU Oil | TRC-P | OLA | |||
---|---|---|---|---|---|---|
ERfflux | ERD | ERfflux | ERD | ERfflux | ERD | |
ME-1 | 3.794 ± 0.700 | 14.734 ± 6.448 | 3.284 ± 0.674 | 15.284 ± 6.783 | 1.935 ± 0.357 | 75.55 ± 23.532 |
ME-2 | 2.681 ± 0.344 | 55.009 ± 45.795 | 2.628 ± 1.318 | 45.148 ± 37.097 | 2.270 ± 1.138 | 5.403 ± 4.439 |
ME-3 | 1.594 ± 0.0364 | 3.127 ± 2.895 | 2.200 ± 0.503 | 2.620 ± 2.426 | 1.317 ± 0.301 | 0.199 ± 0.185 |
ME-4 | 3.077 ± 0.862 | 0.54 ± 0.0380 | 3.262 ± 1.973 | 1.050 ± 0.739 | 3.173 ± 1.92 | 0.782 ± 0.551 |
ME-5 | 2.953 ± 0.668 | 6.33 ± 5.401 | 2.080 ± 0.471 | 3.889 ± 3.319 | 2.317 ± 0.524 | 17.675 ± 16.83 |
ME-6 | 2.136 ± 0.209 | 32.173 ± 1.813 | 1.029 ± 0.101 | 5.612 ± 0.316 | 1.251 ± 0.122 | 2.231 ± 0.126 |
ME-7 | 3.892 ± 0.352 | 14.655 ± 11.417 | 2.503 ± 0.237 | 0.51 ± 0.398 | 6.193 ± 0.587 | 0.527 ± 0.813 |
ME-8 | 1.793 ± 0.497 | 9.055 ± 2.701 | 1.453 ± 0.403 | 1.420 ± 0.424 | 1.372 ± 0.325 | 2.906 ± 0.867 |
5. Conclusions
In this study, we evaluated the skin permeation of NPX using a series of W/O NPX-MEs successfully formulated and characterized in the present work. The optimal NPX formulation was selected based on permeability parameters and permeation rate. According to the permeability studies, the best formulation (ME-3) consisted of 20% TRC-P and LabrafacTM PG as oil, 10% water, and 70% of Tween 80, Span 80, and PG as S/C phases. Moreover, the skin permeation rate of ME-3 was higher compared to others. These drug-encapsulated MEs, which are favorable materials easily distributed on the skin layer, provide a desirable enhancement of drug penetration. Additionally, oil enhancers such as OLA can increase the skin permeation rate due to disruption in the lipid compartment of the stratum corneum layer. The findings showed that the skin permeation rate of NPX depended on the S/C ratio, amount of aqueous/organic phases, and type of enhancer. Furthermore, transdermal delivery studies on rat skin samples indicated a remarkable increase in the permeation of NPX for both MEs formulations and the selected enhancers, which is advantageous in the therapeutic functionality of a MEs-based nanocarrier for the topical delivery of drugs.
Acknowledgements
References
-
1.
Martí-Centelles R, Dolz Pérez I, O J, Ontoria-Oviedo I, Sepulveda P, Nebot V, et al. Two-Component Peptidic Molecular Gels for Topical Drug Delivery of Naproxen. ACS Applied Bio Materials. 2021;4. https://doi.org/10.1021/acsabm.0c01422.
-
2.
Shailaja AK, Afreen U. Formulation and Evaluation of Naproxen Sodium Loaded Invasomes for Topical Delivery. J Current Nanomedicine. 2022;12(1):32-43.
-
3.
Noreen S, Pervaiz F, Ashames A, Buabeid M, Fahelelbom K, Shoukat H, et al. Optimization of Novel Naproxen-Loaded Chitosan/Carrageenan Nanocarrier-Based Gel for Topical Delivery: Ex Vivo, Histopathological, and In Vivo Evaluation. Pharmaceuticals (Basel). 2021;14(6). [PubMed ID: 34207951]. [PubMed Central ID: PMC8230576]. https://doi.org/10.3390/ph14060557.
-
4.
Wiedersberg S, Guy RH. Transdermal drug delivery: 30+ years of war and still fighting!. J Control Release. 2014;190:150-6. [PubMed ID: 24852092]. https://doi.org/10.1016/j.jconrel.2014.05.022.
-
5.
Salimi A, Amirimoghadam S, Bagheri F. Preparation, Optimization, and Investigation of Naringenin-Loaded Microemulsion for Topical Application. Iran Biomed J. 2022;26(5):366-73. [PubMed ID: 36403103]. [PubMed Central ID: PMC9763875]. https://doi.org/10.52547/ibj.3722.
-
6.
Martí-Centelles R, Dolz-Pérez I, De la O J, Ontoria-Oviedo I, Sepúlveda P, Nebot VJ, et al. Two-component peptidic molecular gels for topical drug delivery of naproxen. J ACS Applied Bio Materials. 2021;4(1):935-44.
-
7.
Kajbafvala A, Salabat A, Salimi A. Formulation, characterization, and in vitro/ex vivo evaluation of quercetin-loaded microemulsion for topical application. Pharm Dev Technol. 2018;23(8):741-50. [PubMed ID: 27871215]. https://doi.org/10.1080/10837450.2016.1263995.
-
8.
Salimi A, Sharif Makhmal Zadeh B, Godazgari S, Rahdar A. Development and Evaluation of Azelaic Acid-Loaded Microemulsion for Transfollicular Drug Delivery Through Guinea Pig Skin: A Mechanistic Study. Adv Pharm Bull. 2020;10(2):239-46. [PubMed ID: 32373492]. [PubMed Central ID: PMC7191225]. https://doi.org/10.34172/apb.2020.028.
-
9.
Shukla T, Upmanyu N, Agrawal M, Saraf S, Saraf S, Alexander A. Biomedical applications of microemulsion through dermal and transdermal route. Biomed Pharmacother. 2018;108:1477-94. [PubMed ID: 30372850]. https://doi.org/10.1016/j.biopha.2018.10.021.
-
10.
Ghasemi K, Tasnim S, Mahmud S. PCM, nano/microencapsulation and slurries: A review of fundamentals, categories, fabrication, numerical models and applications. J Sustainable Energy Technologies Assessments. 2022;52:102084.
-
11.
Souto EB, Cano A, Martins-Gomes C, Coutinho TE, Zielinska A, Silva AM. Microemulsions and Nanoemulsions in Skin Drug Delivery. Bioengineering (Basel). 2022;9(4). [PubMed ID: 35447718]. [PubMed Central ID: PMC9028917]. https://doi.org/10.3390/bioengineering9040158.
-
12.
Das S, Lee SH, Chia VD, Chow PS, Macbeath C, Liu Y, et al. Development of microemulsion based topical ivermectin formulations: Pre-formulation and formulation studies. Colloids Surf B Biointerfaces. 2020;189:110823. [PubMed ID: 32036331]. https://doi.org/10.1016/j.colsurfb.2020.110823.
-
13.
Hu L, Hu Q, Yang J. Enhancement of transdermal delivery of ibuprofen using microemulsion vehicle. J Iran Basic Med Sci. 2014;17(10):760.
-
14.
Ita K. Progress in the use of microemulsions for transdermal and dermal drug delivery. Pharm Dev Technol. 2017;22(4):467-75. [PubMed ID: 26931453]. https://doi.org/10.3109/10837450.2016.1148722.
-
15.
Mehanna MM, Abla KK, Domiati S, Elmaradny H. Superiority of microemulsion-based hydrogel for non-steroidal anti-inflammatory drug transdermal delivery: a comparative safety and anti-nociceptive efficacy study. Int J Pharm. 2022;622:121830. [PubMed ID: 35589005]. https://doi.org/10.1016/j.ijpharm.2022.121830.
-
16.
Benbow T, Campbell J. Microemulsions as transdermal drug delivery systems for nonsteroidal anti-inflammatory drugs (NSAIDs): a literature review. Drug Dev Ind Pharm. 2019;45(12):1849-55. [PubMed ID: 31617433]. https://doi.org/10.1080/03639045.2019.1680996.
-
17.
Modan EM, PLĂIAȘU AG. Advantages and disadvantages of chemical methods in the elaboration of nanomaterials. J The Annals of “Dunarea de Jos” University of Galati. Fascicle IX, Metallurgy Materials Science. 2020;43(1):53-60.
-
18.
Ustundag Okur N, Yavasoglu A, Karasulu HY. Preparation and evaluation of microemulsion formulations of naproxen for dermal delivery. Chem Pharm Bull (Tokyo). 2014;62(2):135-43. [PubMed ID: 24492583]. https://doi.org/10.1248/cpb.c13-00051.
-
19.
Ustundag Okur N, Apaydin S, Karabay Yavasoglu NU, Yavasoglu A, Karasulu HY. Evaluation of skin permeation and anti-inflammatory and analgesic effects of new naproxen microemulsion formulations. Int J Pharm. 2011;416(1):136-44. [PubMed ID: 21723930]. https://doi.org/10.1016/j.ijpharm.2011.06.026.
-
20.
Salimi A, Hedayatipour N, Moghimipour E. The Effect of Various Vehicles on the Naproxen Permeability through Rat Skin: A Mechanistic Study by DSC and FT-IR Techniques. Adv Pharm Bull. 2016;6(1):9-16. [PubMed ID: 27099831]. [PubMed Central ID: PMC4833885]. https://doi.org/10.15171/apb.2016.03.
-
21.
Moghimipour E, Farsimadan N, Salimi A. Ocular Delivery of Quercetin Using Microemulsion System: Design, Characterization, and Ex-vivo Transcorneal Permeation. Iran J Pharm Res. 2022;21(1). e127486. [PubMed ID: 36945341]. [PubMed Central ID: PMC10024810]. https://doi.org/10.5812/ijpr-127486.
-
22.
Adamiak-Giera U, Nowak A, Duchnik W, Ossowicz-Rupniewska P, Czerkawska A, Machoy-Mokrzynska A, et al. Evaluation of the in vitro permeation parameters of topical ketoprofen and lidocaine hydrochloride from transdermal Pentravan((R)) products through human skin. Front Pharmacol. 2023;14:1157977. [PubMed ID: 37324484]. [PubMed Central ID: PMC10264579]. https://doi.org/10.3389/fphar.2023.1157977.
-
23.
Salimi A, Sheykholeslami S. The Effect of Herbal Penetration Enhancers on the Skin Permeability of Mefenamic Acid Through Rat Skin. Turk J Pharm Sci. 2023;20(2):108-14. [PubMed ID: 37161659]. [PubMed Central ID: PMC10176627]. https://doi.org/10.4274/tjps.galenos.2022.60669.
-
24.
Salimi A, Noorafrooz R, Fouladi M, Soleymanİ SM. Design and Assessment of a Microemulsion-Based Transdermal Drug Delivery System for Meloxicam; Examination of Formulation Ingredients. J Fabad Pharmaceutical Sci. 2023;48(3):359-72.
-
25.
Salimi A, Sharifmakhmalzadeh B, Amirabadi FS. Design and evaluation of adapalene microemulsion for transfollicular drug delivery through guinea pig skin. J Cosmet Dermatol. 2024;23(1):289-95. [PubMed ID: 37462297]. https://doi.org/10.1111/jocd.15933.
-
26.
Priya S, Koland M, Kumari S. Nanoemulsion components screening of quetiapine fumarate: Effect of surfactant and co surfactant. J Asian J Pharm Clin Res. 2015;8(6):136-40.
-
27.
Abd E, Benson HAE, Mohammed YH, Roberts MS, Grice JE. Permeation Mechanism of Caffeine and Naproxen through in vitro Human Epidermis: Effect of Vehicles and Penetration Enhancers. Skin Pharmacol Physiol. 2019;32(3):132-41. [PubMed ID: 30909278]. https://doi.org/10.1159/000497225.
-
28.
Suksaeree J, Simchareon W, Pichayakorn W. Effect of glycols permeation enhancer on the release and permeation of meloxicam-natural rubber film through pig skin. J Drug Delivery Sci Technol. 2021;66:102874.
-
29.
Lima MPD, Lopes EM, Gomes LDS, Franca ARS, Acha BT, Carvalho ALM, et al. Technological development of microemulsions with perspectives for pain treatment: a patent review. Expert Opin Ther Pat. 2018;28(9):691-702. [PubMed ID: 30175633]. https://doi.org/10.1080/13543776.2018.1519025.
-
30.
Arredondo-Ochoa T, Silva-Martínez GA. Microemulsion based nanostructures for drug delivery. J Frontiers in Nanotechnol. 2022;3:753947.
-
31.
Ha MW, Paek SM. Recent Advances in the Synthesis of Ibuprofen and Naproxen. Molecules. 2021;26(16). [PubMed ID: 34443379]. [PubMed Central ID: PMC8399189]. https://doi.org/10.3390/molecules26164792.
-
32.
Bajpai M, Sharma PK, Mittal A. A study of oleic acid oily base for the tropical delivery of dexamethasone microemulsion formulations. Asian J Pharmaceutics. 2009;3(3).
-
33.
Ng KW, Lau WM, Williams A. [Percutaneous Penetration Enhancers Chemical Methods in Penetration Enhancement]. German: Springer Berlin; 2015. Germany.
-
34.
Mohammad Soleymani S, Salimi A. Enhancement of Dermal Delivery of Finasteride Using Microemulsion Systems. Adv Pharm Bull. 2019;9(4):584-92. [PubMed ID: 31857962]. [PubMed Central ID: PMC6912190]. https://doi.org/10.15171/apb.2019.067.
-
35.
Atef B, Ishak RA, Badawy SS, Osman R. Exploring the potential of oleic acid in nanotechnology-mediated dermal drug delivery: An up-to-date review. J Drug Delivery Sci Technol. 2022;67:103032.