Abstract
Background:
Cardiac surgery-associated acute kidney injury (CSA-AKI) is a major adverse effect of cardiac surgery. The early detection of this complication can improve the quality of postoperative care and help prevent this phenomenon.Methods:
In this prospective descriptive-analytical study, 148 patients were enrolled, 107 of whom were selected for analysis between February and September 2019 in the Cardiac Surgery Unit of Golestan Hospital, Ahvaz, Iran. Kidney tissue oxygen saturation was measured at multiple definite times during surgery. Hemoglobin, blood urea nitrogen, creatinine, and lactate were measured during and 48 hours after the surgery.Results:
Forty-one patients were diagnosed with CSA-AKI according to the KDIGO criteria. Parametric and non-parametric analyses showed no significant difference between the CSA-AKI and non-CSA-AKI groups in the demographic parameters. Repeated measures ANOVA showed no significant difference in parameters, except for BUN. Repeated measures ANOVA showed a significant difference between both groups and time factors (P < 0.001, P = 0.0006, respectively). The ROC curve analyses showed that in a single point of time, the difference in the middle of CPB time from baseline had a high value in the prediction of AKI (AUC: 0.764; CI: 0.57 - 0.951).Conclusions:
Kidney saturation monitoring could be considered in cardiac surgery for the rapid detection of CSA-AKI. Although kidney tissue saturation is not correlated directly to the arterial oxygen saturation, the physician and the surgery team can predict the chance of acute kidney injury.Keywords
Near Infra-Red Spectroscopy Acute Kidney Injury Cardiac Surgery
1. Background
Cardiac surgery and its complications have negative impacts on kidney function and can lead to acute kidney injury, which is related to augmented complications and mortality (1). Multiple factors, including pre-, intra-, and post-cardiac surgery, make the kidney susceptible to injury, which is known as cardiac surgery-associated acute kidney injury (CSA-AKI) (2). There are more than 30 different diagnostic criteria for CSA-AKI such as RIFLE, KDIGO, AKIN, etc., resulting in different ranges of CSA-AKI incidence, up to 30% in the literature (3). Recently, new CSA-AKI markers such as neutrophil gelatinase-associated lipocalin (NGAL) have been discovered, but the common criteria for diagnosis are still based on the increase of plasma creatinine levels (4). Causes and pathways of CSA-AKI induction according to pathophysiology are different, including age, CPB time, cross-clamp time, type of operation, poisons, metabolic factors, ischemia, reperfusion injury, activation of neurohormonal factors, inflammation, and oxidative stress responses (5, 6). Numerous trials have been conducted on the improvement and confirmation of new predictor scales. However, all of the scales have had limitations.
The monitoring of kidney oxygen saturation based on near-infrared spectroscopy is a new approach in the diagnosis of CSA-AKI before the rise of creatinine (7). Near-infrared spectroscopy (NIRS) is a noninvasive approach for the detection of CSA-AKI, which can continuously measure the variance between oxygenated and deoxygenated hemoglobin in a local soft-tissue zone, and so obtaining the regional oxygen saturation (rSO2) (8). This technique is limited by its depth of penetration into the tissue. Interfering with factors like bilirubin, skin pigmentation, and peripheral light also affects its response (9). Near-infrared spectroscopy in open-heart surgery is routinely used for the monitoring of brain oxygen saturation (bSO2). The desaturation of the brain during surgery can be associated with an increased risk of cerebral dysfunction (10). Numerous studies have revealed an association between brain and somatic NIRS monitoring and mixed venous oxygen saturation (ScvO2) and local tissue perfusion (11, 12). Kidney oxygen desaturation during surgery can lead to postoperative AKI (13).
2. Objectives
This study aimed to investigate the effect of NIRS on the early diagnosis of CSA-AKI.
3. Methods
3.1. Study Design
This study was approved by the Anesthesiology and Pain Research Center, Ahvaz Jundishapur University of Medical Sciences (PAIN-9805), under the supervision of the AJUMS Research Ethics Committee (code: IR.AJUMS.REC.1398.097). A prospective descriptive-analytical study was accomplished at the Cardiac Surgery Unit of Golestan Hospital, Ahvaz, Iran, between February and September 2019. Written informed consent was signed by all patients. All procedures were performed according to the standard of care of the center. The inclusion criteria included patients aged between 30 and 75 years, candidates for on-pump cardiac surgery. The exclusion criteria included a history of renal disease, BMI > 35, EF < 30%, history of drug use that would directly affect the kidneys like NSAID, electrolyte disturbance, urgent surgery, and redo surgery. The CSA-AKI diagnosis was according to the KDIGO criteria (including an increase in SCr ≥ 0.3 mg/dL or greater within 48 hours or an increase in SCr ≥ 1.5 times of baseline within seven days after surgery) (3).
Standard monitoring, including five-lead electrocardiography, pulse oximetry, and arterial line for continuous blood pressure monitoring and blood gases, was done after arrival at the operating room. The NIRS sensors were attached to the right and left flank area. Before the induction of anesthesia, kSO2 was recorded as the baseline. A standard anesthesia technique was used for all patients. A central venous catheter was introduced after the induction of anesthesia. The cardiopulmonary bypass was started subsequently injection of 350 µ/kg of heparin for each patient. ACT 450 - 480 second was the goal of heparin dosage. Besides, CPB time and cross-clamp time were recorded. After the termination of CPB, heparin was reversed by protamine (1 mg/kg). The CPB, cardiac surgery, and postoperative management were the same for all patients.
The cardiovascular ICU admission was made for all patients after the surgery, and a standard protocol was used for sedation, analgesia (propofol 0.5 mg/kg/h and morphine sulfate 0.1 mg/kg/h), and mechanical ventilation (SIMV mode of ventilation). Patients were extubated when the weaning criteria emerged.
3.2. Study Patients
In this study, 148 patients were enrolled. According to the inclusion criteria, 107 patients were selected for analysis, and 41 patients were excluded and of them. In the follow-up stage, six patients were excluded owing to the lack of information, and finally, 101 patients were investigated.
3.3. Consort Diagram
3.3.1. Study Variables and Measurements
We recorded characteristic data such as age, sex, body mass index, history of diabetes mellitus, hypertension, left ventricle ejection fraction, Euro SCORE, kidney depth, baseline kSO2, preoperative hemoglobin, preoperative SCr, and estimated glomerular filtration rates. Postoperative parameters were evaluated on ICU admission and 6, 12, 24, and 48 hours postoperatively. The arterial blood gas was measured at all the measurement points (T0-T48) (Figure 1).
Consort diagram: Observational study
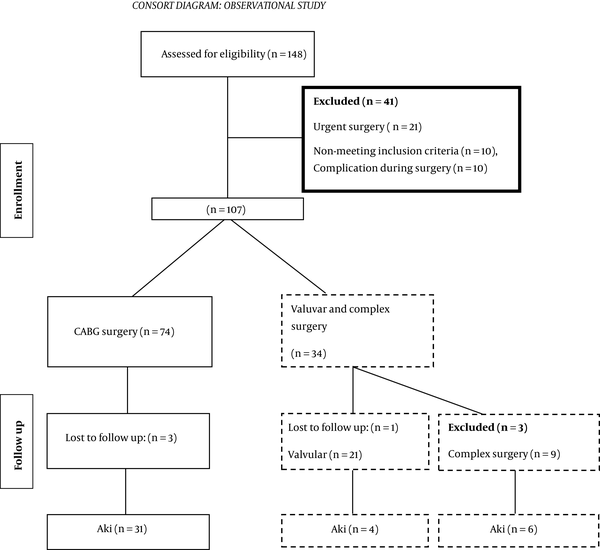
The main outcome was the occurrence of CSA-AKI after the surgery. The secondary outcomes included circulatory arrest, reoperation, sepsis, low cardiac output (cardiac index of < 2.2 mL/min/m2), pneumonia, hepatic dysfunction, prolonged mechanical ventilation time (PMV: mechanical ventilation time > 48 hours), massive transfusion (need for > 10 units of blood component), mortality, need for renal replacement therapy (RRT), and length of ICU stay.
The time points of kSO2 measurement were before the surgery (T0), after induction of anesthesia (T1), at the beginning of CPB (T2), during CPB (T3), after weaning off CPB (T4), and at ICU admission (T5). The left and right kidney saturation was measured at T0-T5 by NIRS (INVOS 5100C; Medtronic, Inc., Minneapolis, Minn). We assessed the correlation between kSO2 and CSA-AKI using the KIDGO criteria (14).
The sample size was calculated using the sample size estimation formula based on prevalence. The prevalence of CSA-AKI was 28.9% based on previous data (7). The study population was designated at 330 patients or whenever we reached at least 35 patients with AKI.
3.4. Statistical Analysis
The normality of all data was checked by the Kolmogorov-Smirnov (K-S) test, Shapiro-Wilk test, and D’Agostino skewness test (15). The parametric and non-parametric correlation was calculated between all study variables. The sensitivity and specificity of kSO2 were analyzed by AUC-ROC analysis. The time-course data were analyzed by RM-ANOVA and non-parametric RM analysis. The frequency of secondary outcomes was examined by the Fisher exact test.
4. Results
In this study, 41 (41.41%) patients developed CSA-AKI. The mean age was 60 (51 - 69), and 25 (60.97) patients were male. The demographics, comorbidity, and intraoperative data are listed in Table 1. We found a significant association between the CSA-AKI event and Euro SCOREII (P = 0.036), LVEF < 50%, CPB time (P < 0.001), cross-clamping time (P = 0.036), median MAP during CPB (P = 0.042), peak serum lactate ( P = 0.049), and valvular surgery (P = 0.017). A history of smoking, diabetes mellitus, hypertension, and dyslipidemia were associated with an increased risk of CSA-AKI (P < 0.001) (Table 1).
Demographic Data and Operative Characteristicsa
Variables | Total (N = 101) | AKI (N = 41) | Non-AKI (N = 70) | P-Value |
---|---|---|---|---|
Age, y | 59 (52 - 67) | 60 (51 - 69) | 58 (54 - 64) | 0.978 |
Male | 70 (69.30) | 25 (60.97) | 45(64.28) | 0.838 |
BMI, kg/m2 | 27.9 ± 3.5) | 30 ± 4.3 | 27.51 ± 3.2) | 0.199 |
Euro SCOREII | 1.3 (0.8 - 2) | 1.96 (1 - 2.3) | 0.95(0.8 - 2) | 0.036b |
Comorbidities | ||||
Smoking | 53 (52.47) | 37 (90.24) | 16 (22.87) | < 0.001b |
Hypertension | 45 (44.54) | 36 (87.8) | 9 (12.86) | < 0.001b |
Diabetes mellitus | 56 (55.45) | 31 (75.61) | 25 (35.71) | < 0.001b |
Dyslipidemia | 68 (67.32) | 28 (68.29) | 30 (49.86) | 0.011b |
Left ventricular dysfunction (LVEF< 50%) | 47 (46.53) | 32 (82.05) | 15 (21.43) | < 0.001b |
Intraoperative data | ||||
CPB time, min | 105.42 ± 20.13 | 135 ± 15.45 | 75.15 ± 15 | < 0.001b |
Cross clamping time | 80.21 ± 17.11) | 97.11 ± 12.14 | 72.32 ± 4.32 | 0.036b |
MAP during CPB | 65.32 ± 15.14 | 54.21 ± 15.15 | 65.64 ± 10.08 | 0.042b |
Peak Lactate level, mg/dL | 40.51 ± 12.11 | 10.75 ± 4.31 | 4.15 ± 3.12 | 0.049b |
CABG | 71 (90.62) | 31 (75.61) | 40 (57.11) | 0.065 |
Valve surgery | 21 (21.09) | 4 (9.76) | 21 (30) | 0.017b |
CABG + valve | 9 (8.91) | 6 (14.63) | 3 (4.23) | 0.071 |
4.1. Kidney Saturation Analysis
The trend of changes in right kidney saturation had no significant difference between the two groups (P = 0.102). The trend of changes in left kidney saturation had no significant differences between the two groups (P = 0.089). The trend of changes on one side of kidney saturation with SpaO2 had a significant difference between the two groups (P < 0.001). The greatest reduction in kSO2 was at T3, which was equivalent to the CPB time. We observed decreases in KSaO2 among cardiac surgery, but in patients with AKI significantly greatest in T3 point (Figure 2).
The trend of changes in kidney saturation by NIRS in the study
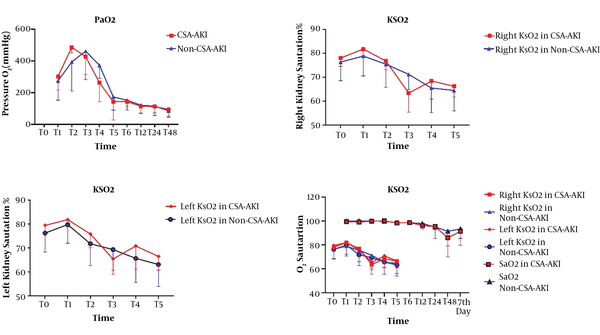
4.2. Hemoglobin, BUN, and Creatinine Analysis
The differences were significant between the two groups in the trend of changes in Hb (Figure 3A), SCr (Figure 3B), and BUN (Figure 3C) (P < 0.001, P < 0.001, P < 0.001, respectively). The highest increase in BUN and creatinine level were at T48, and the lowest hemoglobin level was at this time.
The trend of changes in hemoglobin, creatinine, and blood urea nitrogen in the study
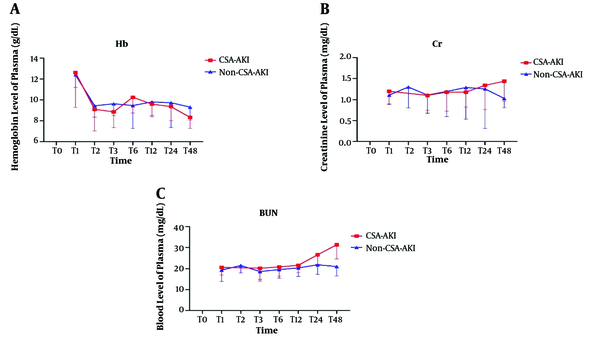
4.3. AUC-ROC Curve Analysis
The ROC curve analysis difference of kSO2 in the middle time of CPB from baseline (Figure 4C) could predict the risk of CSA-AKI (AUC-ROC, 0.914; CI, 0.863 - 0.965; P < 0.001).
AUC-ROC curve at different times from baseline
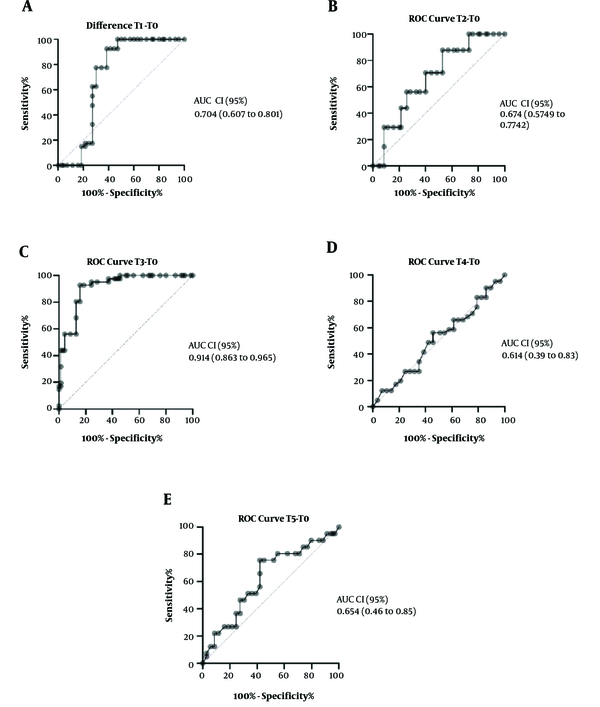
4.4. Secondary Outcomes
The results showed that the secondary outcomes were worse in patients with CSA-AKI, including reoperation (P = 0.009), hepatic dysfunction (P = 0.031), massive transfusion (P = 0.032), Renal Replacement Therapy (RRT) (P = 0.005), and ICU stay (P < 0.0001).
5. Discussion
The early diagnosis of cardiac surgery-associated acute kidney injury by current diagnostic methods is not feasible nor cost-effective. The traditional type of CSA-AKI diagnosis is based on the creatinine rise, which is not distinguishable at the golden time of treatment. A fast and plausible approach in CSA-AKI could save the life of many cardiac surgery patients. Recently, measuring kidney tissue oxygen saturation via NIRS has been suggested as a novel and noninvasive method (7, 16-19). Although previous studies showed the significant application of NIRS in monitoring during cardiac surgery, a practical approach with which the anesthesiologist can clinically make a decision and definite diagnosis is not clear. Focusing on the definite time based on the knowledge of pathophysiology, CSA-AKI can be a diagnostic approach. The measurement times that were chosen in this study were based on the pathophysiological point that had a role in CSA-AKI induction.
This study showed that kidney oxygen saturation monitoring has a high value in the diagnosis of CSA-AKI. In this study, T1 was chosen as a definite point of time because it was the induction time, T2 was at CPB onset, T3 was during CPB, T4 was at the end of CPB, and T5 was at ICU admission, which were chosen based on the pathophysiology of CSA-AKI (5). Although many pathways for CSA-AKI induction are suggested, our conservative inclusion and exclusion criteria reduced confounding variables in this study, such as drug regimen changes and drugs that directly affected the kidneys like NSAID and acid and base disturbances. One of the valuable findings of this study was the definition of a sensitive and specific point for the measurement of kidney saturation. This clinical point was during CPB time. The difference of T3 from baseline could be a good index in diagnosing CSA-AKI.
A study done in 2018 by Ortega-Loubon et al. (7) showed that a 20% decrease of NIRS from baseline could be considered a good index for CSA-AKI diagnosis. However, decreases in kSO2 in our study were not like the mentioned study due to the single-point measurement of kSO2, a difference of T3 from baseline confirmed the Ortega-Loubon et al. (7) study, and have high sensitivity of dismissal in distinguishing of CSA-AKI. As in the Ortega-Loubon et al. (7) study, brain tissue saturation had no relationship with kidney tissue saturation and is the reason why we did not assess brain oxygen saturation bought blood oxygen saturation also has not relation with kSo2. The only aspect that differentiates our study from the study by Ortega-Loubon et al. (7) is that we looked for decreases from baseline at a definite time and evidence of acute kidney injury. That’s meant to evaluate to factor simultaneously. The reperfusion phase after ischemia causes increases in tissue oxygen saturation, but this is an effective factor in AKI. As we showed, the middle of CPB time is suitable, according to ischemia-reperfusion. However, it is not a pharmacologically approved treatment for CSA-AKI, evidence of the effectiveness of dexmedetomidine, vasopressin, and some amino acid suggested (3). Each drug-affected oxidative stress and inflammation pathway, for example, dexmedetomidine in Inhibit jak/stat phosphorylation (20).
The result of this study showed that older age (although insignificantly) and prolonged CPB time were correlated with postoperative CSA-AKI, indicating that its occurrence is multifactorial (21). Age is an independent risk factor for this complication (22).
CPB causes the activation of inflammatory factors and produces vasomotor changes in the kidney. Therefore, as the cardiopulmonary bypass time is longer, more inflammatory reactions will be expected (23). Moreover, the effect of the type of operation on the occurrence of CSA-AKI is clear. Valve surgery and complex surgery are associated with an increased risk of this complication. This fact was provided by this study (21).
The probability of CSA-AKI is between 7% and 40% based on previous studies, while it was 41.41% in our study (24, 25). These findings are comparable to the presently reported data, and this may be due to establishing sufficient perfusion pressure and preventing the use of nephrotoxic drugs. Because serum creatinine can peak in up to seven days after the surgery (rendering to the KDIGO criteria for CSA-AKI) and the measurements of NIRS were done only during the surgery, the measurements of kSO2 provided limited duration to take quick responses. The main benefit of this new noninvasive technique is the rapid and continuous response, and this is the greatest benefit of NIRS. Thus, this can help prevent postoperative CSA-AKI (13).
As shown in Table 2, patients with CSA-AKI had higher rates of reoperation (P = 0.009), hepatic dysfunction (P = 0.031), massive transfusion (P = 0.032), RRT (P = 0.005), and ICU stay (P < 0.0001). These complications may be due to ischemia and reperfusion injury, and which can cause CSA-AKI. These results were similar to those obtained by Ortega-Loubon et al. (7).
Postoperative Outcomes Based on the Presence of Acute Kidney Injury
Variables | Total, No. (%) | Non-AKI (N = 70), No. (%) | AKI (N = 41), No. (%) | P-Value |
---|---|---|---|---|
Reoperation | 20 (19.80) | 7 (10) | 13 (31.71) | 0.009a |
Delirium | 9 (8.91) | 3 (6.29) | 6 (14.63) | 0.073 |
Sepsis | 7 (6.93) | 2 (2.86) | 5 (12.20) | 0.098 |
Infection | 7 (6.93) | 2 (2.86) | 5 (12.20) | 0.098 |
Dysrhythmia | 9 (8.9) | 5 (7.14) | 4 (9.76) | 0.723 |
Circulatory arrest | 4 (3.96) | 2 (2.86) | 2 (4.88) | 0.625 |
Low cardiac output | 12 (11.88) | 7 (12.20) | 5 (10) | 0.757 |
Pneumonia | 1 (0.99) | 0 | 1 (2.4) | 0.369 |
PMV | 11 (10.89) | 2 (2.86) | 9 (21.95) | 0.002a |
Hepatic dysfunction | 18 (17.82) | 7 (10) | 11 (26.83) | 0.031a |
Massive transfusion | 41 (40.59) | 16 (22.86) | 18 (43.90) | 0.032a |
RRT | 5 (12.2) | 5 (12.2) | 0 | 0.005a |
30-d mortality | 10 (9.9) | 5 (7.14) | 5 (12.20) | 0.494 |
ICU stay, median (IQR) | 7 (3 - 12) | 3 (2 - 5) | 12 (10 - 15) | < 0.0001a |
In multiple animal studies that induced ischemia-reperfusion, many factors were involved, such as oxidative stress and inflammation pathways (26). The activation of pro-inflammatory and inflammatory cytokines like TNF-α (27), IL-6 (28), and JAK/STAT (29), and phosphorylation pathways (30, 31) are the fundamental reasons for the development of CSA-AKI. Kidney saturation by NIRS to use antioxidant agents could be considered a future method in the prevention of CSA-AKI.
5.1. Study Limitations
As a major limitation of this study, we needed longer periods of observation and multivariate analysis (cohort study) to omit biases. We suggest a large multicenter study with a heterogeneous population. The second limitation was the lack of NIRS monitoring in the ICU. It is also recommended for further study.
5.2. Conclusions
Kidney saturation monitoring may be considered in cardiac surgery for the rapid detection of CSA-AKI. Although kidney tissue saturation is not correlated directly to the arterial oxygen saturation, the physician and the surgery team can predict the chance of AKI.
Acknowledgements
References
-
1.
Wang Y, Bellomo R. Cardiac surgery-associated acute kidney injury: risk factors, pathophysiology and treatment. Nat Rev Nephrol. 2017;13(11):697-711. [PubMed ID: 28869251]. https://doi.org/10.1038/nrneph.2017.119.
-
2.
Shahbazi S, Alishahi P, Asadpour E. Evaluation of the Effect of Aminophylline in Reducing the Incidence of Acute Kidney Injury After Cardiac Surgery. Anesth Pain Med. 2017;7(4). e21740. [PubMed ID: 29696106]. [PubMed Central ID: PMC5903222]. https://doi.org/10.5812/aapm.21740.
-
3.
Vives M, Hernandez A, Parramon F, Estanyol N, Pardina B, Munoz A, et al. Acute kidney injury after cardiac surgery: prevalence, impact and management challenges. Int J Nephrol Renovasc Dis. 2019;12:153-66. [PubMed ID: 31303781]. [PubMed Central ID: PMC6612286]. https://doi.org/10.2147/IJNRD.S167477.
-
4.
Hoste EA, Cruz DN, Davenport A, Mehta RL, Piccinni P, Tetta C, et al. The epidemiology of cardiac surgery-associated acute kidney injury. Int J Artif Organs. 2008;31(2):158-65. [PubMed ID: 18311732]. https://doi.org/10.1177/039139880803100209.
-
5.
Bellomo R, Auriemma S, Fabbri A, D'Onofrio A, Katz N, McCullough PA, et al. The pathophysiology of cardiac surgery-associated acute kidney injury (CSA-AKI). Int J Artif Organs. 2008;31(2):166-78. [PubMed ID: 18311733]. https://doi.org/10.1177/039139880803100210.
-
6.
Javaherforoosh Zadeh F, Moadeli M, Soltanzadeh M, Janatmakan F. Effect of Remote Ischemic Preconditioning on Troponin I in CABG. Anesth Pain Med. 2017;7(4). e12549. [PubMed ID: 29430406]. [PubMed Central ID: PMC5797663]. https://doi.org/10.5812/aapm.12549.
-
7.
Ortega-Loubon C, Fernandez-Molina M, Fierro I, Jorge-Monjas P, Carrascal Y, Gomez-Herreras JI, et al. Postoperative kidney oxygen saturation as a novel marker for acute kidney injury after adult cardiac surgery. J Thorac Cardiovasc Surg. 2019;157(6):2340-2351 e3. [PubMed ID: 30459107]. https://doi.org/10.1016/j.jtcvs.2018.09.115.
-
8.
Buratto E, Horton S, Konstantinov IE. So near, yet so far: Is isolated cerebral near-infrared spectroscopy in neonates nearly as useful as it is noninvasive? J Thorac Cardiovasc Surg. 2017;154(3):1054-5. [PubMed ID: 28549695]. https://doi.org/10.1016/j.jtcvs.2017.04.061.
-
9.
Biedrzycka A, Lango R. Tissue oximetry in anaesthesia and intensive care. Anaesthesiol Intensive Ther. 2016;48(1):41-8. [PubMed ID: 26966109]. https://doi.org/10.5603/AIT.2016.0005.
-
10.
Slater JP, Guarino T, Stack J, Vinod K, Bustami RT, Brown J3, et al. Cerebral oxygen desaturation predicts cognitive decline and longer hospital stay after cardiac surgery. Ann Thorac Surg. 2009;87(1):36-44. discussion 44-5. [PubMed ID: 19101265]. https://doi.org/10.1016/j.athoracsur.2008.08.070.
-
11.
Murkin JM, Arango M. Near-infrared spectroscopy as an index of brain and tissue oxygenation. Br J Anaesth. 2009;103 Suppl 1:i3-13. [PubMed ID: 20007987]. https://doi.org/10.1093/bja/aep299.
-
12.
Hanson SJ, Berens RJ, Havens PL, Kim MK, Hoffman GM. Effect of volume resuscitation on regional perfusion in dehydrated pediatric patients as measured by two-site near-infrared spectroscopy. Pediatr Emerg Care. 2009;25(3):150-3. [PubMed ID: 19262423]. https://doi.org/10.1097/PEC.0b013e31819a7f60.
-
13.
Choi DK, Kim WJ, Chin JH, Lee EH, Don Hahm K, Yeon Sim J, et al. Intraoperative renal regional oxygen desaturation can be a predictor for acute kidney injury after cardiac surgery. J Cardiothorac Vasc Anesth. 2014;28(3):564-71. [PubMed ID: 24702816]. https://doi.org/10.1053/j.jvca.2013.12.005.
-
14.
Abadeer AI, Kurlansky P, Chiuzan C, Truby L, Radhakrishnan J, Garan R, et al. Importance of stratifying acute kidney injury in cardiogenic shock resuscitated with mechanical circulatory support therapy. J Thorac Cardiovasc Surg. 2017;154(3):856-864 e4. [PubMed ID: 28554672]. https://doi.org/10.1016/j.jtcvs.2017.04.042.
-
15.
Ghasemi A, Zahediasl S. Normality tests for statistical analysis: a guide for non-statisticians. Int J Endocrinol Metab. 2012;10(2):486-9. [PubMed ID: 23843808]. [PubMed Central ID: PMC3693611]. https://doi.org/10.5812/ijem.3505.
-
16.
Ortega-Loubon C, Fernandez-Molina M, Jorge-Monjas P, Fierro I, Herrera-Calvo G, Tamayo E. The Relevance of Renal Oxygen Saturation Over Other Markers in Cardiac Surgery-Associated Acute Kidney Injury. J Cardiothorac Vasc Anesth. 2019;33(10):2622-3. [PubMed ID: 30377051]. https://doi.org/10.1053/j.jvca.2018.09.031.
-
17.
Ortega-Loubon C, Fierro I, Tamayo E. Should kidney oxygen saturation be monitored to prevent acute kidney injury after adult cardiac surgery? J Thorac Cardiovasc Surg. 2019;158(3):e105-6. [PubMed ID: 31208801]. https://doi.org/10.1016/j.jtcvs.2019.05.012.
-
18.
Ortega-Loubon C, Herrera-Gomez F, Bernuy-Guevara C, Jorge-Monjas P, Ochoa-Sangrador C, Bustamante-Munguira J, et al. Near-Infrared Spectroscopy Monitoring in Cardiac and Noncardiac Surgery: Pairwise and Network Meta-Analyses. J Clin Med. 2019;8(12). [PubMed ID: 31847312]. [PubMed Central ID: PMC6947303]. https://doi.org/10.3390/jcm8122208.
-
19.
Ortega-Loubon C, Jorge-Monjas P, Tamayo E. Acute kidney injury after adult cardiac surgery: Trying to find the magic gun. J Thorac Cardiovasc Surg. 2019. [PubMed ID: 31280892]. https://doi.org/10.1016/j.jtcvs.2019.05.056.
-
20.
Gu J, Sun P, Zhao H, Watts HR, Sanders RD, Terrando N, et al. Dexmedetomidine provides renoprotection against ischemia-reperfusion injury in mice. Crit Care. 2011;15(3):R153. [PubMed ID: 21702944]. [PubMed Central ID: PMC3219027]. https://doi.org/10.1186/cc10283.
-
21.
Saydy N, Mazine A, Stevens LM, Jeamart H, Demers P, Page P, et al. Differences and similarities in risk factors for postoperative acute kidney injury between younger and older adults undergoing cardiac surgery. J Thorac Cardiovasc Surg. 2018;155(1):256-65. [PubMed ID: 28942979]. https://doi.org/10.1016/j.jtcvs.2017.08.039.
-
22.
Chen SW, Chang CH, Fan PC, Chen YC, Chu PH, Chen TH, et al. Comparison of contemporary preoperative risk models at predicting acute kidney injury after isolated coronary artery bypass grafting: a retrospective cohort study. BMJ Open. 2016;6(6). e010176. [PubMed ID: 27354068]. [PubMed Central ID: PMC4932284]. https://doi.org/10.1136/bmjopen-2015-010176.
-
23.
Jorge-Monjas P, Bustamante-Munguira J, Lorenzo M, Heredia-Rodriguez M, Fierro I, Gomez-Sanchez E, et al. Predicting cardiac surgery-associated acute kidney injury: The CRATE score. J Crit Care. 2016;31(1):130-8. [PubMed ID: 26700607]. https://doi.org/10.1016/j.jcrc.2015.11.004.
-
24.
Machado MN, Nakazone MA, Maia LN. Prognostic value of acute kidney injury after cardiac surgery according to kidney disease: improving global outcomes definition and staging (KDIGO) criteria. PLoS One. 2014;9(5). e98028. [PubMed ID: 24826910]. [PubMed Central ID: PMC4020924]. https://doi.org/10.1371/journal.pone.0098028.
-
25.
Zou H, Hong Q, Xu G. Early versus late initiation of renal replacement therapy impacts mortality in patients with acute kidney injury post cardiac surgery: a meta-analysis. Crit Care. 2017;21(1):150. [PubMed ID: 28623953]. [PubMed Central ID: PMC5474059]. https://doi.org/10.1186/s13054-017-1707-0.
-
26.
Furuichi K, Wada T, Yokoyama H, Kobayashi KI. Role of Cytokines and Chemokines in Renal Ischemia-Reperfusion Injury. Drug News Perspect. 2002;15(8):477-82. [PubMed ID: 12677185]. https://doi.org/10.1358/dnp.2002.15.8.840067.
-
27.
Donnahoo KK, Shames BD, Harken AH, Meldrum DR. Review article: the role of tumor necrosis factor in renal ischemia-reperfusion injury. J Urol. 1999;162(1):196-203. [PubMed ID: 10379787]. https://doi.org/10.1097/00005392-199907000-00068.
-
28.
Patel NS, Chatterjee PK, Di Paola R, Mazzon E, Britti D, De Sarro A, et al. Endogenous interleukin-6 enhances the renal injury, dysfunction, and inflammation caused by ischemia/reperfusion. J Pharmacol Exp Ther. 2005;312(3):1170-8. [PubMed ID: 15572648]. https://doi.org/10.1124/jpet.104.078659.
-
29.
Yang N, Luo M, Li R, Huang Y, Zhang R, Wu Q, et al. Blockage of JAK/STAT signalling attenuates renal ischaemia-reperfusion injury in rat. Nephrol Dial Transplant. 2008;23(1):91-100. [PubMed ID: 17670769]. https://doi.org/10.1093/ndt/gfm509.
-
30.
Jang HR, Ko GJ, Wasowska BA, Rabb H. The interaction between ischemia-reperfusion and immune responses in the kidney. J Mol Med (Berl). 2009;87(9):859-64. [PubMed ID: 19562316]. https://doi.org/10.1007/s00109-009-0491-y.
-
31.
Stroo I, Stokman G, Teske GJ, Raven A, Butter LM, Florquin S, et al. Chemokine expression in renal ischemia/reperfusion injury is most profound during the reparative phase. Int Immunol. 2010;22(6):433-42. [PubMed ID: 20410256]. [PubMed Central ID: PMC2877810]. https://doi.org/10.1093/intimm/dxq025.