Abstract
Background:
Mitochondrial ATP-sensitive potassium (mKATP) channels play a role in reperfusion arrhythmias (RAs) in ischemia-reperfusion (I/R) injury. Evidence suggests that remote ischemic preconditioning (RIPC) reduces RAs, however not much is known on the mechanistic role of mKATP in RIPC. We evaluated whether mKATP channels are associated with reducing arrhythmia and infarct size in RIPC.Methods:
Isolated rat hearts received 30 minutes of regional ischemia followed by 2 hours of reperfusion through the Langendorff perfusion system. RIPC was induced by 3 cycles of 5 minutes occlusion and 5 minutes release of the bilateral femoral artery. The animals were randomly divided into 4 groups as follows: 1) CON, I/R injury but not RIPC, 2) RIPC, 3) HD+RIPC, pretreatment of the selective mKATP channel blocker, 5-hydroxydecanoate (5-HD), in RIPC, and 4) HD, pretreatment of 5-HD in CON. Cardiodynamics and infarct size were determined. The severity of arrhythmia was quantitated via the Curtis and Walker scoring system as well as the Lepran scoring system.Results:
RIPC significantly reduced the infarct size over AR (25.7 ± 2.6%) compared to CON (37.0 ± 2.6%, P < 0.05). The selective mKATP channel blocker 5-HD significantly inhibited the infarct-reducing effect of RIPC (39.3 ± 3.0%, P < 0.05 vs. RIPC). Additionally, RIPC significantly reduced the arrhythmia score compared to CON (14.6 ± 1.9 to 8.7 ± 0.4, P = 0.023, by Curtis and Walker’s system, 16.1 ± 2.1 to 9.1 ± 0.5, P = 0.006, by Lepran’s system). The anti-arrhythmic effect of RIPC was blocked by 5-HD (15.5 ± 1.6 and 16.0 ± 1.2, by Curtis and Walker’s and Lepran’s system, respectively).Conclusions:
The selective mKATP channel blocker, 5-HD, inhibited the infarct-limitation and anti-arrhythmic effect of RIPC. The mKATP channels play a role in the reduction of both infarct size and RAs in RIPC.Keywords
Arrhythmia Ischemic Preconditioning Potassium Channel Reperfusion Injury
1. Background
Reperfusion injury is defined as cellular death or dysfunction occurring during restoration of blood supply to ischemic tissues. Reperfusion injury includes lethal reperfusion injury (cell death), nonlethal reperfusion injury (myocardial stunning), vascular injury ('no-reflow' phenomenon), and reperfusion-induced arrhythmias (RAs) (1). Early restoration of coronary perfusion (reperfusion) is the most important strategy to reduce myocardial infarction and attenuate RAs, such as ventricular tachycardia (VT) and ventricular fibrillation (VF) in acute myocardial infarction.
RAs originate as a result of the cellular and humoral reactions and can occur within seconds of restoration of blood flow to previously ischemic myocardium. RAs, one of the most important features of myocardial ischemia/reperfusion injury, can cause hemodynamic deterioration, decreased cardiac output, decreased coronary blood flow, and sometimes cardiac arrest (2).
The mitochondrial ATP-sensitive potassium (mKATP) channel has been studied extensively since its discovery in 1991. Based on previous research, this channel is believed to play a major role in ischemic preconditioning. However, three hypotheses have emerged to explain the link between mKATP channel opening and cardioprotection: a decrease in the mitochondrial Ca2+ uptake, swelling of the mitochondrial matrix and changes in ATP synthesis, and changes in the levels of reactive oxygen species (3).
The mKATP channels also reportedly play a role in RAs in ischemia-reperfusion (I/R) injury (4, 5). The mKATP channel opener, diazoxide, significantly reduces the frequency and duration of ventricular tachycardia (VT) episodes in isolated rat hearts (6). Pretreatment with the selective mKATP channel blocker, 5-hydroxydecanoate (5-HD), inhibits the beneficial effects of ischemic preconditioning on RAs, demonstrating the essential role of mKATP channels (1). It has been shown, however, that glibenclambide, a nonselective KATP channel inhibitor, does not inhibit the late preconditioning-induced reduction of arrhythmia in conscious sheep (7). Additionally, Dow et al. (8) demonstrated that the RAs due to ischemic postconditioning were not dependent on mKATP channels. However, there is little supportive data on the role of mKATP channels in remote conditionings.
Meanwhile, there is evidence that remote ischemic preconditioning (RIPC) reduces RAs. Oxman et al. (9) have demonstrated that RIPC protects against reperfusion tachyarrhythmia in isolated Langendorff-perfused rat hearts via norepinephrine release. Dow et al. (10) have also demonstrated that acute RIPC of the lower limbs reduces ventricular arrhythmias in open chest rat hearts but does not activate the reperfusion injury salvage kinase (RISK) pathway.
However, the relationship between RIPC and mKATP is unclear. The few previous studies on the cardioprotective role of mKATP channels in RIPC were mostly performed on extracardiac organs or focused on the infarct size (11).
We evaluated whether mKATP channels are associated with reducing both infarct size and RA in RIPC. We examined the infarct size, as well as the arrhythmia score, with the selective mKATP channel blocker 5-HD in RIPC-induced rat hearts.
2. Methods
Animal preparation and study procedures were performed according to the international guiding principles for biomedical research involving animals. The study process and protocols were approved by the institutional animal care and use committee.
This in vivo and in vitro experimental study was conducted between October 2015 and April 2016 at the institute of cardiovascular research, Pusan University, Yangsan hospital.
2.1. Surgical Procedure
Male Sprague-Dawley rats (mean body weight 350 g) obtained from Koatech Corporation (Cheongwon-gun, Republic of Korea) were used. One week before the experiment, the animals were acclimatized in the animal facility at a room temperature of 21°C with a 12 hours light/dark cycle. They had free access to standard rat food pellets and tap water. Animals were anesthetized with intraperitoneal S-ketamine (150 mg/kg). A tracheostomy was performed and initiated artificial ventilation using a rodent ventilator (model CIV-101, Columbus Instruments, Havard Apparatus, MA, USA) with 95% O2 mixed with 5% CO2 (tidal volume 1 mL/100 mg, respiratory rate 45 - 60/min). Arterial pH, PCO2, and PO2 were measured via the left carotid artery. The tidal volume and respiratory rate were adjusted in order to maintain normal physiological conditions. Body temperature was maintained at 38 ± 1°C using a thermostatically controlled heating mat. All rats were allowed to stabilize for at least 10 minutes after anesthesia.
2.2. Experimental Protocol
To investigate the anti-arrhythmic effect and the involvement of mKATP channels on the incidence and duration of RAs in RIPC, animals were randomly divided into 4 groups according to a computer-generated random table as follows: 1) CON (n = 8), I/R injury hearts but not subjected to RIPC, 2) RIPC (n = 9), remote ischemic preconditioning, 3) HD + RIPC (n = 8), pretreatment of a selective mKATP channel blocker 5-HD in RIPC group, and 4) HD (n = 8), pretreatment of 5-HD in CON group (Figure 1).
Experimental Protocols
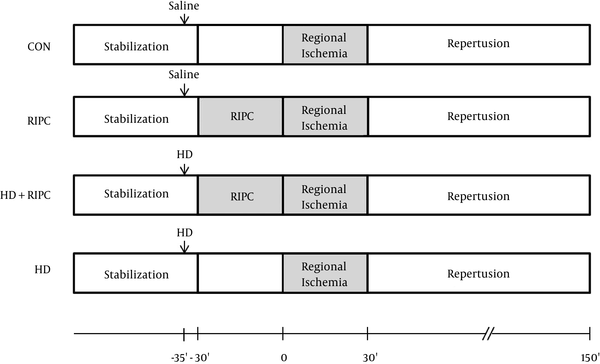
RIPC was induced by 3 cycles of 5 minutes occlusion and 5 minutes release of the bilateral femoral artery. The 5-HD (10 mg/kg) was pretreated 5 minutes before RIPC via the tail vein. CON and RIPC groups were administered 0.9% saline instead of 5-HD.
2.3. Langendorff Perfused Heart Isolation
The heart was removed on completion of RIPC and was connected to the Langendorff system via the aorta within 30 s of excision. Hearts were perfused with modified Krebs-Henseleit (KH) buffer containing (in mM) 118.5 of NaCl, 4.7 of KCl, 1.2 of MgSO4, 1.8 of CaCl2, 24.8 of NaHCO3, 1.2 of KH2PO4, and 10 of glucose (12). Hearts were subjected to 30 minutes of regional ischemia by making a snare at the proximal area of the left coronary artery and 2 h of reperfusion by releasing the snare (Figure 1).
2.4. Assessment of Cardiac Function
In an isolated, air-bubble free heart, a KH buffer-filled latex balloon was inserted into the left ventricle through incision of the left atrial appendage. The balloon volume was regulated to provide a left ventricular end-diastolic pressure (LVEDP) of 5 - 10 mmHg using the BIOPAC system (BIOPAC Systems Inc., Goleta, CA, USA). The left ventricular developed pressure (LVDP) was regarded as the difference between left ventricular systolic pressure (LVSP) and LVEDP. Heart rate (HR) and LV pressure were continuously recorded.
The researcher, who was blinded to the group assignment, measured and recorded the cardiac function, arrhythmia score, and infarction size of the heart.
2.5. Evaluation of Arrhythmia
RAs were evaluated by a representative left ventricle (LV) pressure wave form, according to Gong’s et al. (2). A discrete and identifiable premature QRS complex was diagnosed as premature ventricular contractions (PVCs). A run of 4 or more PVCs was identified as VT. VF was defined as a ventricular rhythm without a recognizable QRS complex and thus an immeasurable HR. The severity of arrhythmia during the early 30 minutes reperfusion (3 minutes intervals with 10 time points) that occurred in each individual heart was evaluated based on the type and duration of arrhythmic episodes.
RAs were quantitated via 2 modified scoring systems previously approved by Curtis and Walker (13) and Lepran et al. (14) (Table 1).
Two Arrhythmia Scoring Systems
Score | Curtis and Walker | Lepran |
---|---|---|
0 | <10 PVC | No arrhythmia |
1 | 10 - 50 PVC | ≤ 10 s VT or other arrhythmia, no VF |
2 | > 50 PVC | 11 - 30 s VT or other arrhythmia, no VF |
3 | 1 VF | 31 - 90 s VT or other arrhythmia, no VF |
4 | 2 - 4 VF | 91 - 180 s VT or other arrhythmia, and/or < 10 s reversible VF |
5 | > 4 VF | > 180 s VT or other arrhythmia, and/or >10 s reversible VF |
6 | Irreversible VF |
2.6. Determination of Area at Risk and Infarct Size
After 2 hours of reperfusion, the snare was retightened and a fluorescent polymer microsphere was injected into the demarcated non-ischemic region and the area at risk (AR). The hearts were separated from the Langendorff apparatus, drained, and weighed. They were then frozen at -20°C for 3 hr. The hearts were cut into 2 mm thick transverse slices using an acrylic rodent heart slicer matrix (Zivic Instruments, Pittsburgh, PA, USA). The slices of LV were incubated in TTC at 37°C for 20 minutes and subsequently fixed in 10% formalin. The LV was differentiated and removed from remaining tissue. The regions of the area of necrosis (AN) and the area of risk (AR) were quantified using UTHSCSA Image Tool (University of Texas Health Science Center, San Antonio, TX). The areas were converted into volumes by multiplying the areas by the slice thickness (2 mm). The AN volume was expressed as a percentage of the AR volume (AN/AR).
2.7. Statistical Analysis
Data were expressed as mean ± SEM. To the check the normality, the Kolmogorov-Smirnov test was used. In cases with a normal distribution, the t-test and one-way analysis of variance were used, whereas the Mann-Whitney and Kruskal-Wallis tests were used in cases without a normal distribution. Data analysis was performed using SPSS (SPSS for Windows, version 21.0, IBM, Armonk, NY, USA). Differences were considered as statistically significant when P values were < 0.05.
3. Results
A total of 36 rat hearts were assigned to the study. Three hearts were excluded due to having less than 250 beats per minute of HR (n = 2) and less than 80 mmHg of LVDP (n = 1) after the stabilization. Therefore, 33 hearts successfully completed the study, in which the infarct size and the arrhythmia score were analyzed.
3.1. Morphometric Analysis and Measurement of Infarct Size
There were no significant differences in body weight, heart weight, LV volume, AR volume, and AR to LV ratio among the groups (Table 2).
Group | BW, gm | HW, gm | LV volume, cm3 | AR volume, cm3 | AR/LV, % |
---|---|---|---|---|---|
CON (n = 8) | 302.5 ± 6.7 | 1.57 ± 0.02 | 0.785 ± 0.034 | 0.441 ± 0.030 | 56.2 ± 2.5 |
RIPC (n = 9) | 306.1 ± 6.7 | 1.52 ± 0.05 | 0.753 ± 0.036 | 0.415 ± 0.019 | 55.4 ± 2.0 |
HD + RIPC (n = 8) | 295.6 ± 8.5 | 1.50 ± 0.03 | 0.733 ± 0.032 | 0.425 ± 0.018 | 58.2 ± 1.7 |
HD (n = 8) | 300.6 ± 8.3 | 1.51 ± 0.06 | 0.726 ± 0.043 | 0.392 ± 0.020 | 54.7 ± 2.8 |
AN/AR in the control hearts was 37.0 ± 2.6% and AN/AR in the RIPC group was 25.7 ± 2.6%. RIPC significantly reduced infarct size over AR (P < 0.05). The infarct-reducing effect of RIPC was significantly inhibited by the selective mKATP channel blocker 5-HD (39.3 ± 3.0%, P < 0.05 vs. RIPC). The 5-HD itself did not significantly alter the infarct size (38.9 ± 3.0%) (Figure 2).
AN and AR Evaluated by 2,3,5-Triphenyltetrazolium Chloride Staining Following 30 Minutes of Occlusion and 2 Hours of Reperfusion in the Isolated Rat Heart
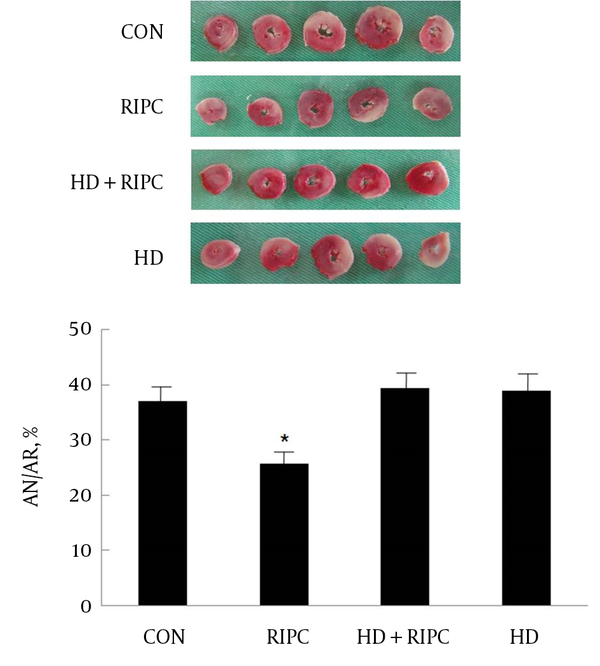
3.2. Cardiodynamic Data
There were no differences in the baseline HR and LVDP after stabilization or before regional ischemia. After 2 hours reperfusion, the HR and LVDP significantly decreased compared to baseline values in all groups (P < 0.05). However, there were no differences found among groups in HR and LVDP after 2 hours of reperfusion (Table 3).
Hemodynamic Changesa
Group | Heart rate, Beats/Min | LVDP, mmHg | ||
---|---|---|---|---|
Baseline | 2 h Reperfusion | Baseline | 2 h Reperfusion | |
CON (n = 8) | 322.7 ± 11.6 | 270.7 ± 11.6b | 123.6 ± 13.4 | 47.8 ± 3.8b |
RIPC (n = 9) | 331.9 ± 11.0 | 262.9 ± 18.1b | 126.0 ± 11.1 | 51.9 ± 7.7b |
HD + RIPC (n = 8) | 332.9 ± 10.4 | 256.6 ± 2.8b | 116.4 ± 3.2 | 50.9 ± 8.3b |
HD (n = 8) | 330.8 ± 11.8 | 278.1 ± 8.5b | 120.4 ± 3.8 | 44.9 ± 5.1b |
3.3. Arrhythmia Score
The arrhythmia score was presented as means ± SEM for the sum of each time point score in an individual heart. Two systems were used to compare the arrhythmia score. RIPC significantly reduced the arrhythmia score (8.7 ± 0.4), as compared to CON (14.6 ± 1.9, P = 0.023), by using the Curtis and Walker’ score system. The anti-arrhythmic effect of RIPC was inhibited by 5-HD (15.5 ± 1.6). Likewise, by using the Lepran’s system, RIPC significantly reduced the arrhythmia score (9.1 ± 0.5), as compared to CON (16.1 ± 2.1, P = 0.006). The anti-arrhythmic effect of RIPC was inhibited by 5-HD (16.0 ± 1.2). The 5-HD itself did not alter the arrhythmia score (16.9 ± 1.4) (Figure 3).
Arrhythmia Scores
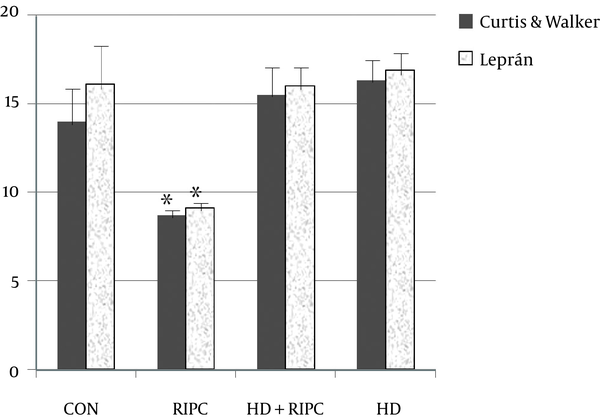
4. Discussion
Ischemic preconditioning (IPC) is a strong cardioprotective mechanism for ischemia and reperfusion injury. IPC is an early stress reaction that happens during repeated and brief vascular occlusion and reperfusion. It can make the ischemic injured tissue more tolerant to a following lethal ischemia (15).
Although the mechanisms underlying preconditioning are not yet completely understood, it is generally considered that opening mKATP channels plays an important role (7, 16).
The final common pathway is initiation of an intracellular kinases cascade following alteration of mitochondrial function within the cell via opening of mKATP channels (17) and closure of mitochondrial permeability transition pores (18). However, the accurate function of mKATP channel involvement remains to be explained (19).
Ischemic postconditioning also induces cardioprotection. The concept of ischemic postconditioning is interrupting reperfusion by making brief periods of ischemia, followed by continued reperfusion (20). However, this system is technically difficult and also causes direct stress to the target organ, which restricts its clinical application.
Przyklenk et al. (21) proposed the idea of remote preconditioning in 1993. They showed that brief interruption of the circumflex artery decreased myocardial infarction in the territory of the left anterior descending artery. Preconditioning at a distance, that is, remote ischemic preconditioning, adumbrates the degree of cardioprotection seen with local ischemic preconditioning (22); remote ischemic preconditioning seems to work through a similar intracellular signaling pathway (23-25). However, recent research proposes that there are important mechanistic differences between IPC and RIPC, and many researchers still claim to stand for different opinions regarding the similarity of degree of cardioprotection between IPC and RIPC (26).
The precise mechanism of the signaling pathway from remote tissue to target organ remains to be completely evaluated. Both a humoral and a neural pathway were suggested for signal transduction in RIPC. In the humoral pathway theory, humoral substance is released from the remote organ being used to induce conditioning (27, 28), while the theory of the neural pathway suggests that preconditioning might activate neural reflexes, which leads to the ischemic effect of cardioprotection (27).
There is some evidence that mKATP channels possibly play a role in organ protection in RIPC. Moses et al. have shown that RIPC reduced infarct size of the latissimus dorsi muscle, but that selective and nonselective mKATP channel blockers abolished this infarct reducing effect of RIPC (29). Kristiansen et al. (24) showed that nonselective mKATP channel blockers eliminated the cardioprotective effect of RIPC in a donor heart, and that selective mKATP channel openers, before explanting a heart, provided a protection similar to RIPC, suggesting that the effects of RIPC are crucially dependent on the role of the mKATP channels. KATP channels also mediate preconditioning of microvasculature and kidneys, while KATP channel blockers eliminate the preconditioning effects (30, 31).
As such, previous research evaluating the relationship between mKATP and RIPC was mostly performed on extracardiac organs or with a focus on infarct size and not on arrhythmias (11). Although RIPC reduces myocardial infarct size (23), there are limited studies on the effect of RIPC on severe reperfusion arrhythmias induced by I/R. The distinct feature of the present study therefore included a focus on cardiac and reperfusion arrhythmia as well as infarct size.
There are 2 studies on reperfusion arrhythmia, however, they are unrelated to mKATP channels as a possible mechanism. Oxman et al. (9) reported norepinephrine as a proposed mechanism. Dow et al. (10) showed that RIPC of lower limbs reduced I/R-induced ventricular arrhythmia, which is unrelated to the RISK pathway.
In this study, RIPC induced by occlusion and release of the bilateral femoral arteries significantly reduced reperfusion arrhythmia scores, as well as infarct size. However, pretreatment of a selective mKATP channel blocker, 5-HD, in the RIPC group abolished the anti-infarct and anti-arrhythmic effect of RIPC. In the absence of RIPC, 5-HD had no effect on both infarct size and arrhythmia. This result strongly suggests that mKATP channels play a role in RIPC-induced cardioprotection. The mKATP channel may be the common pathway mechanism of anti-infarct and anti-arrhythmic cardioprotection in classic ischemic preconditioning, ischemic postconditioning, as well as remote ischemic preconditioning.
While infarct size and arrhythmia were significantly reduced in the RIPC group, the hemodynamic parameters, such as HR and LVDP of the RIPC group were comparable to that of the control group. Not surprisingly, there are evidences that the HR, mean blood pressure, rate-pressure product, and LVDP even were decreased in comparison to the control hearts after reperfusion (32, 33). Even with the same experimental conditions, the changes of hemodynamic parameters after reperfusion are sometimes various and inconsistent.
Meanwhile, a selective mKATP channel blocker, 5-HD, was administrated 5 min before inducing RIPC in this study, which suggests that the mKATP channels play a role as a trigger of RIPC. Further evaluation is required to assess whether 5-HD plays a role as a mediator or as an end effector in RIPC; this could be investigated by administering the 5-HD either during or after RIPC.
RIPC can easily be used in clinical situations. The concept of RIPC has been used to reduce myocardial injury in patients undergoing various surgical interventions including coronary artery bypass graft surgery, abdominal aortic aneurysm repair, percutaneous coronary intervention, and heart valve surgery. The RIPC stimulus is delivered via alternate inflation and deflation of the blood pressure cuff tied on the upper arm of the patient (34).
Recently, new cardioprotective methods, which can easily be used in clinical settings, were presented. The supplementation of trace elements is known to induce cardioprotection by attenuating an inflammatory response in open-heart surgery with cardiopulmonary bypass (35).
The limitation of this study are the experimental models in which RIPC and I/R injury are performed, namely, in vivo and in an isolated perfused heart through the Langendorff system, respectively. Removal of the heart from the body may complicate things by removing the heart from any circulating factors.
In conclusion, RIPC significantly reduced both myocardial infarction and RAs in ischemia-reperfusion induced rat hearts. The mKATP channels play a role in reduction of both infarct size and RAs. RIPC can easily be implemented in clinical practice by using a blood pressure cuff; hence it would be cost effective and safe. The potential antiarrhythmic properties of RIPC in humans merits further investigation.
Acknowledgements
References
-
1.
Das B, Sarkar C. Similarities between ischemic preconditioning and 17beta-estradiol mediated cardiomyocyte KATP channel activation leading to cardioprotective and antiarrhythmic effects during ischemia/reperfusion in the intact rabbit heart. J Cardiovasc Pharmacol. 2006;47(2):277-86. [PubMed ID: 16495767]. https://doi.org/10.1097/01.fjc.0000202563.54043.d6.
-
2.
Gong JS, Yao YT, Fang NX, Li LH. Sevoflurane postconditioning attenuates reperfusion-induced ventricular arrhythmias in isolated rat hearts exposed to ischemia/reperfusion injury. Mol Biol Rep. 2012;39(6):6417-25. [PubMed ID: 22447537]. https://doi.org/10.1007/s11033-012-1447-9.
-
3.
Ardehali H. Role of the mitochondrial ATP-sensitive K+ channels in cardioprotection. Acta Biochim Pol. 2004;51(2):379-90. [PubMed ID: 15218535].
-
4.
Das B, Sarkar C. Cardiomyocyte mitochondrial KATP channels participate in the antiarrhythmic and antiinfarct effects of KATP activators during ischemia and reperfusion in an intact anesthetized rabbit model. Pol J Pharmacol. 2003;55(5):771-86. [PubMed ID: 14704474].
-
5.
Das B, Sarkar C. Is the sarcolemmal or mitochondrial K(ATP) channel activation important in the antiarrhythmic and cardioprotective effects during acute ischemia/reperfusion in the intact anesthetized rabbit model? Life Sci. 2005;77(11):1226-48. [PubMed ID: 15964023]. https://doi.org/10.1016/j.lfs.2004.12.042.
-
6.
Matejikova J, Ravingerova T, Pancza D, Carnicka S, Kolar F. Mitochondrial KATP opening confers protection against lethal myocardial injury and ischaemia-induced arrhythmias in the rat heart via PI3K/Akt-dependent and -independent mechanisms. Can J Physiol Pharmacol. 2009;87(12):1055-62. [PubMed ID: 20029542]. https://doi.org/10.1139/Y09-100.
-
7.
Negroni JA, Lascano EC, del Valle HF, Crottogini AJ. ATP-sensitive potassium channels do not have a main role in mediating late preconditioning protection against arrhythmias and stunning in conscious sheep. Basic Res Cardiol. 2002;97(1):55-64. [PubMed ID: 11998978].
-
8.
Dow J, Bhandari A, Kloner RA. The mechanism by which ischemic postconditioning reduces reperfusion arrhythmias in rats remains elusive. J Cardiovasc Pharmacol Ther. 2009;14(2):99-103. [PubMed ID: 19461101]. https://doi.org/10.1177/1074248408329606.
-
9.
Oxman T, Arad M, Klein R, Avazov N, Rabinowitz B. Limb ischemia preconditions the heart against reperfusion tachyarrhythmia. Am J Physiol. 1997;273(4 Pt 2):H1707-12. [PubMed ID: 9362234].
-
10.
Dow J, Bhandari A, Simkhovich BZ, Hale SL, Kloner RA. The effect of acute versus delayed remote ischemic preconditioning on reperfusion induced ventricular arrhythmias. J Cardiovasc Electrophysiol. 2012;23(12):1374-83. [PubMed ID: 23134527]. https://doi.org/10.1111/j.1540-8167.2012.02397.x.
-
11.
Tapuria N, Kumar Y, Habib MM, Abu Amara M, Seifalian AM, Davidson BR. Remote ischemic preconditioning: a novel protective method from ischemia reperfusion injury--a review. J Surg Res. 2008;150(2):304-30. [PubMed ID: 19040966]. https://doi.org/10.1016/j.jss.2007.12.747.
-
12.
Jang YH, Kim JH, Ban C, Ahn K, Cheong JH, Kim HH, et al. Stromal cell derived factor-1 (SDF-1) targeting reperfusion reduces myocardial infarction in isolated rat hearts. Cardiovasc Ther. 2012;30(5):264-72. [PubMed ID: 22958220]. https://doi.org/10.1111/j.1755-5922.2011.00301.x.
-
13.
Curtis MJ, Walker MJ. Quantification of arrhythmias using scoring systems: an examination of seven scores in an in vivo model of regional myocardial ischaemia. Cardiovasc Res. 1988;22(9):656-65. [PubMed ID: 3242835].
-
14.
Lepran I, Baczko I, Varro A, Papp JG. ATP-sensitive potassium channel modulators: both pinacidil and glibenclamide produce antiarrhythmic activity during acute myocardial infarction in conscious rats. J Pharmacol Exp Ther. 1996;277(3):1215-20. [PubMed ID: 8667181].
-
15.
Sanada S, Komuro I, Kitakaze M. Pathophysiology of myocardial reperfusion injury: preconditioning, postconditioning, and translational aspects of protective measures. Am J Physiol Heart Circ Physiol. 2011;301(5):H1723-41. [PubMed ID: 21856909]. https://doi.org/10.1152/ajpheart.00553.2011.
-
16.
Jaburek M, Costa AD, Burton JR, Costa CL, Garlid KD. Mitochondrial PKC epsilon and mitochondrial ATP-sensitive K+ channel copurify and coreconstitute to form a functioning signaling module in proteoliposomes. Circ Res. 2006;99(8):878-83. [PubMed ID: 16960097]. https://doi.org/10.1161/01.RES.0000245106.80628.d3.
-
17.
Das M, Das DK. Molecular mechanism of preconditioning. IUBMB Life. 2008;60(4):199-203. [PubMed ID: 18344203]. https://doi.org/10.1002/iub.31.
-
18.
Halestrap AP, Clarke SJ, Khaliulin I. The role of mitochondria in protection of the heart by preconditioning. Biochim Biophys Acta. 2007;1767(8):1007-31. [PubMed ID: 17631856]. https://doi.org/10.1016/j.bbabio.2007.05.008.
-
19.
Hanley PJ, Daut J. K(ATP) channels and preconditioning: a re-examination of the role of mitochondrial K(ATP) channels and an overview of alternative mechanisms. J Mol Cell Cardiol. 2005;39(1):17-50. [PubMed ID: 15907927]. https://doi.org/10.1016/j.yjmcc.2005.04.002.
-
20.
Penna C, Mancardi D, Raimondo S, Geuna S, Pagliaro P. The paradigm of postconditioning to protect the heart. J Cell Mol Med. 2008;12(2):435-58. [PubMed ID: 18182064]. https://doi.org/10.1111/j.1582-4934.2007.00210.x.
-
21.
Przyklenk K, Bauer B, Ovize M, Kloner RA, Whittaker P. Regional ischemic 'preconditioning' protects remote virgin myocardium from subsequent sustained coronary occlusion. Circulation. 1993;87(3):893-9. [PubMed ID: 7680290].
-
22.
Liem DA, te Lintel Hekkert M, Manintveld OC, Boomsma F, Verdouw PD, Duncker DJ. Myocardium tolerant to an adenosine-dependent ischemic preconditioning stimulus can still be protected by stimuli that employ alternative signaling pathways. Am J Physiol Heart Circ Physiol. 2005;288(3):H1165-72. [PubMed ID: 15486028]. https://doi.org/10.1152/ajpheart.00899.2004.
-
23.
Wolfrum S, Schneider K, Heidbreder M, Nienstedt J, Dominiak P, Dendorfer A. Remote preconditioning protects the heart by activating myocardial PKCepsilon-isoform. Cardiovasc Res. 2002;55(3):583-9. [PubMed ID: 12160956].
-
24.
Kristiansen SB, Henning O, Kharbanda RK, Nielsen-Kudsk JE, Schmidt MR, Redington AN, et al. Remote preconditioning reduces ischemic injury in the explanted heart by a KATP channel-dependent mechanism. Am J Physiol Heart Circ Physiol. 2005;288(3):H1252-6. [PubMed ID: 15498829]. https://doi.org/10.1152/ajpheart.00207.2004.
-
25.
Wang L, Oka N, Tropak M, Callahan J, Lee J, Wilson G, et al. Remote ischemic preconditioning elaborates a transferable blood-borne effector that protects mitochondrial structure and function and preserves myocardial performance after neonatal cardioplegic arrest. J Thorac Cardiovasc Surg. 2008;136(2):335-42. [PubMed ID: 18692639]. https://doi.org/10.1016/j.jtcvs.2007.12.055.
-
26.
Heidbreder M, Naumann A, Tempel K, Dominiak P, Dendorfer A. Remote vs. ischaemic preconditioning: the differential role of mitogen-activated protein kinase pathways. Cardiovasc Res. 2008;78(1):108-15. [PubMed ID: 18096574]. https://doi.org/10.1093/cvr/cvm114.
-
27.
Lim SY, Yellon DM, Hausenloy DJ. The neural and humoral pathways in remote limb ischemic preconditioning. Basic Res Cardiol. 2010;105(5):651-5. [PubMed ID: 20449597]. https://doi.org/10.1007/s00395-010-0099-y.
-
28.
Przyklenk K, Whittaker P. Remote ischemic preconditioning: current knowledge, unresolved questions, and future priorities. J Cardiovasc Pharmacol Ther. 2011;16(3-4):255-9. [PubMed ID: 21821525]. https://doi.org/10.1177/1074248411409040.
-
29.
Moses MA, Addison PD, Neligan PC, Ashrafpour H, Huang N, Zair M, et al. Mitochondrial KATP channels in hindlimb remote ischemic preconditioning of skeletal muscle against infarction. Am J Physiol Heart Circ Physiol. 2005;288(2):H559-67. [PubMed ID: 15458954]. https://doi.org/10.1152/ajpheart.00845.2004.
-
30.
Mabanta L, Valane P, Borne J, Frame MD. Initiation of remote microvascular preconditioning requires K(ATP) channel activity. Am J Physiol Heart Circ Physiol. 2006;290(1):H264-71. [PubMed ID: 16126818]. https://doi.org/10.1152/ajpheart.00455.2005.
-
31.
Pell TJ, Baxter GF, Yellon DM, Drew GM. Renal ischemia preconditions myocardium: role of adenosine receptors and ATP-sensitive potassium channels. Am J Physiol. 1998;275(5 Pt 2):H1542-7. [PubMed ID: 9815059].
-
32.
Chun KJ, Park YH, Kim JS, Jang Y, Kim JH, Kim J, et al. Comparison of 5 different remifentanil strategies against myocardial ischemia-reperfusion injury. J Cardiothorac Vasc Anesth. 2011;25(6):926-30. [PubMed ID: 21514843]. https://doi.org/10.1053/j.jvca.2011.02.019.
-
33.
Zhang Y, Irwin MG, Wong TM. Remifentanil preconditioning protects against ischemic injury in the intact rat heart. Anesthesiology. 2004;101(4):918-23. [PubMed ID: 15448525].
-
34.
Randhawa PK, Bali A, Jaggi AS. RIPC for multiorgan salvage in clinical settings: evolution of concept, evidences and mechanisms. Eur J Pharmacol. 2015;746:317-32. [PubMed ID: 25176179]. https://doi.org/10.1016/j.ejphar.2014.08.016.
-
35.
Sedighinejad A, Imantalab V, Mirmansouri A, Mohammadzadeh Jouryabi A, Kanani G, Nassiri Sheikhani N, et al. Effects of Low-dose Selenium on the Inflammatory Response in Coronary Artery Bypass Graft Surgery: A Clinical Trial. Iran Red Crescent Med J. 2016;18(8). ee37918. [PubMed ID: 27795837]. https://doi.org/10.5812/ircmj.37918.