Abstract
Background:
It has long been suspected that exposure to stress is a major factor that can increase the risk of Multiple Sclerosis (MS) and exacerbate it, as a stress-related disorder. Therefore, we conducted this study to investigate the response of the hypothalamic-pituitary-adrenal (HPA) axis and sympathetic-adrenal-medullar (SAM) system to acute social stress.Methods:
A total of 46 MS patients and 23 age-sex-matched healthy controls were recruited in the study. The Trier Social Stress Test (TSST) was used to induce acute psychosocial stress. We measured salivary cortisol (SC) to evaluate the HPA axis. In addition, electrocardiography (ECG) was recorded to evaluate the SAM system based on the linear and non-linear features of Heart Rate Variation (HRV). Then, SC and HRV were measured before and after the stress exposure. We also used the Depression Anxiety Stress Scale (DASS) and Emotional Visual Analog Scale (EVAS) to conduct the psychometric assessment and evaluate the perceived stress level, respectively.Results:
The mean age of the MS group was 35.38 ± 15 years, with a mean disease duration of 7.4 ± 60. Besides, the HC group's mean age was 35.8 ± 9 years. There were no significant differences in demographic features and DASS scores between the two groups. In response to TSST, both MS and healthy individuals showed a significant increase in the SC levels and EVAS scores, as well as changes in the HRV indices. Notably, significant differences were also found between the two groups regarding the basic and post-stress SC levels, EVAS score, and HRV indices. Unlike the HC group, the SC level returned to its baseline after recovery in the MS group, and the sympathetic tone was more sensitive.Conclusions:
Our results indicated that both MS and healthy individuals respond to acute stress regarding neuroendocrine assessment; however, patients with multiple sclerosis show some impairments in this response.Keywords
1. Background
Multiple sclerosis (MS) is the most common inflammatory autoimmune disease in the central nervous system (CNS) of young adults (1). Patients with MS usually experience several attacks, leading to severe disability, which is highly associated with morbidity and decreased quality of life (2). There is a growing body of literature indicating the association between stress, brain inflammation, immune system, and worsening of psychological disorders (3-5). In response to stress, neuropeptides such as corticotrophin-releasing hormone (CRH) and neurotensin (NT) activate microglia and mast cells to release inflammatory molecules. Then, it results in the activation of T17 autoimmune cells, breakdown of the blood-brain barrier, and the invasion of T-cells into the brain, all of which contribute to MS pathology (6). Previous studies have also explored the relationships between stressful life events and exacerbations of MS (7). Moreover, it has been suggested that stressful life events may increase the risk of the occurrence of a new gadolinium-enhanced magnetic resonance imaging (MRI) brain lesion (8).
Whilst some research has been carried out on the relationship between MS and stress, the mechanism by which stress affects MS has not been established well. In fact, psychological, social, and biological factors may affect the relationship between stressful life events and MS. It is well-known that the hypothalamic-pituitary-adrenal (HPA) axis and sympathetic-adrenal-medullar (SAM) system are involved in the response to stress (9). One of the most significant current discussions in stress research is the interaction between the HPA axis and MS. It has commonly been assumed that a decrease in cortisol secretion under chronic stress suppresses the immune response to inflammation (10). Therefore, chronic stress may be associated with the increased risk of inflammation and exacerbation in MS patients. In this context, it has been suggested that reduced production of adrenal steroids can cause impairments in the HPA axis, followed by a neurological worsening in MS patients (11). Another explanation for this interaction is the glucocorticoid resistance hypothesis (12).
It should be noted that a limited number of studies have assessed the acute response of the stress system in MS patients. Among them, only one study showed a vigorous SAM activation in response to acute stress (13). None of them found the difference between MS and healthy individuals in terms of the stress-related cortisol/catecholamine levels (13-15). According to the results of these studies, the degree of HPA system activity depends on disease duration so that its activity increases in the first year after MS diagnosis. On the other hand, circulating cortisol levels decrease during the second year or later (15).
To conclude, the data regarding the relationship between acute stress, the HPA axis, and the SAM system are either scarce or inadequate. Therefore, we conducted this study to assess the responses of the HPA axis (index: cortisol level) and SAM system (index: HRV indices), as two stress response systems, to acute stress and determine the baseline stress levels (using Depression Anxiety Stress Scale, DASS) in MS and healthy individuals. Thus, the study hypothesized that MS patients have defective responses to acute stress.
2. Methods
2.1. Study Participants
In this study, we surveyed convenience MS patients who were referred to the MS clinic of Kashani Hospital, affiliated with Isfahan University of Medical Sciences, Isfahan, Iran, between July 2019 and September 2020. In parallel, an age- and gender-matched sample of healthy individuals was recruited. The inclusion criteria for MS patients in this study were (1) a definite diagnosis of MS based on the McDonald criteria in the remission phase with no attacks in the last year (16, 17), (2) age of over 18 years, (3) no other autoimmune disorders or endocrine dysfunction and current exacerbations, (4) no history of smoking, and (5) no use of corticosteroid drugs in the last year. All cases were men to exclude the effect of different sex hormones on the stress system. This study was approved by the Bioethics Committee of Baqiyatallah University of Medical Sciences with a code of IR.BMSU.REC.1398.277 and code of IRCT IRCT20171128037666N2. The participants were adequately informed of the study. Written informed consent was taken from all individuals.
2.2. Demographic and Clinical Characteristics
Demographic information was obtained, including age, gender, employment status (employed/unemployed), and education level (basic/advance). A diploma degree or lower was considered a basic level of education. Clinical features including disease severity, disease duration, and disease course were also recorded. The disease severity was measured using the Expanded Disability Status Scale (EDSS) (18) by a single neurologist.
2.3. Procedure
The participants were asked to have good sleep without stress one day before the test, not to eat anything one hour before the test, and to wash their mouth before the test. We used the Trier Social Stress Test (TSST) to induce the acute psychosocial stress condition in the participants (19). This test consisted of three components, including five minutes to prepare a presentation (anticipatory stress component), two minutes to speech (self-introduction presentation component), and eight minutes of mental arithmetic component (in which the participants must count aloud backward from 2023 to 0 in 17-step sequences) in front of two judges. To control the circadian rhythm, this test was performed between 13:00 and 16:00 in the same room at 23 – 25°C. The procedure was described previously in detail (20).
Before stress exposure, immediately after stress exposure, and 20 minutes after recovery, 5 ml of saliva was taken for salivary cortisol measurement to assess HPA responses. Electrocardiography (ECG) was recorded three times (before and after TSST and 20 minutes after recovery) and during TSST to evaluate the SAM response. For psychometric assessments, we then used the Depression Anxiety Stress Scale (DASS) after the test. In addition, the Emotional Visual Analog Scale (EVAS) was used for rating the intensity of emotions. This measurement instrument included a horizontally scaled line between 0 and 10 scores, while higher scores indicated higher and unbearable distress. The diagram of the study is shown in Figure 1.
Study procedure
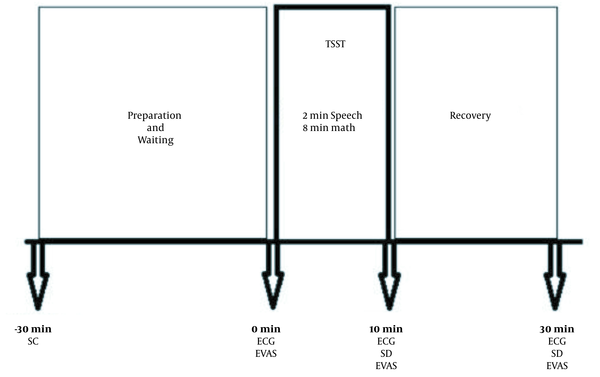
2.4. Salivary Cortisol (SC) Level Assessment
A minimum of 5 ml of saliva sample was collected three times from the patients and healthy controls (HCs) for SC level measurement. All samples were immediately cooled and stored at -80°C until analysis. Salivary cortisol concentrations were determined using a commercial enzyme immunoassay assay (IBL, Hamburg, Germany) according to the manufacturer's instructions. The concentration of free saliva cortisol was reported in nanomoles per liter. The reportable range of SC with this kit was 0.015 – 3 μg/dL.
2.5. Heart Rate Variability Assessment
Biomedical wireless ECG (made in Iran) was used to record the heart rate digitally (21). According to the vectorcardiogram system, the electrodes were fixed by a chest lid, and the instrument was then fixed by a belt to the chest. The participants were asked to stay in a sitting position without deep breathing or speaking during the test. In each phase, data were saved and then transferred to an analog-to-digital converter (ADC) with a 256 HZ sampling rate. We used MATLAB software to calculate heart rate variability (HRV). The linear features extracted from the RR series in the time domain were the mean and SD of RR. In the frequency domain, the HF power (0.15 – 0.5 Hz), LF power (0.05 – 0.15 Hz), very LF power (0 – 0.04 Hz), and finally, the ratio of LF/HF components were analyzed (22). The non-linear features extracted in the time domain were SD1 and SD2 of Poincaré Plot (23) and the alpha 1 of detruded fluctuation analysis (DFA) (24). In the frequency domain, spectral entropy (SpeEn) was extracted as a non-linear feature (25).
2.6. DASS Questionnaire
A self-administered questionnaire was used with 42 questions to assess depression, anxiety, and stress over the last week. Each of the three scales contained 12 items. The patients were asked to rate their chances of dozing on a four-point scale (0 - 3), where higher scores indicated higher severity/frequency of items. The score of each scale could range from 0 to 36 points, and a higher score indicated the more severity of the scale. The validity and reliability of this scale were tested in an Iranian investigation (26, 27).
2.7. Statistical Analysis
Data were presented as the mean ± standard deviation (SD) and frequency (%) for interval and categorical variables, respectively. We used the independent sample t-test to compare the means of normally distributed continuous variables, as well as those continuous variables that were not normally distributed. The Mann–Whitney U test was also used. A chi-square test or two-tailed Fisher’s exact test was used to analyze the differences between categorical variables. Pearson correlation was performed to find associations between continuous variables. We used two-way mixed-model ANOVA to compare repeated variables, including cortisol levels and HRV between the two groups. All statistical calculations were done using SPSS 24.0 for windows (SPSS, Chicago, IL, USA). The P < 0.05 was considered significant.
3. Results
3.1. Study Population Characteristics
A total of 43 MS patients and 23 healthy individuals were recruited in the study. The mean age of all MS patients and HCs was 35.38 ± 15 and 35.8 ± 9, respectively. The mean disease duration and EDSS were 7.4 ± 60 and 1.68 ± 1.7, respectively. No significant difference was observed between the two groups in terms of demographic features and DASS scores (P-value > 0.05). The basic clinical and para-clinical findings are summarized in Table 1.
Demographic and Clinical Characteristics of Participants a
Baseline Characteristics | HC Group (N = 23) | MS Group (N = 43) | P-Value |
---|---|---|---|
Age | 35.38 ± 15 | 35.8 ± 9 | 0.9 |
Weight | 180.56 ± 10 | 176.58 ± 9 | 0.16 |
Height | 80.5 ± 15 | 76.16 ± 16 | 0.35 |
Marital status (married) | 50 | 58.1 | 0.39 |
Education (basic/advance) | 37.5/62.6 | 51.2/48.9 | 0.63 |
EDSS | - | 1.68 ± | |
Duration of disease, y | - | 7.4 ± 6 | |
DASS-stress | 14.8 ± 12 | 16.3 ± 9 | 0.6 |
DASS-anxiety | 11.2 ± 9 | 9.5 ± 5 | 0.47 |
DASS-depression | 11.4 ± 12 | 9 ± 8 | 0.5 |
Pre-stress salivary cortisol | 5.11 ± 1.3 | 7.46 ± 4.1 | 0.001 |
3.2. TSST-related Changes in SC and EVAS Score
As shown in Figure 2, both MS and HC groups showed increased levels of SC in response to TSST. Further analysis showed that MS patients had higher levels of SC than the HC group in HPA response to acute stress (Table 2) (P < 0.01).
Results of Two-way Mixed Model ANOVA of Interaction of Repeated Measurements and Study Groups
Variable | P-Value | ||
---|---|---|---|
Time | Group | Time × Group | |
EVAS (score) | 0.00000 | 0.00002 | 0.05 |
Salivary cortisol (μg/dL) | 0.00000 | 0.01 | 0.09 |
R-R Interval (ms) | 0.00004 | 0.47 | 0.4 |
SD of R-R interval | 0.00008 | 0.74 | 0.49 |
Heart rate (beats/second) | 0.001 | 0.36 | 0.45 |
Relative very low, No. (%) | 0.26 | 0.19 | 0.4 |
Relative low, No. (%) | 0.051 | 0.29 | 0.3 |
Relative high, No. (%) | 0.01 | 0.89 | 0.46 |
LF/HF ratio | 0.066 | 0.74 | 0.036 |
SD1 of poincare plot | 0.001 | 0.6 | 0.81 |
SD2 of poincare plot | 0.0001 | 0.7 | 0.43 |
Sample entropy | 0.0004 | 0.57 | 0.25 |
Alpha 1of DFA | 0.016 | 0.25 | 0.49 |
Spectral entropy | 0.42 | 0.17 | 0.035 |
The level of salivary cortisol (SC) in response to TSST. The levels of SC increased after TSST in both groups (**: P < 0.01 between post-stress and pre-stress state in HC group, ***: P < 0.0000 between post-stress and pre-stress and recovery state in MS group). The SC level was higher in the MS group than in the HC group (*: P < 0.01).
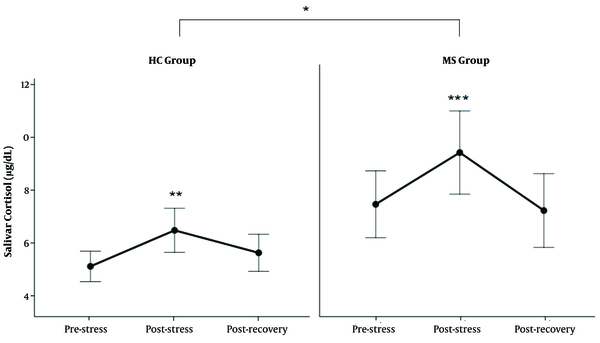
In Figure 3, there is a marked increase in the EVAS score in response to TSST in both groups. Further statistical tests revealed higher EVAS scores in MS patients than in healthy subjects (Table 2).
The score of EVAS in response to TSST; The EVAS score increased after TSST in both groups (** P < 0.0000 between post-stress and two other states and recovery with the pre-stress state in HC group, and between post-stress and pre-stress and recovery state in MS group). The EVAS level was higher in the MS group than in the HC group (**: P < 0.00001).
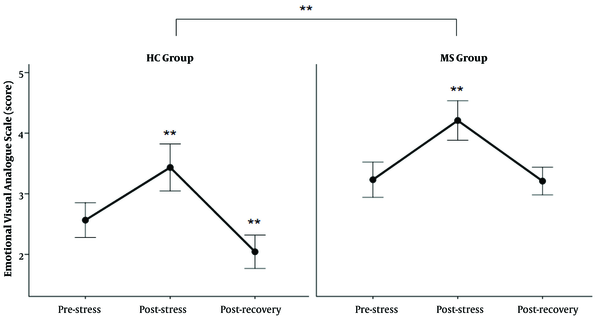
3.3. TSST-related Changes in Heart Rate Variability
Figure 4 shows the responses of the SAM system to TSST. A significant decrease was observed in the mean of R-R interval, the SD of the mean of R-R interval, SD1 of Poincare plot, and spectral entropy of HRV in response to TSST, in both MS patients and HCs. The LF/HF ratio significantly increased only in MS patients. In addition, the alpha 1 of DFA of HRV increased in both study
The response of the SAM system to TSST; (A) A difference was found between recovery state and all five time-sets of 10 minutes. (B) A difference was found between the first time-set of TSST and the second to fifth time-sets. (C) A difference was found between the third time-set and recovery state. (D) A difference was found between recovery state and the second to fifth time-sets of TSST and pre-stress state. (E) There is a difference between recovery state and the first and fourth time-sets of TSST. (F) There is a difference between pre-stress state and the third and fifth time-sets of TSST in the HC group and the fourth time-set of TSST in the MS group. Also, a difference was found between recovery state and the fifth time-set in the HC group and the fourth time-set of TSST in the MS group; *: P < 0.05; **: P < 0.01; ***: P < 0.0005
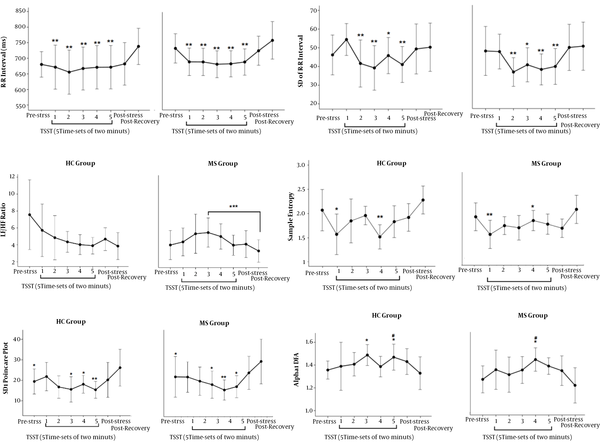
4. Discussion
This study aimed to evaluate the responses of the HPA axis and SAM system to acute stress in MS patients. According to the key findings of the present study, MS patients had higher SC levels at the baseline than healthy controls. Both MS and HC groups revealed an increase in the SC levels and EVAS scores. In addition, an alteration of the SAM system occurred in MS patients to increase the sympathetic tone immediately in response to the TSST. Two significantly different responses were observed in the two groups as follows: (1) A decline and return of cortisol to baseline after recovery in the MS group, but not in the HC group, and (2) a more sensitive LF/HF ratio of HRV to stress in the MS group.
It is well-known that there is an HPA axis abnormality in patients with MS (28). Glucocorticoid, as an end-product of the HPA system, plays a broad role in the regulation of the immune system (29). Although some studies revealed the hypo-reactivity of the HPA axis in some MS patients (28, 30), more recent studies have indicated HPA axis hyperactivity in MS patients. These studies demonstrated that the HPA axis hyperactivity acts as a predisposing factor in the development of MS and the accumulation of disease disability (28). It was shown that the basic levels of cortisol and adrenocorticotropic hormone (ACTH) were higher in MS patients than in people without any health problems (31). In an experimental study, increases in the levels of Interleukin (IL)-1β and tumor necrosis factor (TNF)-α were observed in the hypothalamus of experimental autoimmune encephalomyelitis (EAE) mouse. Besides, they found an increase in the levels of plasma cortisol in comparison with the normal mouse (32). In agreement with these studies, we found that those MS patients with higher levels of pretest salivary cortisol showed more increases in the cortisol level and sympathetic tone after the acute social stress compared to healthy individuals (33).
It should be noted that the response of the HPA axis to acute social stress is not still clear in MS patients. It has been suggested that MS and general populations have different responses to chronic and severe stressors (34). Also, the effects of acute and chronic stress on the immune system are different. The hormonal response to acute stress is mainly mediated by the HPA axis through the release of both neurotransmitter and endocrine mediators, which are peripheral but central actions (35).
We found robust responses to acute stress in both MS and healthy groups, as well as higher levels of cortisol in MS patients than in the HC group. The increased levels of cortisol in response to acute stress in MS patients may be related to peripheral inflammation (36). There is fine coordination between the HPA axis and the immune system. Chronic and autoimmune disorders such as MS can cause disturbance in this fine-tuned circuit. Peripheral inflammation cytokines can induce HPA axis activation, and in turn, cortisol can suppress inflammation (35). It has been proposed that elevated cortisol levels in MS patients are a compensatory mechanism against inflammation by the inhabitation of NF-κΒ, AP1, several signal transducers and activators of transcription (STATs), and proinflammatory cytokines such as TNF-α, IL-1, IL-2, IL-6, IL-12, and IFN-γ (36, 37). Besides, the HPA axis can affect the immune system by increasing the production of anti-inflammatory cytokines such as IL-4 and IL-10 (38).
In a similar study using TSST to induce acute stress, a time-dependent condition was observed in glucocorticoid response. The researchers found that MS patients with a disease duration of less than one year showed elevated cortisol in response to stress, compared to the healthy individuals. This difference was not found in MS patients with a disease duration of more than one year, compared to HCs (15). These results differ from the perspective of Heesen et al. (13), who did not find significant changes in both patients and healthy participants in terms of neuroendocrine parameters including catecholamines, prolactin, IL-6, and TNF-a during exposure to acute stress. Moreover, they found declines in the levels of neuroendocrine parameters in response to stress (13). Ackerman et al. found an increase in the levels of neutrophils, monocytes, CD8+ suppressor/cytotoxic T-lymphocytes, and NK-cells in MS as well as healthy subjects, in response to acute stress (14).
Regarding HRV analysis, we found an important change in the SAM system responding to acute stress in both MS and healthy controls. In addition, during the stress challenge, there was a significant difference between MS and HC groups in terms of the ratio of LF/HF, as an index of sympathetic tone. Autonomic impairment is a common feature of MS, affecting about 7% to 60% of patients (39). It has commonly been assumed that autonomic impairment may be due to demyelinating lesions in the areas that mediate autonomic functions, such as the brainstem and spinal cord (40). In turn, autonomic sensitivity can lead to the enhancement of inflammation and progression of disease (41).
It should be noted that the present study has some limitations. The major limitation is its small sample size. Another limitation is the inclusion of only male patients. Since the responses of the HPA and SAM system to stress might be different in females (33), our findings might not be extended to female MS cases. Generally, females have a more robust HPA and SAM response to acute stress than males (42, 43). Here, we assessed neuroendocrine response in male patients to exclude the effect of gonadal hormones. Notwithstanding these limitations, the study suggests that there is an altered neuroendocrine response in MS patients during acute stress.
References
-
1.
Sospedra M, Martin R. Immunology of multiple sclerosis. Annu Rev Immunol. 2005;23:683-747. [PubMed ID: 15771584]. https://doi.org/10.1146/annurev.immunol.23.021704.115707.
-
2.
MacAllister WS, Christodoulou C, Troxell R, Milazzo M, Block P, Preston TE, et al. Fatigue and quality of life in pediatric multiple sclerosis. Mult Scler. 2009;15(12):1502-8. [PubMed ID: 19965517]. https://doi.org/10.1177/1352458509345902.
-
3.
Nakata A. Psychosocial job stress and immunity: A systematic review. Methods Mol Biol. 2012;934:39-75. [PubMed ID: 22933140]. https://doi.org/10.1007/978-1-62703-071-7_3.
-
4.
Gárate I, Garcia-Bueno B, Madrigal JLM, Caso JR, Alou L, Gomez-Lus ML, et al. Stress-induced neuroinflammation: Role of the toll-like receptor-4 pathway. Biological Psychiatry. 2013;73(1):32-43. https://doi.org/10.1016/j.biopsych.2012.07.005.
-
5.
Theoharides TC, Zhang B, Conti P. Decreased mitochondrial function and increased brain inflammation in bipolar disorder and other neuropsychiatric diseases. J Clin Psychopharmacol. 2011;31(6):685-7. [PubMed ID: 22020358]. https://doi.org/10.1097/JCP.0b013e318239c190.
-
6.
Karagkouni A, Alevizos M, Theoharides TC. Effect of stress on brain inflammation and multiple sclerosis. Autoimmun Rev. 2013;12(10):947-53. [PubMed ID: 23537508]. https://doi.org/10.1016/j.autrev.2013.02.006.
-
7.
Mohr DC, Hart SL, Julian L, Cox D, Pelletier D. Association between stressful life events and exacerbation in multiple sclerosis: a meta-analysis. BMJ. 2004;328(7442):731. [PubMed ID: 15033880]. [PubMed Central ID: PMC381319]. https://doi.org/10.1136/bmj.38041.724421.55.
-
8.
Mohr DC. Stress and multiple sclerosis. J Neurol. 2007;254 Suppl 2:II65-8. [PubMed ID: 17503132]. https://doi.org/10.1007/s00415-007-2015-4.
-
9.
Smith SM, Vale WW. The role of the hypothalamic-pituitary-adrenal axis in neuroendocrine responses to stress. Dialogues Clin Neurosci. 2007;8(4):383-95. https://doi.org/10.31887/DCNS.2006.8.4/ssmith.
-
10.
Dickerson SS, Kemeny ME. Acute stressors and cortisol responses: A theoretical integration and synthesis of laboratory research. Psychol Bull. 2004;130(3):355. [PubMed ID: 15122924]. https://doi.org/10.1037/0033-2909.130.3.355.
-
11.
Silverman MN, Sternberg EM. Glucocorticoid regulation of inflammation and its functional correlates: from HPA axis to glucocorticoid receptor dysfunction. Ann N Y Acad Sci. 2012;1261:55-63. [PubMed ID: 22823394]. [PubMed Central ID: PMC3572859]. https://doi.org/10.1111/j.1749-6632.2012.06633.x.
-
12.
Miller GE, Cohen S, Ritchey AK. Chronic psychological stress and the regulation of pro-inflammatory cytokines: a glucocorticoid-resistance model. Health Psychol. 2002;21(6):531-41. [PubMed ID: 12433005]. https://doi.org/10.1037//0278-6133.21.6.531.
-
13.
Heesen C, Schulz H, Schmidt M, Gold S, Tessmer W, Schulz KH. Endocrine and cytokine responses to acute psychological stress in multiple sclerosis. Brain Behav Immun. 2002;16(3):282-7. [PubMed ID: 12009688]. https://doi.org/10.1006/brbi.2001.0628.
-
14.
Ackerman KD, Martino M, Heyman R, Moyna NM, Rabin BS. Immunologic response to acute psychological stress in MS patients and controls. Journal of Neuroimmunology. 1996;68(1-2):85-94. https://doi.org/10.1016/0165-5728(96)00077-x.
-
15.
Kern S, Rohleder N, Eisenhofer G, Lange J, Ziemssen T. Time matters - acute stress response and glucocorticoid sensitivity in early multiple sclerosis. Brain Behav Immun. 2014;41:82-9. [PubMed ID: 24880115]. https://doi.org/10.1016/j.bbi.2014.04.006.
-
16.
Thompson AJ, Banwell BL, Barkhof F, Carroll WM, Coetzee T, Comi G, et al. Diagnosis of multiple sclerosis: 2017 revisions of the McDonald criteria. Lancet Neurol. 2018;17(2):162-73. https://doi.org/10.1016/s1474-4422(17)30470-2.
-
17.
Polman CH, Reingold SC, Banwell B, Clanet M, Cohen JA, Filippi M, et al. Diagnostic criteria for multiple sclerosis: 2010 revisions to the McDonald criteria. Ann Neurol. 2011;69(2):292-302. [PubMed ID: 21387374]. [PubMed Central ID: PMC3084507]. https://doi.org/10.1002/ana.22366.
-
18.
Kurtzke JF. Rating neurologic impairment in multiple sclerosis: an expanded disability status scale (EDSS). Neurology. 1983;33(11):1444-52. [PubMed ID: 6685237]. https://doi.org/10.1212/wnl.33.11.1444.
-
19.
Kirschbaum C, Pirke KM, Hellhammer DH. The 'Trier Social Stress Test'--a tool for investigating psychobiological stress responses in a laboratory setting. Neuropsychobiology. 1993;28(1-2):76-81. [PubMed ID: 8255414]. https://doi.org/10.1159/000119004.
-
20.
Mohammadi A, Emamgoli A, Shirinkalam M, Meftahi GH, Yagoobi K, Hatef B. The persistent effect of acute psychosocial stress on heart rate variability. Egypt Heart J. 2019;71(1):1-9. [PubMed ID: 31659513]. [PubMed Central ID: PMC6821413]. https://doi.org/10.1186/s43044-019-0009-z.
-
21.
Chia JM, Fischer SE, Wickline SA, Lorenz CH. Performance of QRS detection for cardiac magnetic resonance imaging with a novel vectorcardiographic triggering method. Journal of Magnetic Resonance Imaging. 2000;12(5):678-88. https://doi.org/10.1002/1522-2586(200011)12:5<678::Aid-jmri4>3.0.Co;2-5.
-
22.
Billman GE. The LF/HF ratio does not accurately measure cardiac sympatho-vagal balance. Front Physiol. 2013;4:26. [PubMed ID: 23431279]. [PubMed Central ID: PMC3576706]. https://doi.org/10.3389/fphys.2013.00026.
-
23.
Brennan M, Palaniswami M, Kamen P. Do existing measures of Poincare plot geometry reflect nonlinear features of heart rate variability? IEEE Trans Biomed Eng. 2001;48(11):1342-7. [PubMed ID: 11686633]. https://doi.org/10.1109/10.959330.
-
24.
Perfetto J, Ruiz A, D'Attellis C. Detrended fluctuation analysis (DFA) and R-R interval variability: A new linear segmentation algorithm. Computers In Cardiology. 2006. p. 629-32.
-
25.
Subha DP, Joseph PK, Acharya UR, Lim CM. EEG signal analysis: A survey. J Med Syst. 2010;34(2):195-212. [PubMed ID: 20433058]. https://doi.org/10.1007/s10916-008-9231-z.
-
26.
Afzali A, Delavar A, Borjali A, Mirzamani M. [Psychometric properties of DASS-42 as assessed in a sample of Kermanshah High School students]. J Res Behav Sci. 2007;5(2 (10)). Persian.
-
27.
Habibi M, Dehghani M, Pooravari M, Salehi S. Confirmatory factor analysis of Persian version of depression, anxiety and stress (DASS-42): Non-clinical sample. Razavi Int J Med. 2017;5(4). https://doi.org/10.5812/rijm.12021.
-
28.
Whirledge S, Cidlowski JA. A role for glucocorticoids in stress-impaired reproduction: beyond the hypothalamus and pituitary. Endocrinology. 2013;154(12):4450-68. [PubMed ID: 24064362]. [PubMed Central ID: PMC3836069]. https://doi.org/10.1210/en.2013-1652.
-
29.
Yaribeygi H, Panahi Y, Sahraei H, Johnston TP, Sahebkar A. The impact of stress on body function: A review. EXCLI J. 2017;16:1057-72. [PubMed ID: 28900385]. [PubMed Central ID: PMC5579396]. https://doi.org/10.17179/excli2017-480.
-
30.
Bechmann L, Busse K, Stoppe M, Cotte S, Ettrich B, Then Bergh F. Corticosteroid receptor expression and in vivo glucocorticoid sensitivity in multiple sclerosis. J Neuroimmunol. 2014;276(1-2):159-65. [PubMed ID: 25175068]. https://doi.org/10.1016/j.jneuroim.2014.07.004.
-
31.
Michelson D, Stone L, Galliven E, Magiakou MA, Chrousos GP, Sternberg EM, et al. Multiple sclerosis is associated with alterations in hypothalamic-pituitary-adrenal axis function. J Clin Endocrinol Metab. 1994;79(3):848-53. https://doi.org/10.1210/jcem.79.3.8077372.
-
32.
Acharjee S, Nayani N, Tsutsui M, Hill MN, Ousman SS, Pittman QJ. Altered cognitive-emotional behavior in early experimental autoimmune encephalitis--cytokine and hormonal correlates. Brain Behav Immun. 2013;33:164-72. [PubMed ID: 23886782]. https://doi.org/10.1016/j.bbi.2013.07.003.
-
33.
Mohammadi A, Emamgoli A, Shirinkalam M, Meftahi GH, Yagoobi K, Hatef B. The persistent effect of acute psychosocial stress on heart rate variability. Egypt Heart J. 2019;71(1):18. [PubMed ID: 31659513]. [PubMed Central ID: PMC6821413]. https://doi.org/10.1186/s43044-019-0009-z.
-
34.
Brown RF, Tennant CC, Sharrock M, Hodgkinson S, Dunn SM, Pollard JD. Relationship between stress and relapse in multiple sclerosis: Part I. Important features. Mult Scler. 2006;12(4):453-64. [PubMed ID: 16900759]. https://doi.org/10.1191/1352458506ms1295oa.
-
35.
Anagnostouli M, Markoglou N, Chrousos G. Psycho-neuro-endocrino-immunologic issues in multiple sclerosis: a critical review of clinical and therapeutic implications. Hormones (Athens). 2020;19(4):485-96. [PubMed ID: 32488815]. https://doi.org/10.1007/s42000-020-00197-8.
-
36.
Ruiz F, Vigne S, Pot C. Resolution of inflammation during multiple sclerosis. Semin Immunopathol. 2019;41(6):711-26. [PubMed ID: 31732775]. [PubMed Central ID: PMC6881249]. https://doi.org/10.1007/s00281-019-00765-0.
-
37.
Sudheimer KD, O'Hara R, Spiegel D, Powers B, Kraemer HC, Neri E, et al. Cortisol, cytokines, and hippocampal volume interactions in the elderly. Front Aging Neurosci. 2014;6:153. [PubMed ID: 25071562]. [PubMed Central ID: PMC4079951]. https://doi.org/10.3389/fnagi.2014.00153.
-
38.
Dickerson SS, Kemeny ME. Acute stressors and cortisol responses: a theoretical integration and synthesis of laboratory research. Psychol Bull. 2004;130(3):91-355. [PubMed ID: 15122924]. https://doi.org/10.1037/0033-2909.130.3.355.
-
39.
Huang M, Jay O, Davis SL. Autonomic dysfunction in multiple sclerosis: implications for exercise. Auton Neurosci. 2015;188:82-5. [PubMed ID: 25458432]. [PubMed Central ID: PMC4852554]. https://doi.org/10.1016/j.autneu.2014.10.017.
-
40.
McDougall AJ, McLeod JG. Autonomic nervous system function in multiple sclerosis. J Neurol Sci. 2003;215(1-2):79-85. https://doi.org/10.1016/s0022-510x(03)00205-3.
-
41.
Racosta JM, Kimpinski K. Autonomic dysfunction, immune regulation, and multiple sclerosis. Clin Auton Res. 2016;26(1):23-31. [PubMed ID: 26691635]. https://doi.org/10.1007/s10286-015-0325-7.
-
42.
Kim HG, Cheon EJ, Bai DS, Lee YH, Koo BH. Stress and heart rate variability: A meta-analysis and review of the literature. Psychiatry Investig. 2018;15(3):235-45. [PubMed ID: 29486547]. [PubMed Central ID: PMC5900369]. https://doi.org/10.30773/pi.2017.08.17.
-
43.
Ehteram BZ, Sahraei H, Meftahi GH, Khosravi M. Effect of intermittent feeding on gonadal function in male and female NMRI mice during chronic stress. Braz Arch Biol Technol. 2017;60(0). https://doi.org/10.1590/1678-4324-2017160607.
reply