Abstract
Context:
Cerebral palsy (CP) results from damage to the central nervous system, leading to disturbances of motor and sensory functions, especially the balance. Virtual reality exercise intervention (VRI) is a promising technique to improve motor function in children with CP by engaging such individuals in real-like world events through simulations. This review study examines the effects of VRI on static and functional balances and summarizes the effective protocols of virtual reality-based rehabilitation interventions for the CP patients.Methods:
A comprehensive search was performed using the following databases: Medline/PubMed, Scopus, Cochrane Library, PEDro, EBSCOhost, and the Online Library of the University of London. The PEDro scale was used to assess the methodological quality. The data extracted from the reviewed studies were coded according to Cooper and Hedges’ guidelines considering the following criteria: (I) Patients' characteristics, (II) intervention protocols, (III) outcomes, and (IV) results. To this end, twelve RCTs with 248 patients aged 4 - 20 years old were analyzed and assessed as "fair" to "good" methodological quality according to Pedro’s scale (4 to 8). VRI alone or in combination with a standard physiotherapy program or with other tools such as tDCS was applied.Results:
Twelve RCTs met the inclusion criteria. The meta-analysis showed the good effect of VRI on the static and functional balances of patients with CP (Cohen’s d = 0.66). The funnel plot revealed no significant asymmetry or heterogeneity among the studies (P = 0.271, I2 = 19.71%), reflecting the absence of publication biases.Conclusions:
This review reports four major perspectives of the VRI applications: (1) VRI settings, (2) selection of exercises, (3) outcome measures, and (4) long-term effects. Moreover, this review summarizes the specific effects of VRI on balance improvement in patients with CP from different perspectives. However, considering the limited number of well-conducted RCTs in this field, a large homogeneous samples size is still needed for future RCTs.Keywords
1. Context
Cerebral palsy (CP) is defined as non-progressive damage affecting the central nervous system in the pre-, neo-, or post-natal periods. It can lead to disturbances in the brain development. Consequently, it can cause sensory and physical deficits, including spasticity, muscle shortness, limited range of motion, and delayed motor performance, as well as perceptual, communicative, and behavioral disturbances (1, 2). Accordingly, these deficits affect motor development, posture, and balance and restrict patients’ ability to perform the activities of daily living (ADL). Reactive balance control is a determinant of performing most functional skills, enabling a child to recover from unexpected balance disturbances (3). Accordingly, CP may also result in poor walking abilities and impaired balance in patients. Previous studies have indicated that neuromuscular strategies related to balance recovery represent specific constraints in patients with CP (4, 5), including inability to adapt the muscle contraction level to the amount of displacement of the center of mass (COM) (6) and the weak muscle activation in the ankle joint in a standing position (7). Furthermore, various factors such as biomechanical constraints significantly affect patients' ability to adjust posture, maintain balance, or perform functional tasks (8). A poor postural control pattern in CP patients is the mal-adaptation of adjustment, characterized by the inappropriate recruitment of antagonist muscles (co-activation) as well as the top-down control of postural muscles (9).
Virtual reality interventions (VRI) have recently been suggested to improve motor function in patients with CP (10). VRI is defined as interactive simulations created by computers to involve patients in real- world like objects and events (11). Three types of virtual reality are provided in the commerce: (1) immersive virtual reality, which gives users the most realistic simulation experience, completed with sight and sound, using headsets and goggles, (2) semi-immersive virtual reality, which is mainly used in educational settings and provides users with a partially virtual environment, and (3) non-immersive virtual reality used in games such as Xbox and Wii-fit plus.
Different rehabilitation techniques could be used to increase motor functional abilities and prevent or delay the complications of patients with CP (12-14). Some rehabilitation techniques are virtual reality-based interventions, described as “viable techniques” (10, 15), and “intensive training tools” based on the interactive stimulations provided by a computer system (11). It aims to optimize patients' motor abilities through repetitive task-oriented practice (10).
As a basis for motor learning, repetition is an essential variable of VRI exercises with potential effects on brain plasticity (16, 17). This, in turn, activates the mirror neurons (18), reorganizes the primary motor cortex (9, 19, 20), and ameliorates the voluntary motor control (21), thereby improving the ADL performance (13). Both neurophysiological and neuropsychological factors in VRI contribute to the progress in motor functions and balance function in CP. Further, VRI is rich in visual, auditory, vestibular, and proprioceptive sensory feedbacks, associated with advancements in postural balance (9, 22).
An increase in the loading of the affected limb of patients with hemiplegia during the VRI training could also increase sensory feedback carried to the cortical sensory areas, consequently causing the higher activation of the motor areas responsible for the postural adjustments and balance maintenance. This hypothesis was evidenced by Son et al. (23), reporting that the compelled weight shift approach facilitated a more symmetrical weight-bearing of patients with stroke and thus promoted their balance (24).
Furthermore, internal motivation and self-esteem were reported to be highly influenced by using VRI (9, 25, 26) and performing competitions (27, 28) in a safe environment (21, 29). Patients with CP have reported enjoyment following only one session of VRI, as well as their increased self-competence and involvement in virtual reality play (30). Thus, VRI using entertaining games and exercises could increase the acceptance and adherence to treatment by family and peers by avoiding the tedious repetitiveness of traditional physiotherapy protocols, mainly if the group therapy program is employed.
A meta-analysis by Chen et al. revealed VRI as an effective technique to improve motor function in general in children with CP (10). Although the present review study aimed to focus specifically on balance function, it failed to make a conclusive recommendation due to the heterogeneity, small sample size, and different protocols used in the included studies. Another systematic review by Warnier et al. was published in 2020 (31), using only two databases (ie, PubMed and Embase); however, they did not analyze the effect of VRI on the balance function of patients with CP and addressed a limited number of studies. Similarly, Wu et al., in a well-designed meta-analysis, showed the positive effect of VRI on the balance of children with CP; however, their suggestion was exclusively based on clinical measures (32).
Two other systematic reviews have recently been published by Montoro-Cardenas et al. and Chesser et al. (33, 34). According to the findings of six articles, the former introduced the Nintendo-Wii as an effective VRI in improving standing balance and gait when used alone or in combination with traditional physiotherapy protocols. Chesser et al. suggested that 30-minute Nintendo-Wii therapy sessions for at least three weeks were an effective virtual reality system in improving functional balance in children with CP (33, 34). Both reviews focused on a single VRI tool (ie, the Nintendo-Wii,) limiting the generalizability of their interesting findings.
Accordingly, no clear evidence exists regarding the effects of VRI on the static and functional balance of patients with CP. In this regard, this review study aimed to systematically discuss the effect of different VRI tools on the static and functional balances of patients with CP with regard to the outcome measures (ie, center of pressure (COP), COM, balance…), intervention protocol, and its long-term effects. Accordingly, this review will summarize the headings of an effective virtual reality-based rehabilitation intervention protocol for patients with CP.
2. Methods
Before conducting the review study, the scientific committee of the Tehran University of Medical Sciences approved this proposal as part of a Ph.D. thesis on Jun 27, 2020. In May 2020, the authors stated, discussed, and detailed the existing review methods, and no deviations were discovered in subsequent phases.
2.1. Eligibility Criteria
We limited our search to randomized control trials (RCTs) in the present review. Inclusion criteria for papers were as follows: (1) published in English, (2) full-text available, (3) published in a peer-reviewed journals, (4) addressing children with various categories of CP, who were aged between 4 - 20 years, (5) comparing the effects of VRI applied alone or in combination with traditional physiotherapy or other techniques versus control intervention, (6) the Physiotherapy Evidence Database scale (PEDro) score > 4/10, and (7) involved intervention studies with pre-and post-assessments using the outcome measures of balance (ie, velocity and standard deviation of the displacement of [COP]_sway, Time Up and Go Test, Gross Motor Function Measures (GMFM- D & E), and Pediatric Balance Scale (PBS)).
2.2. Search Strategy and Data Extraction
A comprehensive search was performed using the following databases from January 2010 till December 2020: Medline/ PubMed, Scopus, Cochrane library, PEDro, EBSCOhost, and the Online Library of the University of London. The review focused on the most updated software and tools used in VRI since it is still a developing technique and encompasses a wide range of games, devices, and applications. Experts in pediatric rehabilitation and VRI were also consulted to confirm our search strategy, particularly the key terms (Appendix 1 in Supplementary File).
Furthermore, the reviewers used reference checking to identify eligible studies not found using our search strategy in any of the abovementioned databases. The authors manually screened the references of all the included studies to check if they met the inclusion criteria.
Two independent reviewers (H. Z. & R. M.) carried out the paper selection and data extraction. In the case of disagreement between the reviewers, a third reviewer (M. R. H.) was involved. Then the data extracted from the included studies were coded into three major categories according to Cooper and Hedges’ guidelines (35). The patients’ age, gender, and CP sub-types were coded as the subjects’ characteristics. Moreover, sample size, VRI settings (eg, commercial, customized), VRI dosing (eg, duration, frequency, length), comparison therapy type, and dosing in the comparison therapy were coded as intervention protocols. Then the outcome measures and the results were allocated two separated columns.
2.3. Quality Assessment
The methodological quality of the included studies was evaluated by two assessors using the PEDro scale. It consists of 11 items and a scale ranging from 1 to 10 (36). The studies were classified following Cashin and McAuley’s study, which considered 0 - 3 as "poor," 4 - 5 as "fair," 6 - 8 as "good," and 9 - 10 as "excellent" methodological quality (37). The decision to include a study was made based on the highest score of the assessors; however, in the case of a significant difference between the assessors’ scores, the third assessor’s decision was considered. As shown in Table 1, the PEDro scores range from “fair” to “good” levels (4 - 8 out of 10).
PEDro Scale for Included Studies
Items | Gatica-Rojas et al. (38) | Lazzari et al. (13) | Grecco et al. (39) | AlSaif and Alsenany (25) | Atasavun Uysal and Baltaci (27) | Arnoni et al. (40) | Wade and Porter (41) | Pin and Butler (42) | Jelsma et al. (43) | Cho et al. (29) | Sharan et al. (17) | Sajan et al. (21) |
---|---|---|---|---|---|---|---|---|---|---|---|---|
1. Eligibility criteria | Y | Y | Y | Y | Y | Y | Y | Y | Y | Y | Y | Y |
2. Random allocation to groups | 1 | 1 | 1 | 1 | 1 | 1 | 1 | 1 | 1 | 1 | 1 | 1 |
3. Concealment | 0 | 1 | 1 | 0 | 0 | 1 | 0 | 1 | 0 | 0 | 0 | 1 |
4. Similarity at baseline | 1 | 1 | 1 | 1 | 1 | 1 | 0 | 1 | 0 | 1 | 0 | 1 |
5. Blinding of subjects | 0 | 1 | 1 | 0 | 0 | 1 | 0 | 0 | 1 | 0 | 1 | 0 |
6. Blinding of therapists | 0 | 1 | 1 | 0 | 0 | 0 | 0 | 0 | 0 | 0 | 0 | 0 |
7. Blinding of assessors | 0 | 0 | 0 | 0 | 1 | 1 | 0 | 1 | 0 | 0 | 0 | 1 |
8. Adequate follow-up | 1 | 1 | 0 | 1 | 0 | 1 | 0 | 1 | 1 | 1 | 0 | 1 |
9. Intention-to-treat analysis | 0 | 0 | 0 | 0 | 0 | 1 | 1 | 1 | 1 | 1 | 0 | 1 |
10. Comparisons between groups | 1 | 1 | 1 | 0 | 1 | 0 | 1 | 0 | 0 | 1 | 1 | 1 |
11. Point measures and measures of variability | 1 | 1 | 1 | 1 | 1 | 1 | 1 | 1 | 1 | 1 | 1 | 1 |
Total score | 5 | 8 | 7 | 4 | 5 | 8 | 4 | 7 | 5 | 6 | 5 | 8 |
2.4. Statistical Analysis
Two authors (H. Z. & R. M.) carried out the meta-analysis using Meta Essentials 1.5. According to Cooper et al. (35), the pooled effect size was estimated at a 95% confidence interval (CI) by using the random-effects models accounting for unexplained heterogeneity (44-46). The pooled effect of the 14 data points from the subgroup analysis was then plotted using the standardized mean difference (SMD) as being more generalizable than the mean difference (MD) (47). It was then interpreted at three strength levels: small (0.2), moderate (0.5), or large (> 0.8) (48).
The degree of inconsistency (I2) was used to assess heterogeneity, classified as small (25 %), moderate (25 - 50 %), and large (> 50 %), with P < 0.1 indicating high heterogeneity (49). The risk of publication bias was evaluated using asymmetry in the funnel plot and Egger's test scores of < 0.1 (50).
3. Results
3.1. Description of Selected Studies
Figure 1 illustrates the strategy used to select the included studies. Three hundred thirty-eight articles were identified during the literature search (PRISMA Flowchart in Figure 1), of which 59 duplicated articles were removed, and 279 were further considered. Out of the concerned studies, 205 articles were excluded after reviewing their titles and abstracts. The remained potentially-relevant articles (n = 74) were screened, and 61 articles were excluded for reasons such as not being RCTs (n = 15) or not recruiting patients with CP (n = 12) (Appendix 2 in Supplementary File). In the concerned papers, 248 CP Patients with GMFCS levels I, II, and III were included. Moreover, spastic hemiplegia, spastic diplegia, and other types of CP in patients with an age range of 4 - 20 years were included in these studies.
PRISMA flow chart. Steps of literature review to select eligible studies.
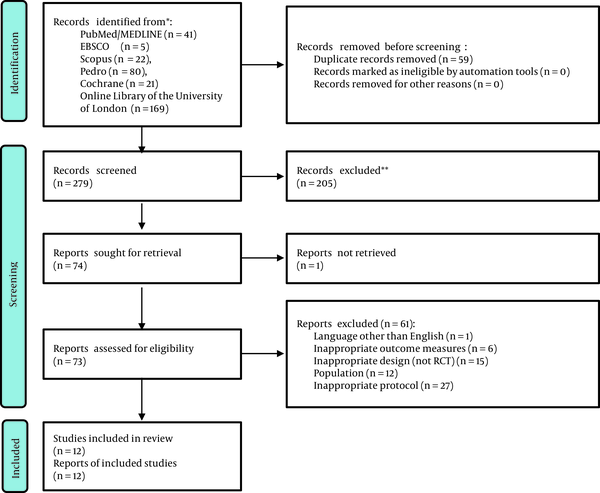
3.2. Findings
This research included 12 RCTs, of which only 8 RCTs were included in our meta-analysis due to the lack of consistency in the outcome measures in those four studies. Four subgroup analyses were performed to estimate the effect of VRI on functional and static balance using PBS, Walking Tests (10-minute-walk-test, 1-minute-walk-test, and 2-minute-walk-test), and GMFM. The pooled effect of GMFM - Items on D & E (SMDD 0.46, 0.13 - 0.79, and SMDE 0.43, -0.07 - 0.93; 95% CI), PBS (SMD 0.64; 95% CI 0.12 - 1.15), and walking test (WT) (SMD 0.98; 95% CI 0.68 - 1.27), as well as the overall pooled effect size (SMD 0.65; 95% CI -0.6 - 1.9) showed moderate evidence for the significant effect of VRI on balance in children with CP. Moreover, the funnel plot shows that the effect size of different outcome measures is in favor of the experimental group (ie, VRI), towards the right side of the graph (Figure 2).
Short term effect of VRI on balance of patients with cerebral palsy. Size effect (Cohen’s d) and heterogeneity (I2) of four clinical outcome measures (GMFM – D, GMFM-E, PBS, and Walking tests)
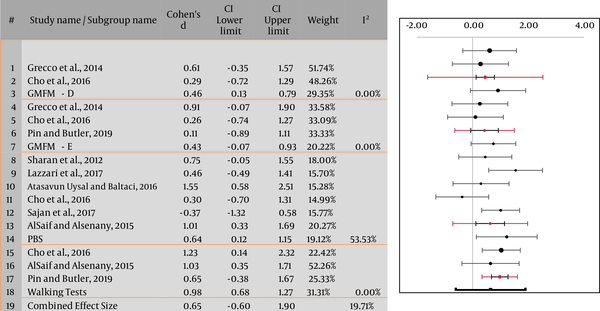
Furthermore, no heterogeneity was detected for GMFM and walking tests (I2 = 0.00%), whereas it was moderate for PBS (I2 = 53.53%). In general, the heterogeneity was low (I2 = 19.71%). The funnel plot appeared to be symmetrical, and Egger's test indicated a low risk of publication bias (P = 0.271) (Figures 2 and 3).
The funnel plot of the effect of VRI on the balance of patients with CP. Publication bias of included studies by distribution of standard error
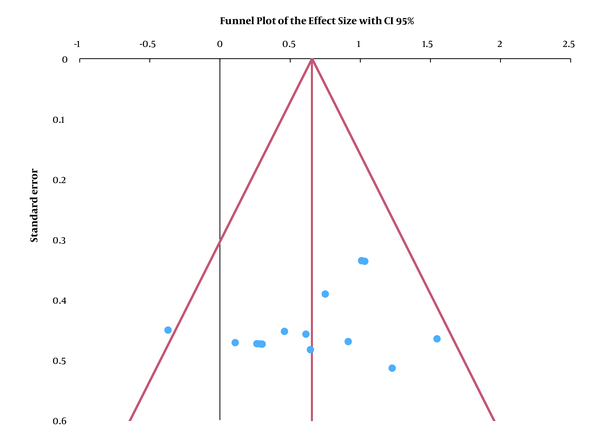
Table 1 shows the results of 12 relevant studies meeting the inclusion criteria. The main effect of VRI on static and functional balance is summarized as follows:
3.3. Immediate Effect on Balance
3.3.1. Effects of VRI on Static Balance
We found only three studies on using COP to detect the changes in the static balance after VRI. The findings were not consistent, and only two studies reported a significant improvement in [COP]_sway when using VRI alone or in combination with other techniques. More significant improvements in the area of [COP]_sway (P < 0.001) were detected by Lazzari et al. having five sessions per week for two weeks of VRI training, combined with unilateral active tDCS compared to VRI training combined with sham tDCS (P = 0.005) (13). These findings were confirmed by Gatica-Rojas et al., concluding that three sessions per week for six weeks of "Wii fit plus" training decreased the [COP]_sway (-2.92 to -4.92; P = 0.02) and the standard deviation of the anteroposterior sway of the COP (SDAP) (-2.92 to 4.92; P = 0.01) (38). In contrast, Arnoni et al. reported no change in postural stability after eight weeks of VRI combined with conventional physiotherapy (P = 0.411) (40).
3.3.2. Effects of VRI on Functional Balance
Functional balance was assessed using clinical tests. Jelsma et al. reported significant changes in the Bruininks-Oseretsky Test of Motor Performance 2 (BOT-2) (F (2, 26) = 9.8286, P = 0.001) with no significant improvement in the Running Speed and Agility test (RSA) (F (2, 26) = 0.86198, P = 0.434) and the Timed Up and Down Stairs Test (TUDS) (F (2, 26) = 1.3862, P = 0.268) after nine weeks of VRI training (43). AlSaif and Alsenany suggested that an intensive protocol of 20 minutes per day of Wii fit games for 12 weeks significantly promoted the balance assessed by the Movement Assessment Battery for Patients-2 (mABC-2) and the 1-minute walk test (ES = 3.4, 95% CI) (25). Similarly, Atasavun Uysal and Baltaci (27), Cho et al., Sharan et al., and Sajan et al. reported significant changes in PBS, the 2-minute walk test, and GMFM- D&E after using different VRI tools (ie, Nintendo Wii fit Training or Virtual Reality Treadmill Training) combined with traditional physiotherapy (17, 21, 29). Grecco et al. documented that GMFM- D & E (ES = 7 and 9.2 respectively, 95% CI) and the PEDI-mobility scale (ES = 0.15, 95% CI) changed significantly when combining tDCS with gait-virtual reality training (39). Finally, Wade and Porter found a significant change in the sitting assessment for children with neuromotor dysfunction (SACND) and the upright sitting of the Chailey Levels of Ability test, reflecting the improvement of functional balance in the sitting position (41). Pin and Butler stated a significant change in trunk muscle co-contraction, with no significant improvement in other outcome measures related to balance (ie, PRT, GMFM-66, and 2-MWT) (42).
3.3.3. Long-term Effects
Although the long-lasting effect of VRI was not addressed as the inclusion criteria in our meta-analysis, the follow-up evaluation was used to assess the long-term effect of VRI. In this regard, the findings were not consistent in the reviewed studies (13, 25, 38, 39, 42). Some authors reported that the improvements declined after one month (38), while others reported that these improvements were maintained over six post-treatment weeks (9, 13, 39). The most extended retention period was reported by Jelsma et al., suggesting that improvement in balance score lasted for a 2-month post-treatment period (P = 0.001) (43).
3.4. Funding of the Studies
Only three studies received no funding from any source. Three other studies did not disclose their funding sources, while the other studies were funded by universities, laboratories, or the ministries. Table 2 summarizes the funding of the included studies.
Funding of Included Studies
Study | Financial Support | |
---|---|---|
1 | Gatica-Rojas et al. (38) | No |
2 | Lazzari et al. (13) | Yes |
3 | Grecco et al. (39) | Yes |
4 | AlSaif and Alsenany (25) | Yes |
5 | Atasavun Uysal and Baltaci (27) | No |
6 | Arnoni et al. (40) | Yes |
7 | Wade and Porter (41) | Yes |
8 | Pin and Butler (42) | Yes |
9 | Jelsma et al. (43) | No |
10 | Cho et al. (29) | Not reported |
11 | Sharan et al. (17) | Not reported |
12 | Sajan et al. (21) | Not reported |
4. Discussion
This review aimed to better understand the effect of VRIs on the static and functional balance of persons with CP. This systematic review reported the possible effect(s) of VRI on the balance variables in the CP patients. In general, the studies with fair to good methodological quality (ie, according to Pedro scores) provided useful recommendations on a protocol for the VRI rehabilitation-based approach in balance improvement.
As mentioned, the present review study provides some evidence on the positive effects of VRI tools such as the Wii-therapy on improving ability to adjust the posture and increase standing balance (ie, reactive and proactive) compared to conventional therapies (29). These findings were supported by the small heterogeneity between studies (I2 = 19.71%) with a good effect size of interventional protocols (Cohen’s d = 0.66,). In the literature, spastic hemiplegic patients demonstrated a reduction in [CoP]_sway and [SD]_AP under the open-eye condition after six weeks of VRI (38). Accordingly, Deutsch et al. suggested that the interaction of visual, vestibular, and proprioceptive systems strongly modified the weight-shifting strategies through the symmetrical distribution of body weight on both lower limbs following VRI (51).
After reviewing the included studies carefully, we noticed that the effects of VRI could be examined from four main perspectives:
4.1. VRI Settings
There is no consensus in the literature regarding the guidelines of VRI usage. Three weeks of interactive videogames were found to significantly improve the balance of the CP patients (25). In another study, Gatica-Rojas and Mendez-Rebolledo suggested that three sessions per week (for six weeks) promoted the standing balance (9). Similarly, Atasavun Uysal and Baltaci reported that 30 minutes of Nintendo Wii games twice a week for 12 weeks, was long enough to improve functional balance (27).
Previous studies reported a relationship between the settings of the intervention protocol and the motor learning improvement (52-54). Accordingly, considering the settings of the used protocols (ie, duration of sessions, number of sessions, and length of intervention) and the rate of patients’ improvements, we found out that increasing the number of sessions (length of protocol) and the amount of practice (number and duration of sessions) may consolidate newly acquired motor tasks (the static and functional balances). A comparison between the protocols used in the included studies and the post-treatment findings shows that the best improvement was detected after applying 20-minute daily sessions of VRI, for 12 weeks (mABC-2; P < 0.05) (25).
This hypothesis was supported by Yamada et al., indicating that an extended practice (ie, several repetitions in different sessions) might help stabilize the new internal model of motor skills and increase the task performance to reach the plateau phase and improve the motor efficiency (52, 53). Accordingly, the more sessions of practice, the greater the re-acquisition or re-expression of a new internal model. In this regard, three sessions per week (for > 30 minutes) may be recommended as an effective protocol for the rehabilitation of children with CP. Furthermore, Shadmehr and Brashers-Krug stated that a novel internal model maintained in the working memory does not persist for a longer period, and it may interfere with other new learned skills. Accordingly, there is an urgent need for an extensive practice to facilitate transfer to the long-term memory or motor memory (55).
Considering the various methods used in the abovementioned studies, we conclude that a minimum of two 20-60-minute sessions per week for at least two weeks of VRI combined with active exercises could be an effective protocol for CP patients in terms of balance improvement.
4.2. Selection of Exercises
Needless to note that the VRI games must be as specific as possible since the clinical improvements are dependent on delivered motor training. In the present review study, different VRI games were used for patients with CP. For example, Gatica-Rojas and Mendez-Rebolledo reported that snowboards, penguin slides, and super Hula Hoop might recruit mediolateral and antero-posterior balance to induce valuable changes in weight-shifting strategies (9). Similarly, Grecco et al. suggested that games encompassing a racetrack to simulate intercalated walking at different velocities, eg, the Your Shape Kinect program (Your Shape: Fitness Evolved 2012 runs the world, Microsoft, USA), could significantly increase the performance scores (39).
To conclude, three main features should be considered when selecting VRI exercises or games: (1) games should be selected as per the patients’ preferences to increase motivation and induce better clinical improvements (27) and elaborate an adequate applied clinical intervention (40), (2) To avoid any adverse effects (39), VRI cannot replace the conventional rehabilitation (29) and it acts better when combined with actual motor practice or neurodevelopmental training tailored to the child's goals and needs (27, 40, 43), and (3) Despite their high costs, customized games, built on each child’s abilities, might be more effective than the commercial products (10, 56).
4.3. Outcome Measures
Two different systems analyzed the improvement in static and functional balances in the literature. The first was underpinned by clinical measures such as standing and walking domains in GMFM-88 or GMFM-66 (25), Time Up-and-Go Test, six-minute walk test, Berg Balance Scale, and others (Appendix 3 in Supplementary File). The second system was mainly based on quantitative tools such as 3-D gait analysis and posturo-graphic analysis (eg, [CoP]_sway, oscillation velocity, the standard deviation in the anteroposterior and mediolateral directions) as well as motion analysis.
Reliable and validated measures are needed in clinical practice to examine the real improvement in the static and functional balances of CP patients. According to our sub-group analysis, moderate evidence was reported about the effect of VRI on the balance function of children with CP, as measured by PBS (I2 = 53.53 %), whereas GMFM (D&E) and walking tests provided sufficient evidence (I2 = 0%). Although quantitative measures are more accurate and objective than qualitative measures, we recommend combining the quantitative and qualitative tests to describe any change in the balance of patients with CP.
On the other hand, we do not believe that a short interval (eg, three weeks) between assessments could accurately reflect the improvement as motor learning is a long-term process. Accordingly, regardless of the outcome measures of the studies, the time interval between assessment and re-assessment is proposed to be extended to avoid the learning effect of biases in the findings (42) and ensure that the child has enough time to improve.
4.4. Long-term Effect of VRI
We failed to set conclusive results from the included studies regarding the long-term effects of VRI. Gatica-Rojas et al. reported that the increased balance control observed in children with spastic hemiplegia declined within 2 - 4 weeks after training (six weeks of VRI, three sessions per week) (38). Wii Fit training for the same category of CP improved balance maintained two months after treatment (nine weeks of VRI, four sessions per week) (43). Accordingly, no study detected the effects of applying a continuous VRI protocol regarding the resting time intervals between practice session; however, as clinicians, we suggest applying VRI periodically (ie, each 3 - 4 weeks of rest, VRI could be re-applied) to avoid any improvement weaning and reduce the time interval between practice sessions to facilitate the transfer of acquired skills from working memory to long-term memory, as Yamanda et al. stated (52).
4.5. Limitations
The generalizability of the present findings might be limited by the heterogeneity and variability among the participants, as well as the small sample size and the limited number of eligible studies reported in this review. According to the authors, inappropriate criteria, the inclusion of a variety of CP sub-categories (ie, different levels of gross motor function classification system (GMFCS) or subtypes), and inability to recruit enough homogenous samples may arouse large variability in post- treatment outcomes (13, 42, 56). Other factors may also pose variability in outcomes. For example, Gatica-Rojas and Mendez-Rebolledo found that spastic diplegia did not significantly improve in standing balance compared to spastic hemiplegia (9). Similarly, Deutsch et al. reported that the reduction of trajectories caused by differences in the severity of impairments also limited their study (51). Accordingly, it is difficult to determine which sub-type of CP might benefit from VRI more than the other sub-types as the included studies encompassed heterogeneous participants from different CP categories. Accordingly, the heterogeneity of participants should be limited by recruiting patients with the same functional level or from the same CP sub-type in future studies.
4.6. Conclusions
This review reports four major perspectives on the application of VRI: (1) the VRI settings, (2) selection of exercises, (3) outcome measures, and (4) long-term effect. Moreover, this review summarizes the specific effects of VRI on the balance improvement in patients with CP from different perspectives. However, considering the limited number of well-conducted RCTs in this field, a large homogeneous samples size is recommended for future RCTs.
References
-
1.
Richards CL, Malouin F. Cerebral palsy: definition, assessment and rehabilitation. Handb Clin Neurol. 2013;111:183-95. [PubMed ID: 23622163]. https://doi.org/10.1016/B978-0-444-52891-9.00018-X.
-
2.
Zhang B, Li D, Liu Y, Wang J, Xiao Q. Virtual reality for limb motor function, balance, gait, cognition and daily function of stroke patients: A systematic review and meta-analysis. J Adv Nurs. 2021;77(8):3255-73. [PubMed ID: 33675076]. https://doi.org/10.1111/jan.14800.
-
3.
Assaiante C, Mallau S, Viel S, Jover M, Schmitz C. Development of postural control in healthy children: a functional approach. Neural Plast. 2005;12(2-3):109-18. discussion 263-72. [PubMed ID: 16097479]. [PubMed Central ID: PMC2565455]. https://doi.org/10.1155/NP.2005.109.
-
4.
Das SP, Ganesh GS. Evidence-based Approach to Physical Therapy in Cerebral Palsy. Indian J Orthop. 2019;53(1):20-34. [PubMed ID: 30905979]. [PubMed Central ID: PMC6394183]. https://doi.org/10.4103/ortho.IJOrtho_241_17.
-
5.
Schmit JM, Riley M, Cummins-Sebree S, Schmitt L, Shockley K. Functional Task Constraints Foster Enhanced Postural Control in Children With Cerebral Palsy. Phys Ther. 2016;96(3):348-54. [PubMed ID: 26112256]. https://doi.org/10.2522/ptj.20140425.
-
6.
Roncesvalles MN, Woollacott MW, Burtner PA. Neural factors underlying reduced postural adaptability in children with cerebral palsy. Neuroreport. 2002;13(18):2407-10. [PubMed ID: 12499838]. https://doi.org/10.1097/00001756-200212200-00006.
-
7.
Ferdjallah M, Harris GF, Smith P, Wertsch JJ. Analysis of postural control synergies during quiet standing in healthy children and children with cerebral palsy. Clin Biomech. 2002;17(3):203-10. https://doi.org/10.1016/s0268-0033(01)00121-8.
-
8.
Pavao SL, dos Santos AN, Woollacott MH, Rocha NA. Assessment of postural control in children with cerebral palsy: a review. Res Dev Disabil. 2013;34(5):1367-75. [PubMed ID: 23466474]. [PubMed Central ID: PMC4157894]. https://doi.org/10.1016/j.ridd.2013.01.034.
-
9.
Gatica-Rojas V, Mendez-Rebolledo G. Virtual reality interface devices in the reorganization of neural networks in the brain of patients with neurological diseases. Neural Regen Res. 2014;9(8):888-96. [PubMed ID: 25206907]. [PubMed Central ID: PMC4146258]. https://doi.org/10.4103/1673-5374.131612.
-
10.
Chen Y, Fanchiang HD, Howard A. Effectiveness of Virtual Reality in Children With Cerebral Palsy: A Systematic Review and Meta-Analysis of Randomized Controlled Trials. Phys Ther. 2018;98(1):63-77. [PubMed ID: 29088476]. [PubMed Central ID: PMC6692882]. https://doi.org/10.1093/ptj/pzx107.
-
11.
Weiss PL, Rand D, Katz N, Kizony R. Video capture virtual reality as a flexible and effective rehabilitation tool. J Neuroeng Rehabil. 2004;1(1):12. [PubMed ID: 15679949]. [PubMed Central ID: PMC546410]. https://doi.org/10.1186/1743-0003-1-12.
-
12.
Han H, Chung Y. Effects of task-oriented training for Gross Motor Function Measure, balance and gait function in persons with cerebral palsy. Phys Ther Rehabil Sci. 2016;5(1):9-14. https://doi.org/10.14474/ptrs.2016.5.1.9.
-
13.
Lazzari RD, Politti F, Belina SF, Collange Grecco LA, Santos CA, Dumont AJL, et al. Effect of Transcranial Direct Current Stimulation Combined With Virtual Reality Training on Balance in Children With Cerebral Palsy: A Randomized, Controlled, Double-Blind, Clinical Trial. J Mot Behav. 2017;49(3):329-36. [PubMed ID: 27644454]. https://doi.org/10.1080/00222895.2016.1204266.
-
14.
Viana RT, Laurentino GE, Souza RJ, Fonseca JB, Silva Filho EM, Dias SN, et al. Effects of the addition of transcranial direct current stimulation to virtual reality therapy after stroke: a pilot randomized controlled trial. NeuroRehabilitation. 2014;34(3):437-46. [PubMed ID: 24473248]. https://doi.org/10.3233/NRE-141065.
-
15.
Chen YP, Lee SY, Howard AM. Effect of virtual reality on upper extremity function in children with cerebral palsy: a meta-analysis. Pediatr Phys Ther. 2014;26(3):289-300. [PubMed ID: 24819682]. https://doi.org/10.1097/PEP.0000000000000046.
-
16.
Ketelaar M, Vermeer A, Hart H, van Petegem-van Beek E, Helders PJ. Effects of a functional therapy program on motor abilities of children with cerebral palsy. Phys Ther. 2001;81(9):1534-45. [PubMed ID: 11688590]. https://doi.org/10.1093/ptj/81.9.1534.
-
17.
Sharan D, Ajeesh PS, Rameshkumar R, Mathankumar M, Paulina RJ, Manjula M. Virtual reality based therapy for post operative rehabilitation of children with cerebral palsy. Work. 2012;41 Suppl 1:3612-5. [PubMed ID: 22317271]. https://doi.org/10.3233/WOR-2012-0667-3612.
-
18.
Rizzolatti G, Craighero L. The mirror-neuron system. Annu Rev Neurosci. 2004;27:169-92. [PubMed ID: 15217330]. https://doi.org/10.1146/annurev.neuro.27.070203.144230.
-
19.
You SH, Jang SH, Kim Y, Kwon Y, Barrow I, Hallett M. Cortical reorganization induced by virtual reality therapy in a child with hemiparetic cerebral palsy. Dev Med Child Neurol. 2005;47(9):628-35. https://doi.org/10.1111/j.1469-8749.2005.tb01216.x.
-
20.
Howcroft J, Klejman S, Fehlings D, Wright V, Zabjek K, Andrysek J, et al. Active video game play in children with cerebral palsy: potential for physical activity promotion and rehabilitation therapies. Arch Phys Med Rehabil. 2012;93(8):1448-56. [PubMed ID: 22571917]. https://doi.org/10.1016/j.apmr.2012.02.033.
-
21.
Sajan JE, John JA, Grace P, Sabu SS, Tharion G. Wii-based interactive video games as a supplement to conventional therapy for rehabilitation of children with cerebral palsy: A pilot, randomized controlled trial. Dev Neurorehabil. 2017;20(6):361-7. [PubMed ID: 27846366]. https://doi.org/10.1080/17518423.2016.1252970.
-
22.
Saxena S, Rao BK, Kumaran S. Analysis of postural stability in children with cerebral palsy and children with typical development: an observational study. Pediatr Phys Ther. 2014;26(3):325-30. [PubMed ID: 24979087]. https://doi.org/10.1097/PEP.0000000000000060.
-
23.
Son SM. Effects of Compelled Weight Shift on Balance Ability in Patients with Stroke. J Korean Phys Ther. 2017;29(5):255-8. https://doi.org/10.18857/jkpt.2017.29.5.255.
-
24.
Aruin AS, Rao N, Sharma A, Chaudhuri G. Compelled body weight shift approach in rehabilitation of individuals with chronic stroke. Top Stroke Rehabil. 2012;19(6):556-63. [PubMed ID: 23192720]. [PubMed Central ID: PMC3676671]. https://doi.org/10.1310/tsr1906-556.
-
25.
AlSaif AA, Alsenany S. Effects of interactive games on motor performance in children with spastic cerebral palsy. J Phys Ther Sci. 2015;27(6):2001-3. [PubMed ID: 26180367]. [PubMed Central ID: PMC4500030]. https://doi.org/10.1589/jpts.27.2001.
-
26.
Harris K, Reid D. The influence of virtual reality play on children's motivation. Can J Occup Ther. 2005;72(1):21-9. [PubMed ID: 15727045]. https://doi.org/10.1177/000841740507200107.
-
27.
Atasavun Uysal S, Baltaci G. Effects of Nintendo Wii() Training on Occupational Performance, Balance, and Daily Living Activities in Children with Spastic Hemiplegic Cerebral Palsy: A Single-Blind and Randomized Trial. Games Health J. 2016;5(5):311-7. [PubMed ID: 27705006]. https://doi.org/10.1089/g4h.2015.0102.
-
28.
Sandlund M, Waterworth EL, Hager C. Using motion interactive games to promote physical activity and enhance motor performance in children with cerebral palsy. Dev Neurorehabil. 2011;14(1):15-21. [PubMed ID: 21241174]. https://doi.org/10.3109/17518423.2010.533329.
-
29.
Cho C, Hwang W, Hwang S, Chung Y. Treadmill Training with Virtual Reality Improves Gait, Balance, and Muscle Strength in Children with Cerebral Palsy. Tohoku J Exp Med. 2016;238(3):213-8. [PubMed ID: 26947315]. https://doi.org/10.1620/tjem.238.213.
-
30.
Reid DT. Benefits of a virtual play rehabilitation environment for children with cerebral palsy on perceptions of self-efficacy: a pilot study. Pediatr Rehabil. 2002;5(3):141-8. [PubMed ID: 12581476]. https://doi.org/10.1080/1363849021000039344.
-
31.
Warnier N, Lambregts S, Port IV. Effect of Virtual Reality Therapy on Balance and Walking in Children with Cerebral Palsy: A Systematic Review. Dev Neurorehabil. 2020;23(8):502-18. [PubMed ID: 31674852]. https://doi.org/10.1080/17518423.2019.1683907.
-
32.
Wu J, Loprinzi PD, Ren Z. The Rehabilitative Effects of Virtual Reality Games on Balance Performance among Children with Cerebral Palsy: A Meta-Analysis of Randomized Controlled Trials. Int J Environ Res Public Health. 2019;16(21). [PubMed ID: 31661938]. [PubMed Central ID: PMC6861947]. https://doi.org/10.3390/ijerph16214161.
-
33.
Chesser BT, Blythe SA, Ridge LD, Tomaszewski RE, Kinne BL. Effectiveness of the Wii for pediatric rehabilitation in individuals with cerebral palsy: a systematic review. Phys Ther Rev. 2020;25(2):106-17. https://doi.org/10.1080/10833196.2020.1740402.
-
34.
Montoro-Cardenas D, Cortes-Perez I, Zagalaz-Anula N, Osuna-Perez MC, Obrero-Gaitan E, Lomas-Vega R. Nintendo Wii Balance Board therapy for postural control in children with cerebral palsy: a systematic review and meta-analysis. Dev Med Child Neurol. 2021;63(11):1262-75. [PubMed ID: 34105150]. https://doi.org/10.1111/dmcn.14947.
-
35.
Cooper H, Hedges LV, Valentine JC. Handbook of Research Synthesis and Meta-Analysis. Russell Sage Foundation; 2009.
-
36.
PEDro. Summary of measurement properties of the PEDro scale. PEDro; 2019, [cited 3/12/2021]. Available from: https://pedro.org.au/english/summary-of-measurement-properties-of-the-pedro-scale/.
-
37.
Cashin AG, McAuley JH. Clinimetrics: Physiotherapy Evidence Database (PEDro) Scale. J Physiother. 2020;66(1):59. [PubMed ID: 31521549]. https://doi.org/10.1016/j.jphys.2019.08.005.
-
38.
Gatica-Rojas V, Mendez-Rebolledo G, Guzman-Munoz E, Soto-Poblete A, Cartes-Velasquez R, Elgueta-Cancino E, et al. Does Nintendo Wii Balance Board improve standing balance? A randomized controlled trial in children with cerebral palsy. Eur J Phys Rehabil Med. 2017;53(4):535-44. [PubMed ID: 27882910]. https://doi.org/10.23736/S1973-9087.16.04447-6.
-
39.
Grecco LA, de Almeida Carvalho Duarte N, Mendonca ME, Cimolin V, Galli M, Fregni F, et al. Transcranial direct current stimulation during treadmill training in children with cerebral palsy: a randomized controlled double-blind clinical trial. Res Dev Disabil. 2014;35(11):2840-8. [PubMed ID: 25105567]. https://doi.org/10.1016/j.ridd.2014.07.030.
-
40.
Arnoni JLB, Pavao SL, Dos Santos Silva FP, Rocha N. Effects of virtual reality in body oscillation and motor performance of children with cerebral palsy: A preliminary randomized controlled clinical trial. Complement Ther Clin Pract. 2019;35:189-94. [PubMed ID: 31003657]. https://doi.org/10.1016/j.ctcp.2019.02.014.
-
41.
Wade W, Porter D. Sitting playfully: does the use of a centre of gravity computer game controller influence the sitting ability of young people with cerebral palsy? Disabil Rehabil Assist Technol. 2012;7(2):122-9. [PubMed ID: 21967300]. https://doi.org/10.3109/17483107.2011.589485.
-
42.
Pin TW, Butler PB. The effect of interactive computer play on balance and functional abilities in children with moderate cerebral palsy: a pilot randomized study. Clin Rehabil. 2019;33(4):704-10. [PubMed ID: 30599772]. https://doi.org/10.1177/0269215518821714.
-
43.
Jelsma J, Pronk M, Ferguson G, Jelsma-Smit D. The effect of the Nintendo Wii Fit on balance control and gross motor function of children with spastic hemiplegic cerebral palsy. Dev Neurorehabil. 2013;16(1):27-37. [PubMed ID: 23030836]. https://doi.org/10.3109/17518423.2012.711781.
-
44.
DerSimonian R, Laird N. Meta-analysis in clinical trials. Control Clin Trials. 1986;7(3):177-88. https://doi.org/10.1016/0197-2456(86)90046-2.
-
45.
Barili F, Parolari A, Kappetein PA, Freemantle N. Statistical Primer: heterogeneity, random- or fixed-effects model analyses? Interact Cardiovasc Thorac Surg. 2018;27(3):317-21. [PubMed ID: 29868857]. https://doi.org/10.1093/icvts/ivy163.
-
46.
Olkin I; Hedges. Statistical Methods for Meta-Analysis. Academic Press; 2014.
-
47.
Takeshima N, Sozu T, Tajika A, Ogawa Y, Hayasaka Y, Furukawa TA. Which is more generalizable, powerful and interpretable in meta-analyses, mean difference or standardized mean difference? BMC Med Res Methodol. 2014;14:30. [PubMed ID: 24559167]. [PubMed Central ID: PMC3936842]. https://doi.org/10.1186/1471-2288-14-30.
-
48.
Cohen J. Statistical Power Analysis for the Behavioral Sciences. 2nd ed. New York, USA: Routledge; 1988.
-
49.
Higgins JP, Thompson SG, Deeks JJ, Altman DG. Measuring inconsistency in meta-analyses. BMJ. 2003;327(7414):557-60. [PubMed ID: 12958120]. [PubMed Central ID: PMC192859]. https://doi.org/10.1136/bmj.327.7414.557.
-
50.
Sterne JA, Egger M. Funnel plots for detecting bias in meta-analysis. J Clin Epidemiol. 2001;54(10):1046-55. https://doi.org/10.1016/s0895-4356(01)00377-8.
-
51.
Deutsch JE, Borbely M, Filler J, Huhn K, Guarrera-Bowlby P. Use of a low-cost, commercially available gaming console (Wii) for rehabilitation of an adolescent with cerebral palsy. Phys Ther. 2008;88(10):1196-207. [PubMed ID: 18689607]. https://doi.org/10.2522/ptj.20080062.
-
52.
Yamada C, Itaguchi Y, Fukuzawa K. Effects of the amount of practice and time interval between practice sessions on the retention of internal models. PLoS One. 2019;14(4). e0215331. [PubMed ID: 30990823]. [PubMed Central ID: PMC6467396]. https://doi.org/10.1371/journal.pone.0215331.
-
53.
Criscimagna-Hemminger SE, Shadmehr R. Consolidation patterns of human motor memory. J Neurosci. 2008;28(39):9610-8. [PubMed ID: 18815247]. [PubMed Central ID: PMC2665258]. https://doi.org/10.1523/JNEUROSCI.3071-08.2008.
-
54.
Krakauer JW, Shadmehr R. Consolidation of motor memory. Trends Neurosci. 2006;29(1):58-64. [PubMed ID: 16290273]. [PubMed Central ID: PMC2553888]. https://doi.org/10.1016/j.tins.2005.10.003.
-
55.
Shadmehr R, Brashers-Krug T. Functional stages in the formation of human long-term motor memory. J Neurosci. 1997;17(1):409-19. [PubMed ID: 8987766]. [PubMed Central ID: PMC6793707].
-
56.
Bonnechere B, Jansen B, Omelina L, Degelaen M, Wermenbol V, Rooze M, et al. Can serious games be incorporated with conventional treatment of children with cerebral palsy? A review. Res Dev Disabil. 2014;35(8):1899-913. [PubMed ID: 24794289]. https://doi.org/10.1016/j.ridd.2014.04.016.