Abstract
Background:
This study aimed to assess the test-retest intra-rater reliability and convergent validity of digital photography (DP) in detecting the postural orientation of children with cerebral palsy (CP).Methods:
The study recruited children with various types of CP with the Gross Motor Function Classification System level I or II and spasticity < 2 on the Ashworth Scale, without any visual or cognitive impairments. Children who had undergone any surgical intervention or received a botulinum toxin injection within the previous six months were excluded. A digital camera was fixed at 1.5 meters from the participants at the height of 90 cm. Non-reflective markers were attached to eight anatomical landmarks to localize the upper and lower center of mass on both sides. The same examiner took three digital photos to detect intra-rater reliability using the intraclass correlation coefficient (ICC). Pearson's correlation and linear regression analysis were used to assess the convergent validity of the DP method compared with the Pediatric Balance Scale (PBS) scores.Results:
Thirty children (7.44 ± 2.38 years) were assessed to test the reliability of DP, and 55 others (8.06 ± 2.19 years) participated in the convergent validity study. Intra-rater reliability was found to be perfect (ICC > 0.995) and there was a strong significant negative correlation between DP measures and PBS scores (Pearson's correlation > 0.75) with high adjusted R2 (R2 > 0.567), indicating goodness of fit between the measures.Conclusions:
Digital photography (DP) is a reliable and valid method for assessing postural orientation in children with various types of CP.Keywords
Cerebral Palsy Center of Mass Digital Photography Balance Pediatric Balance Scale
1. Background
Cerebral palsy (CP) refers to a group of permanent non-progressive disorders of the central nervous system that cause a variety of disturbances in the development of children's movement and posture (1, 2). Deficits in the visual, somatosensory, and vestibular systems (2, 3) are among the major limitations of CP, resulting in a decrease in anticipatory and reactive postural adjustments (4) and manifesting as limited postural control, weak balance, and difficulties maintaining balance during daily activities (5). Consequently, the rehabilitation protocols aim to improve postural control in children with CP (CwCP) to increase their participation in daily life activities.
It is worth noting that postural control is no longer analyzed as a simple sum of static reflexes but as a complex skill based on the interaction of dynamic sensorimotor processes. Postural orientation and postural stability (balance or equilibrium) are the two primary functional patterns of postural behavior. The active alignment of the trunk and head concerning gravity, support surfaces, the visual surround, and internal references is referred to as postural orientation. Also, postural stability refers to the coordination of movement strategies used to stabilize the center of body mass during both self-initiated and externally induced disturbances of stability (6, 7).
According to Dewar et al., research should focus on establishing links between postural control impairments, treatment options, and outcome measures (4). Accordingly, technological advances in digital photogrammetry systems have facilitated body posture assessment for researchers and practitioners (8). Within this context, three-dimensional (3D) motion-tracking systems, such as Vicon and Qualisys, are the most accurate and valid methods for evaluating human movement and posture (9, 10). However, their costs and technical requirements (space, time, data processing, and user expertise) limit their practical application (11, 12). Instead, two-dimensional (2D) photosystems are expected to be less expensive and easier to use for assessing postural adjustments (11).
Despite the fact that 2D techniques are a valid, consistent, and reliable tool for assessing various domains in comparison to 3D systems (13-15), research on the use of 2D digital photography (DP) systems with CwCP is limited. Thus, the aim of this study was twofold: (1) to evaluate the test-retest intra-rater reliability of DP-based outcomes (i.e., total COM); and (2) to determine the convergent validity of the DP COM-based measures as a predictor of improvement in postural orientation in CwCP when compared to clinical measures.
3. Methods
A methodological study was conducted to assess the intra-rater reliability and convergent validity of DP in the postural analysis of CwCP. The protocol of this study was approved by the ethical and scientific committees of the Tehran University of Medical Sciences (ID: IR.TUMS.VCR.REC.1399.537).
3.1. Participants
The inclusion criteria included children with CP aged 4 to 12 with monoplegia, hemiplegia, or diplegia with the Gross Motor Function classification system level I or II and spasticity < 2 on the Ashworth scale.
Children with visual or cognitive impairments and those with any surgical intervention (osteotomy, tenotomy, or others) or Botulinum Toxin injection in the previous six months were excluded.
Before data collection, the children's parents were asked to sign an informed written consent.
3.2. Procedures
3.2.1. Digital Photography (DP) Measures
3.2.1.1. Digital Images
For detecting the reliability and validity of DP systems, the protocol described by Vander Linden et al. and approved by Scholz et al. was used (14, 16, 17). Eligible participants attempted to keep their feet parallel in their natural standing posture. Square non-reflective markers (1.5 * 1.5 cm) were attached to eight anatomical landmarks of each participant's upper and lower limbs, as shown in Figure 1. The landmarks were chosen to help localize the center of mass (COM).
A, The standardized position of patients with the anatomical landmarks; B, COM vectors.
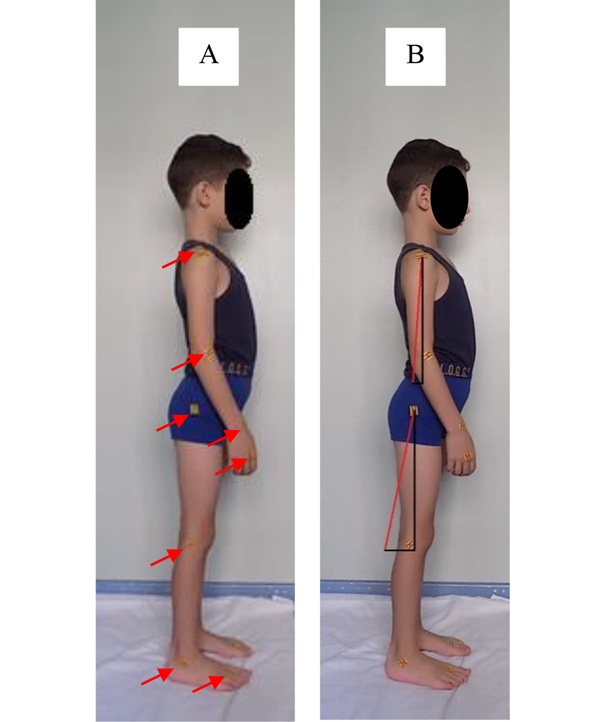
The camera was fixed on a tripod in a standardized position at the height of 90 cm at 1.5 meters from the participants, over fixed markers on the floor, to improve the consistency of DP.
The digital camera used was a NIKON COOLPIX L340 (Nikon Inc, Melville, NY) with a zoom lens ranging from 18 to 200 mm. The images were captured with a focal length of 4 mm and an aperture of F 3.1. The resolution (two megapixels) was combined with a convergently low ISO (400) to ensure clarity in locating measurement landmarks.
3.2.1.2. Localization of the Center of Mass
The participants were asked to dress lightly before taking digital photos to better identify anatomical landmarks. Two expert physiotherapists (with more than five years of experience in pediatric rehabilitation) localized the anatomical landmarks manually. The acromion, lateral epicondyle, radial styloid, and base of the third metacarpi were then identified and highlighted using non-reflective markers to locate the participants' upper COM (i.e., location of COM from the shoulder). Four other markers were attached to the greater trochanter, lateral condyle, lateral malleolus, and the base of the second metatarsal bone (i.e., location of COM from the greater trochanter) to detect lower COM. Before measurement, all digital images were de-identified and saved in JPEG format (5152 * 3864 pixels, 8-bit RGB JPEG).
The coordinates of the landmarks mentioned above were identified using the free MicroDicom viewer software. Following that, the data were computed in a specific program designed in Excel to calculate the coordinates of COM at each segment of the upper and lower limbs using Equations 1 and then the total COM locations using Equations 3 (18):
Where X and Y refer to the coordinates of the landmarks, length % refers to the percentage of the total segment length, and (m) is the mass of each body segment, referring to the anthropometric data of Winter (18), as mentioned in Appendix 1.
Subsequently, the vectors of total upper and lower COM were computed using an objective method of the evaluation of Euclidean distance among the joint coordinates, including subtracting the x and y dimensions of COM from the x and y dimensions of shoulder and hip joints followed by taking root mean square of the sum of the results according to the following equation:
Then, each vector (i.e., the vectors of right and left upper and lower COM) was drawn on the same photo, as shown in Figure 2.
Linear regression analysis charts of DP measures and PBS scores; (X refers to the vector of COM, and Y refers to the PBS scores in each graph).
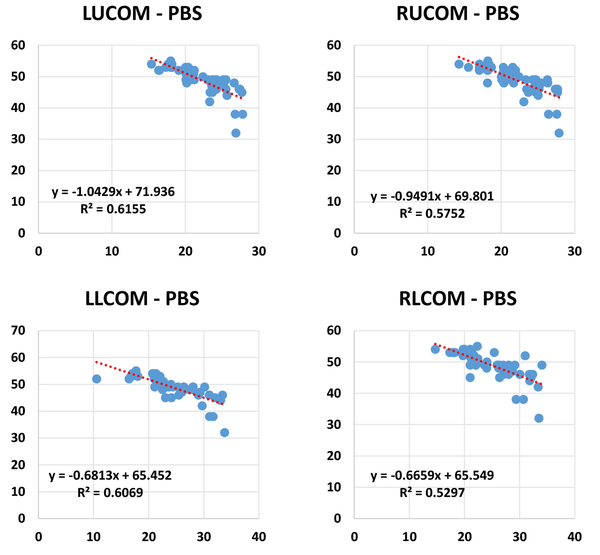
3.2.2. Pediatric Balance Scale Measures
The Pediatric Balance Scale (PBS), a modified version of the Berg Balance Scale, was used by a second assessor to evaluate the balance of all participants in the validity study (19). It consists of 14 items scored from 0 to 4, with 0 being the lowest function and 4 being the highest, for a total of 56 points (20). Pediatric balance scale (PBS) is a reliable and valid tool for measuring balance in children under 15 years with mild to moderate motor impairments, with an intraclass correlation coefficient (ICC) = 0.998 for test-retest reliability and 0.997 for inter-rater reliability (19).
3.3. Statistical Analysis
Data from three digital photographs for each side (i.e., left and right) of the participants were analyzed statistically for intra-rater reliability. The coordinates of the eight anatomical landmarks were computed, and the total COM vectors (upper and lower COM) were then calculated and coded as the right-upper center of mass (RUCOM), the left-upper center of mass (LUCOM), the right-lower center of mass (RLCOM), and the left-lower center of mass (LLCOM).
Thus, two separate analyses were performed. The first analysis involved investigating the intra-rater reliability of DP using ICC (21). The absolute reliability was calculated using the standard error of measurement (SEM), defined in the formula
Interpretation of this correlation was based on Dancy and Reidy’s classification for Pearson's coefficients described as follows: perfect correlation represented by coefficients between 0.9 and 1, strong correlation represented by coefficients between 0.70 and 0.89, and moderate correlation indicated by coefficients less than 0.7 (24). Statistical analyses were conducted using SPSS for Windows, version 23 (SPSS Inc., Chicago, Illinois, USA).
4. Results
4.1. Intra-rater Reliability
Intra-rater reliability has been defined as the consistency in measuring a constant phenomenon when the same individual is measuring its variables (25).
Thirty CwCP (eight females and 22 males) with a mean age of 7.44 (2.38) years were recruited for the reliability test. According to GMFCS-RE, 24 participants were classified as level 1, and six participants were classified as level II. Height and weight were also measured, with an average of 114 (19.8) cm and 24.8 (9.27) kg. Three children were classified as having monoplegia, twelve as having diplegia, ten as having left spastic hemiplegia, and five as having right spastic hemiplegia (Table 1).
Demographic Data of the Participants
Variables | Reliability Study | Validity Study |
---|---|---|
N | ||
F | 8 | 15 |
M | 22 | 40 |
Total | 30 | 55 |
Diagnosis | ||
Mono | 3 | 6 |
Di | 12 | 15 |
LSH | 10 | 25 |
RSH | 5 | 9 |
Age (y) | 7.44 (2.38) | 8.06 (2.19) |
Height (cm) | 114 (19.8) | 118.5 (16.9) |
Weight (kg) | 24.8 (9.27) | 26.5 (8.5) |
GMFCS | ||
I | 24 | 42 |
II | 6 | 13 |
COM | ||
LUCOM | 3.69 (0.23) | 22.1 (3.27) |
RUCOM | 4.53 (0.31) | 21 (3.48) |
LLCOM | 4.94 (0.46) | 24.25 (4.98) |
RLCOM | 6 (0.52) | 24.96 (4.76) |
According to De Vaus's classification for reliability coefficients, the ICC measurements for the three trials performed by the examiner showed perfect correlations with values greater than 0.91 for all four measures of the vector of COM (95% CI: LUCOM 0.996, RUCOM 0.995, LLCOM 0.991, RLCOM 0.992). Both SEM and MDC values showed high test-retest reliability for DP (0.15° ≤ SEM ≥ 0.452°; 0.424° ≤ MDC ≥ 1.254°). All measurements were also highly significant, with P-value < 0.01 (Table 2).
The Intra-rater Reliability of digital photography (DP) Tool for the Vector of Center of Mass
Variables | ICC | Lower | Upper | Approx. ± | SEM | MDC | CV | P Value |
---|---|---|---|---|---|---|---|---|
LUCOM | 0.996 | 0.990 | 1.000 | 0.000 | 0.153 | 0.424 | 1.060 | 0.00000 |
RUCOM | 0.995 | 0.990 | 1.000 | 0.000 | 0.216 | 0.600 | 1.457 | 0.00000 |
LLCOM | 0.991 | 0.980 | 1.000 | 0.010 | 0.435 | 1.206 | 1.803 | 0.00001 |
RLCOM | 0.992 | 0.980 | 1.000 | 0.010 | 0.452 | 1.254 | 2.167 | 0.00001 |
4.2. Convergent Validity
Convergent validity refers to the degree of consistency in results between a new tool or scale compared to other measures of the same variables or patterns (26). Emma Stokes recommended using convergent validity to correlate the scores obtained on a new instrument with a gold standard or non-gold-standard measurements of similar domains (26-28).
Fifty-five CwCP (15 females and 40 males) with a mean age of 8.06 (2.19) years were recruited for the convergent validity study. According to GMFCS-RE, 42 participants were classified as level I, and 13 participants were classified as level II. Of the participants, six had monoplegia, 15 had diplegia, 25 had left spastic hemiplegia, and nine had right spastic hemiplegia (Table 1).
There were moderate to strong negative significant correlations between the four COM measurements (LUCOM, RUCOM, LLCOM, and RLCOM) and the PBS scores, according to Dancy and Reidy's correlation classification, with 0.728 < Pearson's correlation > 0.785 at P-value 0.01.
On the other hand, and regardless of the comparison with the pediatric balance scale, there was a strong to perfect positive significant correlation between the COM measurements (0.75 < Pearson's correlation > 0.93, P-value < 0.01) (Table 3).
Convergent Validity and Linear Regression Analysis of Digital Photography (DP) Measures and Pediatric Balance Scale (PBS) Scores
Variables | N | Pearson's Correlation | Linear Regression Analysis | ||
---|---|---|---|---|---|
Pearson Correlation | Sig. (2-tailed) | Equations | R2 | ||
LUCOM - PBS | 55 | -0.785 a | 0.000 | Y = -1.0429X + 71.936 | 0.6155 |
RUCOM – PBS | 55 | -0.758 a | 0.000 | Y = -0.9491X + 69.801 | 0.5752 |
LLCOM - PBS | 55 | -0.779 a | 0.000 | Y = -0.6813X + 65.452 | 0.6069 |
RLCOM - PBS | 55 | -0.728 a | 0.000 | Y = -0.6659X + 65.549 | 0.5297 |
Furthermore, linear regression analysis revealed a high R2 (> 0.567), indicating goodness of fit between data of both measures, and more than 56% of total COM measures were explained by PBS scores (see plots of each measure in Figure 2). As the P-value measured through the t-statistic was less than 0.05 (P-value = 0.00), we suggest that PBS as the independent variable is statistically significant and can be included in the model as a good predictor of total COM. Moreover, linear regression analysis allowed the authors to develop correlation equations between both measures, enabling them to predict one's value based on the second scale score, as shown in Table 3 and Figure 2.
5. Discussion
To the best of our knowledge, this is the first study to assess the convergent validity and intra-rater reliability of the DP measures of total COM as a tool for detecting the postural orientation of children with various types of CP.
Our findings revealed a perfect correlation for all COM measurements with ICC > 0.9 (CI = 95%) and a likely variation of SEM less than 1° (0.15° ≤ SEM ≥ 0.452°), along with a small MDC (0.424° ≤ MDC ≥ 1.254°), demonstrating good repeatability of DP measures. These results support previous research indicating that DP is highly consistent and reliable in the clinical detection of postural patterns (29-32). Our results are very close to what Ashnagar et al. reported when studying the reliability of DP for assessing lower extremity alignment in individuals with flatfeet and normal feet types (1.25 - 2.78) (33). Similarly, Matamalas et al. suggested strong intra-rater reliability of DP when used to assess idiopathic scoliosis with ICC values > 0.80 (34). Stolinski et al. also reported very good intra-reliability in the measurement of sagittal trunk alignment, with SEMs ranging between 0.7 and 1.3 (8).
Koozekanani et al., on the other hand, introduced a new approach to analyzing postural stability in 1980 by discussing the stability boundaries and the proximity of the center of pressure, as the projection of the total COM in the base of support, to the boundaries as a key element in maintaining balance and preventing falling (35). Later, Dutt-Mazumder et al. reported that increasing the slop angle increased the center of pressure-CoP sway of a healthy individual (displacement) and the virtual time to collision (VTC) dynamics. It revealed that postural orientation and body alignment could be measured by displacing CoP in a standing position and VTC with the functional stability boundary (36).
In the current study, a strong significant negative correlation was detected between the DP measurements of total COM and the PBS scores of CwCP (0.728 < Pearson's correlation > 0.785 with R2 ranging from 0.52 to 0.61 at P-value 0.01). As a result, a high score of PBS is associated with a small length of the vector of total COM, indicating that total COM is becoming closer to the body, showing an improvement in the children's postural orientation. These findings are more significant than previous findings. Matamalas et al. reported a poor to moderate correlation between DP and radiography in evaluating shoulder balance in idiopathic scoliosis, with R2 ranging from 0.26 to 0.51 (34). However, Nix et al. suggested a perfect correlation of both techniques in measuring the hallux valgus angle, with correlations ranging from 0.96 to 0.98 (30). Furthermore, with ICC values greater than 0.84, Cobb et al. reported moderate to strong concurrent validity between the clinical and DP measurement methods (37).
Moreover, the limited discrepancy between DP and PBS, as measured by the linear regression analysis, and the strong correlation between the two measures allow us to consider this amount of difference an appropriate clinical standard for our analysis. Thus, digital photographic measurements of postural orientation can be used as an alternative to other clinical measures validated for measuring the balance in the standing position of CwCP.
As a strength of this study, we recommend DP, which is simple, easy to apply, non-invasive, and inexpensive, for detecting postural orientation in CwCP when performing 3D-motion analysis is difficult or impossible. Furthermore, having a digital image in the archive allows clinicians to track patients' progress prospectively and retrospectively over time.
When applying these findings in the clinic, several limitations should be considered. Subject positioning, subject cooperation, camera placement, and identification of landmarks in photos are all potential sources of error when using this method. We used each subject's natural standing position as a standardized position that can easily be replicated in a clinical setting. Therefore, since our study was not concerned with validating the standardized camera position appropriate for clinical use, further work is required to examine this parameter.
Another significant limitation was the homogeneity of the participants. In this study, a small sample of participants with monoplegia, hemiplegia, and diplegia (n = 30 for reliability and n = 55 for validity) was recruited. As a result, the analysis was not based on CP sub-groups, which could be considered a source of bias given the difference in the impact of the damage (i.e., monoplegia, hemiplegia, diplegia) on CwCP balance strategies. Accordingly, it is essential to note that further investigation of the reliability and validity of DP by CP subgroups may be more relevant for clinical use.
5.1. Conclusions
Clinical photography is a reliable and valid method for assessing postural orientation in children with different types of CP. Intra-rater reliability is perfect, with ICC values greater than 0.9. Furthermore, a comparison of the DP measures of total COM with PBS scores revealed a strong significant negative correlation, allowing the authors to develop an equation based on regression analysis to link the two measurements. Based on our findings, we propose to use DP as an alternative tool for assessing postural patterns in CwCP.
References
-
1.
Blair E, Love S. Definition and classification of cerebral palsy. Dev Med Child Neurol. 2005;47(8):510. https://doi.org/10.1017/S0012162205241002.
-
2.
Montoro-Cardenas D, Cortes-Perez I, Zagalaz-Anula N, Osuna-Perez MC, Obrero-Gaitan E, Lomas-Vega R. Nintendo Wii Balance Board therapy for postural control in children with cerebral palsy: a systematic review and meta-analysis. Dev Med Child Neurol. 2021;63(11):1262-75. [PubMed ID: 34105150]. https://doi.org/10.1111/dmcn.14947.
-
3.
Riquelme I, Montoya P. Developmental changes in somatosensory processing in cerebral palsy and healthy individuals. Clin Neurophysiol. 2010;121(8):1314-20. [PubMed ID: 20363181]. https://doi.org/10.1016/j.clinph.2010.03.010.
-
4.
Dewar R, Love S, Johnston LM. Exercise interventions improve postural control in children with cerebral palsy: a systematic review. Dev Med Child Neurol. 2015;57(6):504-20. [PubMed ID: 25523410]. https://doi.org/10.1111/dmcn.12660.
-
5.
Imms C. Children with cerebral palsy participate: a review of the literature. Disabil Rehabil. 2008;30(24):1867-84. [PubMed ID: 19037780]. https://doi.org/10.1080/09638280701673542.
-
6.
Horak FB. Postural Control. In: Binder MD, Hirokawa N, Windhorst U, editors. Encyclopedia of Neuroscience. Berlin, Heidelberg: Springer; 2009. p. 3212-9. https://doi.org/10.1007/978-3-540-29678-2_4708.
-
7.
Horak FB. Postural orientation and equilibrium: what do we need to know about neural control of balance to prevent falls? Age Ageing. 2006;35 Suppl 2:ii7-ii11. [PubMed ID: 16926210]. https://doi.org/10.1093/ageing/afl077.
-
8.
Stolinski L, Kozinoga M, Czaprowski D, Tyrakowski M, Cerny P, Suzuki N, et al. Two-dimensional digital photography for child body posture evaluation: standardized technique, reliable parameters and normative data for age 7-10 years. Scoliosis Spinal Disord. 2017;12:38. [PubMed ID: 29276784]. [PubMed Central ID: PMC5738151]. https://doi.org/10.1186/s13013-017-0146-7.
-
9.
Padulo J, Vando S, Chamari K, Chaouachi A, Bagno D, Pizzolato F. Validity of the MarkWiiR for kinematic analysis during walking and running gaits. Biol Sport. 2015;32(1):53-8. [PubMed ID: 25729150]. [PubMed Central ID: PMC4314604]. https://doi.org/10.5604/20831862.1127282.
-
10.
Zhou H, Hu H. Human motion tracking for rehabilitation—A survey. Biomed Signal Process Control. 2008;3(1):1-18. https://doi.org/10.1016/j.bspc.2007.09.001.
-
11.
Damsted C, Nielsen RO, Larsen LH. Reliability of video-based quantification of the knee- and hip angle at foot strike during running. Int J Sports Phys Ther. 2015;10(2):147-54. [PubMed ID: 25883863]. [PubMed Central ID: PMC4387722].
-
12.
Littrell ME, Chang YH, Selgrade BP. Development and Assessment of a Low-Cost Clinical Gait Analysis System. J Appl Biomech. 2018;34(6):503-8. [PubMed ID: 29989476]. https://doi.org/10.1123/jab.2017-0370.
-
13.
Belyea BC, Lewis E, Gabor Z, Jackson J, King DL. Validity and Intrarater Reliability of 2-Dimensional Motion Analysis Using a Handheld Tablet Compared to Traditional 3-Dimensional Motion Analysis. J Sport Rehabil. 2015;24(4). [PubMed ID: 25612081]. https://doi.org/10.1123/jsr.2014-0194.
-
14.
Haggard P, Wing AM. Assessing and reporting the accuracy of position measurements made with optical tracking systems. J Mot Behav. 1990;22(2):315-21. [PubMed ID: 15111295]. https://doi.org/10.1080/00222895.1990.10735516.
-
15.
Schurr SA, Marshall AN, Resch JE, Saliba SA. Two-Dimensional Video Analysis Is Comparable to 3d Motion Capture in Lower Extremity Movement Assessment. Int J Sports Phys Ther. 2017;12(2):163-72. [PubMed ID: 28515970]. [PubMed Central ID: PMC5380858].
-
16.
Scholz JP. Reliability and validity of the WATSMART three-dimensional optoelectric motion analysis system. Phys Ther. 1989;69(8):679-89. [PubMed ID: 2748722]. https://doi.org/10.1093/ptj/69.8.679.
-
17.
Vander Linden DW, Carlson SJ, Hubbard RL. Reproducibility and accuracy of angle measurements obtained under static conditions with the Motion Analysis video system. Phys Ther. 1992;72(4):300-5. [PubMed ID: 1584861]. https://doi.org/10.1093/ptj/72.4.300.
-
18.
Winter DA. Biomechanics and Motor Control of Human Movement. 4th ed. USA: Wiley and Sons; 2009. https://doi.org/10.1002/9780470549148.
-
19.
Franjoine MR, Gunther JS, Taylor MJ. Pediatric balance scale: a modified version of the berg balance scale for the school-age child with mild to moderate motor impairment. Pediatr Phys Ther. 2003;15(2):114-28. [PubMed ID: 17057441]. https://doi.org/10.1097/01.PEP.0000068117.48023.18.
-
20.
Yi SH, Hwang JH, Kim SJ, Kwon JY. Validity of pediatric balance scales in children with spastic cerebral palsy. Neuropediatrics. 2012;43(6):307-13. [PubMed ID: 23011753]. https://doi.org/10.1055/s-0032-1327774.
-
21.
Shrout PE, Fleiss JL. Intraclass correlations: uses in assessing rater reliability. Psychol Bull. 1979;86(2):420-8. [PubMed ID: 18839484]. https://doi.org/10.1037//0033-2909.86.2.420.
-
22.
Domholdt E. Physical therapy research. Principles and applications. 1993.
-
23.
Haley SM, Fragala-Pinkham MA. Interpreting change scores of tests and measures used in physical therapy. Phys Ther. 2006;86(5):735-43. [PubMed ID: 16649896]. https://doi.org/10.1093/ptj/86.5.735.
-
24.
Akoglu H. User's guide to correlation coefficients. Turk J Emerg Med. 2018;18(3):91-3. [PubMed ID: 30191186]. [PubMed Central ID: PMC6107969]. https://doi.org/10.1016/j.tjem.2018.08.001.
-
25.
Underwood FB. Clinical Research and Data Analysis. In: Placzek JD, Boyce DA, editors. Orthopaedic Physical Therapy Secrets. 3rd ed. Amsterdam, Netherlands: Elsevier; 2017. p. 150-62. https://doi.org/10.1016/B978-0-323-28683-1.00020-5.
-
26.
Krabbe PFM. Validity. In: Krabbe PFM, editor. The Measurement of Health and Health Status. Amsterdam, Netherlands: Elsevier; 2017. p. 113-34. https://doi.org/10.1016/B978-0-12-801504-9.00007-6.
-
27.
Stokes EK. Validity. In: Stokes EK, editor. Rehabilitation Outcome Measures. Amsterdam, Netherlands: Elsevier; 2011. p. 35-46. https://doi.org/10.1016/B978-0-443-06915-4.00005-X.
-
28.
Zaki R. Validation of Instrument Measuring Continuous Variable in Medicine. In: Hokimoto T, editor. Advances in Statistical Methodologies and Their Application to Real Problems. London, United Kingdom: IntechOpen; 2017. https://doi.org/10.5772/66151.
-
29.
Elrahim RM, Embaby EA, Ali MF, Kamel RM. Inter-rater and intra-rater reliability of Kinovea software for measurement of shoulder range of motion. Bulletin of Faculty of Physical Therapy. 2016;21(2):80-7. https://doi.org/10.4103/1110-6611.196778.
-
30.
Nix S, Russell T, Vicenzino B, Smith M. Validity and reliability of hallux valgus angle measured on digital photographs. J Orthop Sports Phys Ther. 2012;42(7):642-8. [PubMed ID: 22282040]. https://doi.org/10.2519/jospt.2012.3841.
-
31.
Noor R, Olyaei G, Hadian MR, Talebian S, Bashir MS. A reliable and accurate system of joint position sense measurement. Biomed Res. 2018;29(12):2528-31. https://doi.org/10.4066/biomedicalresearch.29-18-410.
-
32.
Roghani T, Khalkhali Zavieh M, Rahimi A, Talebian S, Dehghan Manshadi F, Akbarzadeh Baghban A, et al. The Reliability of Standing Sagittal Measurements of Spinal Curvature and Range of Motion in Older Women With and Without Hyperkyphosis Using a Skin-Surface Device. J Manipulative Physiol Ther. 2017;40(9):685-91. [PubMed ID: 29229059]. https://doi.org/10.1016/j.jmpt.2017.07.008.
-
33.
Ashnagar Z, Hadian MR, Olyaei G, Talebian Moghadam S, Rezasoltani A, Saeedi H, et al. Reliability of digital photography for assessing lower extremity alignment in individuals with flatfeet and normal feet types. J Bodyw Mov Ther. 2017;21(3):704-10. [PubMed ID: 28750988]. https://doi.org/10.1016/j.jbmt.2016.12.006.
-
34.
Matamalas A, Bago J, D'Agata E, Pellise F. Reliability and validity study of measurements on digital photography to evaluate shoulder balance in idiopathic scoliosis. Scoliosis. 2014;9(1):23. [PubMed ID: 25520746]. [PubMed Central ID: PMC4269069]. https://doi.org/10.1186/s13013-014-0023-6.
-
35.
Koozekanani SH, Stockwell CW, McGhee RB, Firoozmand F. On the role of dynamic models in quantitative posturography. IEEE Trans Biomed Eng. 1980;27(10):605-9. [PubMed ID: 7439918]. https://doi.org/10.1109/TBME.1980.326583.
-
36.
Dutt-Mazumder A, Slobounov SM, Challis JH, Newell KM. Postural Stability Margins as a Function of Support Surface Slopes. PLoS One. 2016;11(10). e0164913. [PubMed ID: 27764158]. [PubMed Central ID: PMC5072559]. https://doi.org/10.1371/journal.pone.0164913.
-
37.
Cobb SC, James CR, Hjertstedt M, Kruk J. A digital photographic measurement method for quantifying foot posture: validity, reliability, and descriptive data. J Athl Train. 2011;46(1):20-30. [PubMed ID: 21214347]. [PubMed Central ID: PMC3017485]. https://doi.org/10.4085/1062-6050-46.1.20.