Abstract
Background:
Obsessive-compulsive disorder (OCD) is characterized by alterations in brain connectivity, particularly within the default mode network (DMN) and the salience network (SN). Investigating these connectivity differences can provide a deeper understanding of the neural mechanisms underlying OCD.Methods:
This cross-sectional study involved 58 patients diagnosed with OCD and 38 healthy control subjects, totaling 96 participants. Resting-state functional MRI (fMRI) data were acquired and analyzed using the CONN toolbox to examine functional connectivity within intrinsic resting-state networks. Graph theory metrics were applied to evaluate node connections and the overall network topology. Clinical symptoms were assessed using the Yale-Brown Obsessive-Compulsive Scale (Y-BOCS), and their correlations with connectivity patterns and graph-theory parameters were analyzed.Results:
The OCD patients and healthy controls were matched in terms of age, gender, marital status, socioeconomic status, and handedness. However, OCD patients had significantly worse general health, quality of life, and higher levels of depression and anxiety. Network analyses revealed altered whole-brain connectivity in OCD patients, particularly within the DMN and the frontoparietal network. The most significant between-group connectivity differences were observed between the posterior parietal cortex (PPC) and the precuneus. Disruptions in the DMN, specifically altered connectivity between the medial prefrontal cortex and posterior cingulate cortex, and changes in the SN involving the anterior insula and anterior cingulate cortex, were significantly correlated with the severity of OCD symptoms.Conclusions:
The findings suggest that OCD is associated with distinct alterations in DMN connectivity, which may play a critical role in the disorder's pathophysiology. These disruptions offer potential targets for therapeutic intervention. Further research is needed to explore these connectivity changes in larger cohorts and at various stages of OCD to better understand their clinical significance.Keywords
OCD Sertraline fMRI Functional Connectivity Graph-Theory Default Mode Network Salience Network Brain Mapping Brain Networks
1. Background
Obsessive-compulsive disorder (OCD) is a chronic mental health condition characterized by intrusive, unwanted thoughts (obsessions) and repetitive behaviors (compulsions) that can significantly disrupt daily functioning and reduce quality of life (1, 2). Affecting approximately 2 - 3% of the population, the precise brain mechanisms underlying OCD are not yet fully understood. However, advancements in brain imaging techniques, particularly functional magnetic resonance imaging (fMRI), have provided valuable insights into the neural circuits implicated in the disorder (2, 3). This study aims to contribute to the growing body of research by investigating functional connectivity within two key brain networks: The default mode network (DMN) and the salience network (SN) following treatment with sertraline, a commonly prescribed selective serotonin reuptake inhibitor (SSRI).
The DMN is primarily associated with self-referential thinking, mind-wandering, and the integration of internal and external information. The key nodes of the DMN include the medial prefrontal cortex (mPFC) and the posterior cingulate cortex (PCC) (4). Previous research has linked the DMN to various psychiatric disorders, including OCD, suggesting that disruptions in this network may contribute to the disorder's symptomatology (5-7). Specifically, abnormal DMN connectivity may play a role in the repetitive, intrusive thoughts characteristic of OCD.
The SN, in contrast, is involved in detecting and filtering salient stimuli and facilitating the switch between the DMN and the central executive network (CEN), which governs goal-directed behaviors. Key regions of the SN include the anterior insula and the dorsal anterior cingulate cortex (ACC). Research has shown alterations in the SN in OCD, suggesting that this network may contribute to the heightened focus on intrusive thoughts and compulsive actions that define the disorder (8, 9).
The mPFC and PCC, as central nodes of the DMN, are of particular interest in the study of OCD due to their roles in cognitive and emotional regulation (9). The mPFC is associated with decision-making, emotional regulation, and social cognition, while the PCC is involved in memory and the processing of autobiographical information (10, 11). Disruptions in the connectivity of these regions could underlie some of the core symptoms of OCD, such as the persistence of intrusive thoughts and difficulty in shifting attention away from them (12-15).
This study investigates how the functional connectivity of these networks changes following treatment with sertraline, with the aim of identifying neural mechanisms that may explain symptom reduction and therapeutic effects in OCD patients.
Sertraline, a commonly prescribed selective serotonin reuptake inhibitor (SSRI) for treating OCD, works by increasing serotonin levels to help regulate the disrupted serotonin system in the brain. Clinically, it reduces the intensity of obsessions and compulsions, leading to an enhanced quality of life for patients, although the improvement may take several weeks, and the effectiveness can vary among individuals. Often, dosage adjustments or additional therapeutic interventions, such as cognitive behavioral therapy (CBT), are required to achieve optimal outcomes. Sertraline not only modulates neurotransmitter levels but also impacts brain connectivity, as demonstrated by fMRI studies. These studies reveal that sertraline reduces hyperconnectivity in anxiety- and depression-related networks, including the DMN and amygdala circuits. The observed changes in connectivity are thought to be linked to its therapeutic effects, and are accompanied by alterations in synaptic plasticity, receptor sensitivity, and downstream signaling pathways (16-19).
This study employs graph theory, a powerful tool for analyzing the brain as a complex network of interconnected regions, to quantify the properties of brain networks such as efficiency, modularity, and centrality. Graph theory allows for the examination of the brain's connectivity by treating brain regions as "nodes" and the connections between them as "edges." Through this method, we can measure specific characteristics of the brain networks in patients with OCD that may be altered in the disorder. Previous research indicates that OCD patients often exhibit increased connectivity within the DMN and decreased connectivity between the DMN and other networks, such as the SN and Central Executive Network (CEN). These alterations may be responsible for the rigid and repetitive thought patterns that characterize OCD (20, 21).
To overcome limitations in previous research, such as the heterogeneity of medications used or small and non-adult samples, this study focused on a more homogeneous group of adults diagnosed with OCD who were consistently treated with sertraline. We compared the brain connectivity of 57 OCD patients with that of 64 healthy controls, with a particular focus on the mPFC and PCC, which are key regions within the DMN and SN. Using resting-state fMRI and graph theory metrics, we aimed to clarify how sertraline modulates these brain networks, potentially providing novel insights into the neural mechanisms underlying OCD and offering evidence to enhance the efficacy of SSRI-based treatments.
2. Objectives
This study advances our understanding of how OCD impacts brain connectivity, particularly in areas involved in self-referential thinking and emotional regulation, contributing to improved therapeutic strategies.
3. Methods
3.1. Participants
This study included a total of 96 participants, with 58 individuals diagnosed with OCD and 38 healthy controls. Participants were recruited through local clinics and public advertisements, and all provided written informed consent. The study protocol was approved by the Institutional Review Board (IRB).
3.2. Inclusion and Exclusion Criteria
- Obsessive-compulsive disorder group: Participants in the OCD group were required to have a primary diagnosis of OCD according to the DSM-5 criteria, be between the ages of 18 and 50, and be right-handed.
- Healthy controls: Similarly, healthy control participants were required to be right-handed, aged 18 to 50, with no current or previous psychiatric diagnoses as determined through the Structured Clinical Interview for DSM-5 (SCID).
Exclusion criteria for both groups included the presence of any neurological disorders, significant head injury, substance abuse or dependence within the past six months, the use of psychoactive medications that could affect fMRI outcomes, contraindications for MRI (such as metallic implants or claustrophobia), pregnancy or breastfeeding, and any significant medical conditions. In addition, participants with a history of major depressive disorder, bipolar disorder, psychotic disorders, or other anxiety disorders were excluded to maintain the specificity of the OCD diagnosis.
3.3. Medication History
The majority of participants (all but three) were drug-naive, meaning they had not been exposed to psychiatric medications prior to the study. The three non-naive participants had been on sertraline for at least six months before transitioning to the study protocol. These participants had their sertraline regimen adjusted under the supervision of a study psychiatrist. The sertraline treatment began with an initial dose of 12.5 mg once daily, which was gradually increased to 25 mg after 3 days and then to 50 mg after another 3 days. Based on patient tolerance, the dose was further adjusted to 75 - 100 mg once daily after 5 days and maintained for 7 - 8 weeks. Dosing adjustments were tailored to ensure both patient safety and optimal therapeutic outcomes, with participants being closely monitored throughout the study period.
3.4. Clinical Assessments
The Yale-Brown Obsessive-Compulsive Scale (Y-BOCS) is a clinician-administered tool measuring OCD severity across ten items, each rated on a 5-point scale, with total scores from 0 to 40. Higher scores indicate more severe symptoms. The Dimensional Obsessive-Compulsive Scale (DOCS) is a self-report questionnaire assessing OCD severity across four dimensions—contamination, harm responsibility, unacceptable thoughts, and symmetry/completeness—each rated on a 0 - 20 scale, with total scores from 0 to 80. Higher scores indicate greater severity. The Beck Depression Inventory (BDI) and Beck Anxiety Inventory (BAI) are 21-item self-report tools measuring depression and anxiety severity, respectively, each rated on a 4-point scale, with scores ranging from 0 to 63, where higher scores indicate more severe symptoms.
3.5. Functional Magnetic Resonance Imaging Data Acquisition
All subjects underwent 3T fMRI (Siemens Magnetom) at the National Brain Mapping Library using a 64-channel head coil. Data acquisition involved single-shot gradient-recalled EPI with 78 slices (TR = 1098 ms, TE = 30.0 ms, matrix size 128 × 128, FOV 192 × 192 mm, slice thickness 3.0 mm), and a high-resolution T1 anatomical sequence using a 3D MPRAGE protocol (TR = 2060.0 ms, TE = 3.63 ms, voxel size 0.5 × 0.5 × 0.8 mm). Resting-state fMRI runs, each 7:25 minutes long, were conducted with participants viewing a black screen with a central cross. Data processing, using Conn Toolbox v22, included realignment, slice timing correction, outlier detection, segmentation, normalization to MNI space, and smoothing. Denoising involved regression of confounding effects and bandpass filtering (0.008 - 0.09 Hz), with an effective BOLD signal degrees of freedom post-denoising around 66.9. Analyses included seed-based connectivity maps (SBC) and ROI-to-ROI connectivity matrices (RRC) for 165 regions of interest (ROIs), quantified via Fisher-transformed bivariate correlation coefficients from a weighted general linear model. Graph-theory parameters—such as node degree, efficiency, centrality, node eccentricity, path length, and clustering coefficient—were assessed to evaluate network connectivity and efficiency.
3.6. Statistical Analysis
Statistical analyses were conducted to compare functional connectivity and graph theory metrics between OCD patients and healthy controls. Group comparisons were performed using two-sample t-tests, and partial correlation analyses (controlling for age, gender, and IQ) were conducted to examine potential correlations between these parameters, utilizing SPSS v. 27 and the Python package (v. 3.12: Parcorr). Additionally, for second-level functional connectivity analysis, we employed the General Linear Model (GLM) within the Conn toolbox. This approach enabled us to analyze group-level differences and correlations by constructing a design matrix that incorporated experimental conditions, subject groups, and covariates like age and symptom severity. By applying the GLM, we compared connectivity patterns between patient and control groups and explored relationships with continuous variables. The resulting statistical maps, refined through multiple comparison corrections, identified brain regions with significant connectivity differences, providing insights into the neural mechanisms under investigation.
4. Results
The baseline characteristics of OCD patients and healthy controls, summarized in Table 1, showed no significant differences in age (OCD: Mean 28.78 years, controls: Mean 26.76 years, P = 0.21), gender distribution (OCD: 21 men, 37 women; controls: 20 men, 18 women, P = 0.11), marital status (OCD: 14 married; controls: 8 married, P = 0.72), socioeconomic status, or handedness (OCD: 53 right-handed, 5 left-handed; controls: 34 right-handed, 4 left-handed, P = 0.75). IQ (OCD: Mean 109.91; controls: Mean 113.18, P = 0.07) and education years (OCD: Mean 14.93; controls: Mean 15.82, P = 0.08) approached significance. However, the OCD group had significantly worse general health (mean 28.34 vs. 10.08, P < 0.001), lower quality of life (mean 63.66 vs. 87.01, P < 0.001), and higher depression (mean 20.50 vs. 2.16, P < 0.001) and anxiety scores (mean 17.88 vs. 4.29, P < 0.001). Overall, while the groups were similar in many baseline characteristics, the OCD group exhibited significantly poorer health, quality of life, and mental health outcomes, with trends towards significance in IQ and education level, warranting further investigation.
Demographic and Baseline Characteristics of Study Groups
Variables | Descriptive Statistics | Comparisons | ||||||||
---|---|---|---|---|---|---|---|---|---|---|
Patients | Healthy Controls | |||||||||
N | Mean (Std. E) | Std. D | Range / CI | N | Mean (Std. E) | Std. D | Range / CI | Sig. | Ղ2 | |
Age | 58 | 28.78 (1.05) | 8.00 | 18 - 49 | 38 | 26.76 (1.06) | 6.57 | 20 - 44 | 0.21 | 0.26 |
Gender | 1.3 (0.06) | 0.48 | (1.24 - 1.48) | 1.53 (0.08) | 0.50 | (1.37 - 1.68) | 0.11 | - | ||
Men | 21 | 20 | ||||||||
Women | 37 | 18 | ||||||||
Marital status | 1.24 (0.05) | 0.43 | (1.14 - 1.34) | 1.21 (0.06) | 0.41 | (1.08 - 1.37) | 0.72 | - | ||
Married | 14 | 8 | ||||||||
Not-married | 44 | 20 | ||||||||
IQ | 58 | 109.91 (0.97) | 7.45 | 92 - 133 | 38 | 113.18 (1.62) | 9.99 | 98 - 138 | 0.07 | - 0.38 |
Education level (y) | 58 | 14.93 (0.30) | 2.28 | 10 - 22 | 38 | 15.82 (0.42) | 2.63 | 6 - 20 | 0.08 | - 0.36 |
Socioeconomic status | 1.93 (0.04) | 0.36 | (1.84 - 2.03) | 1.92 (0.6) | 0.42 | (1.79 - 2.05) | 0.81 | - | ||
Low | 6 | 5 | ||||||||
Middle | 50 | 31 | ||||||||
High | 2 | 2 | ||||||||
Handedness | 1.08 (0.03) | 0.28 | (1.01 - 1.17) | 1.10 (0.05) | 0.31 | (1.02 - 1.21) | 0.75 | - | ||
Right | 53 | 34 | ||||||||
Left | 5 | 4 | ||||||||
General health state | 58 | 28.34 (2.05) | 15.62 | 8 - 78 | 38 | 10.08 (8.95 - 11.10) | 3.65 | 4 - 20 | < 0.001 | 1.47 |
Quality of life | 58 | 63.66 (2.20) | 16.66 | (28.53 - 94.86) | 38 | 87 - 01 (1.17) | 7.13 | (71.94 - 97.78) | < 0.001 | - 1.61 |
Depression | 58 | 20.50 (1.56) | 11.93 | 0 - 55 | 38 | 2.16 (0.17) | 1.10 | 0 - 5 | < 0.001 | 1.96 |
Anxiety | 58 | 17.88 (1.45) | 11.04 | 3 - 40 | 38 | 4.29 (0.24) | 1.50 | 0 - 7 | < 0.001 | 1.57 |
4.1. Network-Specific Alterations in Functional Connectivity in Obsessive-Compulsive Disorder
4.1.1. Whole Brain Connectivity
The first row of ring plots in Figure 1 illustrates whole-brain connectivity patterns for both the OCD and healthy control (HC) groups, visualizing functional connections between various brain regions. In the OCD group, a distinct connectivity pattern emerges, differing notably from the HC group, suggesting altered brain functional architecture in OCD. The Diff plot highlights statistically significant connections that differentiate the two groups. Hierarchical clustering analysis identified the most significant connection within the Default Mode Network in the OCD group, specifically between the posterior parietal cortex (PPC) and the precuneus, with an F-statistic of 2458.31 and a highly significant p-FDR value (< 0.0001). When manually grouping connections by networks, the most significant connection was found between the left and right putamen, with an F-statistic of 1092.39 and an equally significant p-FDR value (< 0.0001). These findings support the hypothesis that alterations in subcortical structures, such as the putamen, play a crucial role in the pathophysiology of OCD.
Whole-brain and network specific connectivity patterns in OCD and healthy controls. Functional connectivity patterns across the brain in OCD and healthy control groups, as determined by General Linear Model (GLM) analysis. The GLM was employed to assess differences in connectivity by modeling the relationship between brain activity and group membership (OCD vs. healthy controls), while controlling for potential confounding variables such as age and gender. This approach allows for the identification of statistically significant differences in connectivity patterns across the entire brain as well as within specific networks. The first row displays whole-brain connectivity patterns segmented by major brain networks: Default Mode Network (DMN), salience network (SN), frontoparietal network, visual network, language network, cerebellar network, and dorsal attention network. The second row illustrates connectivity specifically within the DMN, comparing both groups and highlighting differential connections. The third row depicts connectivity within the salience network, and the fourth row shows connections within the frontoparietal network, with a focus on group-specific differences. Brain regions in the chord plots are ordered based on network-based (manual) approach, providing an overview of whole-brain functional connectivity patterns and sparsed for more clarification standard setting for cluster-based inference, depicted as spatial pairwise connections (connection threshold: P < 0.05, FDR corrected and two-sided; cluster threshold: P < 0.05 and FDR corrected).
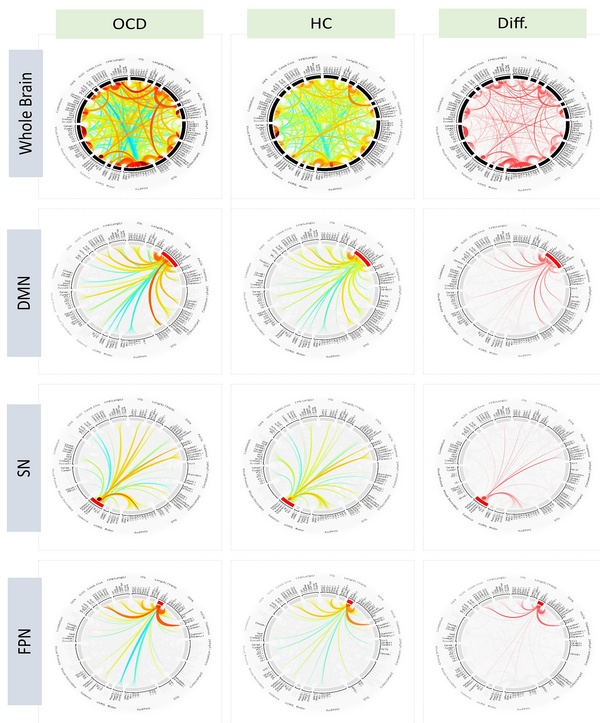
4.1.2. Default Mode Network
The second row of Figure 1 highlights the DMN, comprising regions such as the mPFC, PCC, and angular gyrus, which are crucial for self-referential thought and mind-wandering. In the OCD group, DMN connectivity significantly differs from that of healthy controls. The Diff plot reveals notable functional alterations, with the most significant connection observed between the left and right posterior middle temporal gyrus (pMTG), showing an F-statistic of 398.82 and a highly significant p-FDR value (< 0.0001). This marked connectivity difference between bilateral pMTG regions in OCD patients suggests disruptions in the DMN's role in internal thought processes, potentially contributing to the persistent intrusive thoughts characteristic of OCD.
4.1.3. Salience Network
The third row of ring plots in Figure 1 highlights the SN, including key regions such as the anterior cingulate cortex (ACC) and anterior insula, which are essential for detecting salient stimuli and coordinating appropriate behavioral responses. In OCD patients, the SN displays distinct connectivity patterns compared to healthy controls. The Diff plot reveals significant alterations, with the most pronounced connections observed between the right and left putamen (F-statistic 1092.39, p-FDR < 0.0001), indicating altered connectivity related to habit formation and motor control. Additionally, a significant connection between the right frontal operculum and right insular cortex (F-statistic 219.48, p-FDR < 0.0001) suggests disruptions in salience detection and emotional processing, which could contribute to OCD's heightened threat sensitivity and compulsive behaviors.
4.1.4. Frontoparietal Network (FPN)
The fourth row of ring plots in Figure 1 illustrates the FPN, which includes critical regions such as the dorsolateral prefrontal cortex and the posterior parietal cortex. These areas are essential for executive functions like working memory, decision-making, and cognitive control. Notably, the connectivity patterns within this network differ between patients with OCD and healthy controls. The most significant connection, based on between-group contrasts, was identified between the right frontal pole and the right middle frontal gyrus, yielding an F-statistic of 664.84 and a highly significant p-FDR value (< 0.0001). This finding highlights altered connectivity in regions critical for executive functions in individuals with OCD, suggesting that these disruptions may contribute to the impaired cognitive control observed in the disorder.
4.2. Correlations Between Obsessive-Compulsive Severity and Brain Network Parameters
In the partial correlation analysis (Figure 2), controlling for age, significant associations were identified between Y-BOCS scores and various brain network parameters in patients with OCD. Specifically, within the SN, the severity of obsessive-compulsive symptoms was significantly correlated with the eccentricity of the left anterior insula (r = 0.33, P = 0.018), the left supramarginal gyrus (r = 0.31, P = 0.025), and the anterior cingulate cortex (r = 0.28, P = 0.041). A marginally significant association was also observed for the left rostral prefrontal cortex (r = 0.27, P = 0.049). In the FPN, significant correlations were found with the eccentricity (r = 0.30, P = 0.031) and local efficiency (r = 0.28, P = 0.041) of the right posterior parietal cortex, as well as the clustering coefficient of the left posterior parietal cortex (r = 0.28, P = 0.044). Additionally, in the DMN, the eccentricity of the right lateral parietal cortex was significantly associated with OCD severity (r = 0.36, P = 0.009). These findings highlight the importance of specific brain regions and their network characteristics in relation to the severity of OCD symptoms.
Partial correlation analyses of graph theory measures and OCD severity. Results of the partial correlation analysis, controlled for age, assessing the relationship between graph theory measures derived from functional connections and OCD severity, as indicated by the Yellow-Brown Obsessive Compulsive Scale (Y-BOCS) total score. This multi-panel figure illustrates correlations within nodes of the default mode network (DMN), salience network (SN), and frontoparietal network (FPN), focusing on eccentricity (EC), local efficiency (LE), and clustering coefficient (COL), with significance set at P < 0.05.
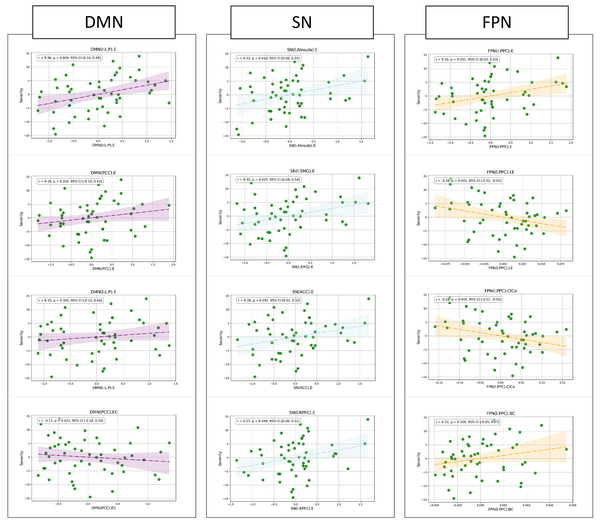
5. Discussion
This study explored the functional connectivity within the DMN and SN in patients with OCD compared to healthy controls using resting-state fMRI. We identified significant graph-theory-driven differences, particularly within the DMN, and key nodes of the SN, such as the anterior insula, anterior cingulate cortex, and frontoparietal regions. These connectivity alterations were significantly associated with OCD severity, suggesting that disruptions in these brain networks contribute to the disorder's symptoms.
Selective Serotonin Reuptake Inhibitors (SSRIs), a common treatment for OCD, influence neuronal pathways and functional connectivity by increasing serotonin (5-HT) availability in the synaptic cleft. Serotonin plays a crucial role in mood regulation, anxiety control, and cognitive functions by modulating synaptic plasticity, which is vital for learning and memory processes (22, 23). By blocking serotonin reuptake into presynaptic neurons, SSRIs enhance serotonergic signaling, triggering a cascade of intracellular events that affect both short-term synaptic function and long-term neuronal adaptations (23, 24). SSRIs primarily influence synaptic plasticity by affecting long-term potentiation (LTP), a process that strengthens synaptic connections, essential for learning and memory. Serotonin receptors, particularly 5-HT1A, modulate LTP by regulating neurotransmitter release (e.g., glutamate) and calcium influx, which can enhance or inhibit LTP depending on the brain region. Chronic SSRI treatment induces gene expression changes and protein synthesis, supporting synaptic growth and stability, particularly in the hippocampus, a region crucial for memory and emotional regulation (25-27). SSRIs also boost brain-derived neurotrophic factor (BDNF) expression, a protein essential for synaptic plasticity and neurogenesis in the hippocampus, further enhancing mood and cognitive function, contributing to their antidepressant effects (25-27).
The functional connectivity differences between individuals with OCD and healthy controls are particularly evident within the DMN, a network known for its role in self-referential thought and internal mental processes (28-30). The DMN comprises several key regions: The medial prefrontal cortex (mPFC), the PCC, and the angular gyrus. Research employing resting-state fMRI has revealed significant disruptions in the connectivity of these DMN regions in OCD patients (31-33). A prominent study by Wang et al. identified decreased functional connectivity within the DMN in individuals with OCD (34). Specifically, the connectivity between the mPFC and PCC was found to be diminished in OCD patients compared to healthy controls. This reduced connectivity within the DMN correlates with symptom severity and the presence of intrusive thoughts and compulsive behaviors (35). For instance, the altered functional connectivity between the mPFC and PCC has been associated with increased self-referential processing and heightened self-focused attention, which may contribute to the persistence of obsessive thoughts.
Further research by Gürsel et al. (15) using seed-based connectivity analyses showed that the connectivity between the DMN and the fronto-striatal circuits, which are involved in cognitive control and reward processing, is disrupted in OCD. This disruption suggests that the DMN's interactions with other cognitive networks are altered in OCD, potentially leading to difficulties in regulating obsessive-compulsive symptoms (9, 35, 36). In OCD patients, this plot demonstrates significant reductions in connectivity strength between key DMN regions and altered connectivity patterns between the DMN and other brain networks. For example, increased connectivity between the mPFC and the orbitofrontal cortex has been observed, which may reflect a compensatory mechanism or a maladaptive response to intrusive thoughts. These alterations in DMN connectivity underscore the critical role of the DMN in maintaining cognitive and emotional regulation, suggesting that targeting the DMN through interventions like CBT or neuromodulation techniques such as transcranial magnetic stimulation (TMS) could be beneficial (34, 37). By focusing on these connectivity disruptions, therapeutic strategies may be able to more effectively address the underlying neural mechanisms contributing to OCD symptoms and improve treatment outcomes.
Notably, the eccentricity of the nodes within the salience network (38-40), particularly the anterior insula and the anterior cingulate cortex, has demonstrated strong correlations with obsessive-compulsive disorder symptoms, highlighting their critical role in the disorder's pathophysiology. The salience network is pivotal in detecting and prioritizing significant stimuli and orchestrating appropriate behavioral responses. The anterior insula, a key component of this network, is essential for interoceptive awareness, which involves the integration of sensory, emotional, and cognitive information to assess the relevance of internal and external stimuli. Altered connectivity and activation patterns in the anterior insula have been linked to increased sensitivity to perceived threats and maladaptive responses, which are hallmark features of OCD (41, 42). Furthermore, other researchers in this field have identified abnormalities in the cortico-striato-thalamo-cortical (CSTC) pathways, which overlap with nodes of the salience network, partially supporting these previous findings. The involvement of these overlapping areas is crucial in contextualizing the effects of SSRIs, as these medications are known to modulate connectivity within these networks, thereby mitigating OCD symptoms (38, 43).
The ACC, another integral component of the salience network, is involved in error detection, conflict monitoring, and cognitive control. Dysfunction in the ACC has been linked to persistent and intrusive thoughts characteristic of OCD, potentially due to its impaired ability to signal the need for behavioral adjustments or to inhibit compulsive behaviors. Studies have shown that patients with OCD exhibit increased ACC activation during tasks requiring error detection and conflict resolution, suggesting that this overactivity may contribute to the persistence of obsessive thoughts and compulsive actions (44-46).
5.1. Conclusions
This study identified significant alterations in functional connectivity in key brain networks of individuals with OCD compared to healthy controls. Disruptions were found in the DMN, particularly between the left and right posterior middle temporal gyrus, suggesting altered self-referential processing in OCD. In the SN, significant changes were noted between the right and left putamen and between the right frontal operculum and right insular cortex, which may relate to the heightened sensitivity and compulsive behaviors in OCD. The FPN showed disrupted connectivity between the right frontal pole and right middle frontal gyrus, implicating cognitive control deficits.
Acknowledgements
References
-
1.
Stein DJ, Costa DLC, Lochner C, Miguel EC, Reddy YCJ, Shavitt RG, et al. Obsessive-compulsive disorder. Nat Rev Dis Primers. 2019;5(1):52. [PubMed ID: 31371720]. [PubMed Central ID: PMC7370844]. https://doi.org/10.1038/s41572-019-0102-3.
-
2.
Singh A, Anjankar VP, Sapkale B. Obsessive-Compulsive Disorder (OCD): A Comprehensive Review of Diagnosis, Comorbidities, and Treatment Approaches. Cureus. 2023;15(11). e48960. [PubMed ID: 38111433]. [PubMed Central ID: PMC10726089]. https://doi.org/10.7759/cureus.48960.
-
3.
Ferreira S, Pego JM, Morgado P. A Systematic Review of Behavioral, Physiological, and Neurobiological Cognitive Regulation Alterations in Obsessive-Compulsive Disorder. Brain Sci. 2020;10(11). [PubMed ID: 33138023]. [PubMed Central ID: PMC7692269]. https://doi.org/10.3390/brainsci10110797.
-
4.
Alves PN, Foulon C, Karolis V, Bzdok D, Margulies DS, Volle E, et al. An improved neuroanatomical model of the default-mode network reconciles previous neuroimaging and neuropathological findings. Communications Biology. 2019;2(1):370. https://doi.org/10.1038/s42003-019-0611-3.
-
5.
Doucet GE, Janiri D, Howard R, O'Brien M, Andrews-Hanna JR, Frangou S. Transdiagnostic and disease-specific abnormalities in the default-mode network hubs in psychiatric disorders: A meta-analysis of resting-state functional imaging studies. Eur Psychiatry. 2020;63(1). e57. [PubMed ID: 32466812]. [PubMed Central ID: PMC7355168]. https://doi.org/10.1192/j.eurpsy.2020.57.
-
6.
Choi KM, Kim JY, Kim YW, Han JW, Im CH, Lee SH. Comparative analysis of default mode networks in major psychiatric disorders using resting-state EEG. Sci Rep. 2021;11(1):22007. [PubMed ID: 34759276]. [PubMed Central ID: PMC8580995]. https://doi.org/10.1038/s41598-021-00975-3.
-
7.
Chou T, Deckersbach T, Dougherty DD, Hooley JM. The default mode network and rumination in individuals at risk for depression. Soc Cogn Affect Neurosci. 2023;18(1). [PubMed ID: 37261927]. [PubMed Central ID: PMC10634292]. https://doi.org/10.1093/scan/nsad032.
-
8.
Bijanki KR, Pathak YJ, Najera RA, Storch EA, Goodman WK, Simpson HB, et al. Defining functional brain networks underlying obsessive-compulsive disorder (OCD) using treatment-induced neuroimaging changes: a systematic review of the literature. J Neurol Neurosurg Psychiatry. 2021;92(7):776-86. [PubMed ID: 33906936]. [PubMed Central ID: PMC8223624]. https://doi.org/10.1136/jnnp-2020-324478.
-
9.
Luo L, Li Q, You W, Wang Y, Tang W, Li B, et al. Altered brain functional network dynamics in obsessive-compulsive disorder. Hum Brain Mapp. 2021;42(7):2061-76. [PubMed ID: 33522660]. [PubMed Central ID: PMC8046074]. https://doi.org/10.1002/hbm.25345.
-
10.
Xu P, Chen A, Li Y, Xing X, Lu H. Medial prefrontal cortex in neurological diseases. Physiol Genomics. 2019;51(9):432-42. [PubMed ID: 31373533]. [PubMed Central ID: PMC6766703]. https://doi.org/10.1152/physiolgenomics.00006.2019.
-
11.
Euston DR, Gruber AJ, McNaughton BL. The role of medial prefrontal cortex in memory and decision making. Neuron. 2012;76(6):1057-70. [PubMed ID: 23259943]. [PubMed Central ID: PMC3562704]. https://doi.org/10.1016/j.neuron.2012.12.002.
-
12.
van Noordt SJ, Segalowitz SJ. Performance monitoring and the medial prefrontal cortex: a review of individual differences and context effects as a window on self-regulation. Front Hum Neurosci. 2012;6:197. [PubMed ID: 22798949]. [PubMed Central ID: PMC3394443]. https://doi.org/10.3389/fnhum.2012.00197.
-
13.
Foster BL, Koslov SR, Aponik-Gremillion L, Monko ME, Hayden BY, Heilbronner SR. A tripartite view of the posterior cingulate cortex. Nat Rev Neurosci. 2023;24(3):173-89. [PubMed ID: 36456807]. [PubMed Central ID: PMC10041987]. https://doi.org/10.1038/s41583-022-00661-x.
-
14.
Reess TJ, Rus OG, Schmidt R, de Reus MA, Zaudig M, Wagner G, et al. Connectomics-based structural network alterations in obsessive-compulsive disorder. Transl Psychiatry. 2016;6(9). e882. [PubMed ID: 27598966]. [PubMed Central ID: PMC5048203]. https://doi.org/10.1038/tp.2016.163.
-
15.
Gürsel DA, Avram M, Sorg C, Brandl F, Koch K. Frontoparietal areas link impairments of large-scale intrinsic brain networks with aberrant fronto-striatal interactions in OCD: a meta-analysis of resting-state functional connectivity. Neurosci Biobehav Rev. 2018;87:151-60. [PubMed ID: 29410103]. https://doi.org/10.1016/j.neubiorev.2018.01.016.
-
16.
Chu SH, Parhi KK, Westlund Schreiner M, Lenglet C, Mueller BA, Klimes-Dougan B, et al. Effect of SSRIs on Resting-State Functional Brain Networks in Adolescents with Major Depressive Disorder. J Clin Med. 2021;10(19). [PubMed ID: 34640340]. [PubMed Central ID: PMC8509847]. https://doi.org/10.3390/jcm10194322.
-
17.
Anand A, Li Y, Wang Y, Gardner K, Lowe MJ. Reciprocal effects of antidepressant treatment on activity and connectivity of the mood regulating circuit: an FMRI study. J Neuropsychiatry Clin Neurosci. 2007;19(3):274-82. [PubMed ID: 17827412]. [PubMed Central ID: PMC3465666]. https://doi.org/10.1176/jnp.2007.19.3.274.
-
18.
Zhao K, Xie H, Fonzo GA, Tong X, Carlisle N, Chidharom M, et al. Individualized fMRI connectivity defines signatures of antidepressant and placebo responses in major depression. Mol Psychiatry. 2023;28(6):2490-9. [PubMed ID: 36732585]. https://doi.org/10.1038/s41380-023-01958-8.
-
19.
Kotoula V, Evans JW, Punturieri CE, Zarate CJ. Review: The use of functional magnetic resonance imaging (fMRI) in clinical trials and experimental research studies for depression. Front Neuroimaging. 2023;2:1110258. [PubMed ID: 37554642]. [PubMed Central ID: PMC10406217]. https://doi.org/10.3389/fnimg.2023.1110258.
-
20.
Armstrong CC, Moody TD, Feusner JD, McCracken JT, Chang S, Levitt JG, et al. Graph-theoretical analysis of resting-state fMRI in pediatric obsessive-compulsive disorder. J Affect Disord. 2016;193:175-84. [PubMed ID: 26773910]. [PubMed Central ID: PMC5767329]. https://doi.org/10.1016/j.jad.2015.12.071.
-
21.
Kim SG, Jung WH, Kim SN, Jang JH, Kwon JS. Disparity between dorsal and ventral networks in patients with obsessive-compulsive disorder: evidence revealed by graph theoretical analysis based on cortical thickness from MRI. Front Hum Neurosci. 2013;7:302. [PubMed ID: 23840184]. [PubMed Central ID: PMC3699763]. https://doi.org/10.3389/fnhum.2013.00302.
-
22.
Taler M, Miron O, Gil-Ad I, Weizman A. Neuroprotective and procognitive effects of sertraline: in vitro and in vivo studies. Neurosci Lett. 2013;550:93-7. [PubMed ID: 23827216]. https://doi.org/10.1016/j.neulet.2013.06.033.
-
23.
Normann C, Frase S, Haug V, von Wolff G, Clark K, Munzer P, et al. Antidepressants Rescue Stress-Induced Disruption of Synaptic Plasticity via Serotonin Transporter-Independent Inhibition of L-Type Calcium Channels. Biol Psychiatry. 2018;84(1):55-64. [PubMed ID: 29174591]. https://doi.org/10.1016/j.biopsych.2017.10.008.
-
24.
Izumi Y, Reiersen AM, Lenze EJ, Mennerick SJ, Zorumski CF. Sertraline modulates hippocampal plasticity and learning via sigma 1 receptors, cellular stress and neurosteroids. bioRxiv. 2024. [PubMed ID: 38328198]. [PubMed Central ID: PMC10849579]. https://doi.org/10.1101/2024.01.23.576911.
-
25.
Matrisciano F, Bonaccorso S, Ricciardi A, Scaccianoce S, Panaccione I, Wang L, et al. Changes in BDNF serum levels in patients with major depression disorder (MDD) after 6 months treatment with sertraline, escitalopram, or venlafaxine. J Psychiatr Res. 2009;43(3):247-54. [PubMed ID: 18511076]. [PubMed Central ID: PMC3744240]. https://doi.org/10.1016/j.jpsychires.2008.03.014.
-
26.
Brunoni AR, Machado-Vieira R, Zarate CJ, Vieira EL, Vanderhasselt MA, Nitsche MA, et al. BDNF plasma levels after antidepressant treatment with sertraline and transcranial direct current stimulation: results from a factorial, randomized, sham-controlled trial. Eur Neuropsychopharmacol. 2014;24(7):1144-51. [PubMed ID: 24702987]. [PubMed Central ID: PMC4081041]. https://doi.org/10.1016/j.euroneuro.2014.03.006.
-
27.
Amidfar M, Réus GZ, Quevedo J, Kim Y, Arbabi M. Effect of co-administration of memantine and sertraline on the antidepressant-like activity and brain-derived neurotrophic factor (BDNF) levels in the rat brain. Brain Research Bulletin. 2017;128:29-33. https://doi.org/10.1016/j.brainresbull.2016.11.003.
-
28.
Mohan A, Roberto AJ, Mohan A, Lorenzo A, Jones K, Carney MJ, et al. The Significance of the Default Mode Network (DMN) in Neurological and Neuropsychiatric Disorders: A Review. Yale J Biol Med. 2016;89(1):49-57. [PubMed ID: 27505016]. [PubMed Central ID: PMC4797836].
-
29.
Mak LE, Minuzzi L, MacQueen G, Hall G, Kennedy SH, Milev R. The Default Mode Network in Healthy Individuals: A Systematic Review and Meta-Analysis. Brain Connect. 2017;7(1):25-33. [PubMed ID: 27917679]. https://doi.org/10.1089/brain.2016.0438.
-
30.
Menon V. 20 years of the default mode network: A review and synthesis. Neuron. 2023;111(16):2469-87. [PubMed ID: 37167968]. [PubMed Central ID: PMC10524518]. https://doi.org/10.1016/j.neuron.2023.04.023.
-
31.
Goncalves OF, Soares JM, Carvalho S, Leite J, Ganho-Avila A, Fernandes-Goncalves A, et al. Patterns of Default Mode Network Deactivation in Obsessive Compulsive Disorder. Sci Rep. 2017;7:44468. [PubMed ID: 28287615]. [PubMed Central ID: PMC5347382]. https://doi.org/10.1038/srep44468.
-
32.
Stern ER, Fitzgerald KD, Welsh RC, Abelson JL, Taylor SF. Resting-state functional connectivity between fronto-parietal and default mode networks in obsessive-compulsive disorder. PLoS One. 2012;7(5). e36356. [PubMed ID: 22570705]. [PubMed Central ID: PMC3343054]. https://doi.org/10.1371/journal.pone.0036356.
-
33.
Geffen T, Smallwood J, Finke C, Olbrich S, Sjoerds Z, Schlagenhauf F. Functional connectivity alterations between default mode network and occipital cortex in patients with obsessive-compulsive disorder (OCD). Neuroimage Clin. 2022;33:102915. [PubMed ID: 34933233]. [PubMed Central ID: PMC8688720]. https://doi.org/10.1016/j.nicl.2021.102915.
-
34.
Wang YM, Cai XL, Zhou HY, Zhang RT, Zhang YJ, Wang YY, et al. Altered default mode network functional connectivity in individuals with co-occurrence of schizotypy and obsessive-compulsive traits. Psychiatry Res Neuroimaging. 2020;305:111170. [PubMed ID: 32836136]. https://doi.org/10.1016/j.pscychresns.2020.111170.
-
35.
Peng ZW, Xu T, He QH, Shi CZ, Wei Z, Miao GD, et al. Default network connectivity as a vulnerability marker for obsessive compulsive disorder. Psychol Med. 2014;44(7):1475-84. [PubMed ID: 24001350]. https://doi.org/10.1017/S0033291713002250.
-
36.
Cui G, Ou Y, Chen Y, Lv D, Jia C, Zhong Z, et al. Altered Global Brain Functional Connectivity in Drug-Naive Patients With Obsessive-Compulsive Disorder. Front Psychiatry. 2020;11:98. [PubMed ID: 32194450]. [PubMed Central ID: PMC7062961]. https://doi.org/10.3389/fpsyt.2020.00098.
-
37.
Tomiyama H, Murayama K, Nemoto K, Hasuzawa S, Mizobe T, Kato K, et al. Alterations of default mode and cingulo-opercular salience network and frontostriatal circuit: A candidate endophenotype of obsessive-compulsive disorder. Prog Neuropsychopharmacol Biol Psychiatry. 2022;116:110516. [PubMed ID: 35108587]. https://doi.org/10.1016/j.pnpbp.2022.110516.
-
38.
Peters SK, Dunlop K, Downar J. Cortico-Striatal-Thalamic Loop Circuits of the Salience Network: A Central Pathway in Psychiatric Disease and Treatment. Front Syst Neurosci. 2016;10:104. [PubMed ID: 28082874]. [PubMed Central ID: PMC5187454]. https://doi.org/10.3389/fnsys.2016.00104.
-
39.
Shao J, Meng C, Tahmasian M, Brandl F, Yang Q, Luo G, et al. Common and distinct changes of default mode and salience network in schizophrenia and major depression. Brain Imaging Behav. 2018;12(6):1708-19. [PubMed ID: 29460166]. https://doi.org/10.1007/s11682-018-9838-8.
-
40.
Schimmelpfennig J, Topczewski J, Zajkowski W, Jankowiak-Siuda K. The role of the salience network in cognitive and affective deficits. Front Hum Neurosci. 2023;17:1133367. [PubMed ID: 37020493]. [PubMed Central ID: PMC10067884]. https://doi.org/10.3389/fnhum.2023.1133367.
-
41.
McCutcheon RA, Nour MM, Dahoun T, Jauhar S, Pepper F, Expert P, et al. Mesolimbic Dopamine Function Is Related to Salience Network Connectivity: An Integrative Positron Emission Tomography and Magnetic Resonance Study. Biol Psychiatry. 2019;85(5):368-78. [PubMed ID: 30389131]. [PubMed Central ID: PMC6360933]. https://doi.org/10.1016/j.biopsych.2018.09.010.
-
42.
Fan J, Zhong M, Gan J, Liu W, Niu C, Liao H, et al. Altered connectivity within and between the default mode, central executive, and salience networks in obsessive-compulsive disorder. J Affect Disord. 2017;223:106-14. [PubMed ID: 28743059]. https://doi.org/10.1016/j.jad.2017.07.041.
-
43.
Liu J, Cao L, Li H, Gao Y, Bu X, Liang K, et al. Abnormal resting-state functional connectivity in patients with obsessive-compulsive disorder: A systematic review and meta-analysis. Neurosci Biobehav Rev. 2022;135:104574. [PubMed ID: 35151769]. https://doi.org/10.1016/j.neubiorev.2022.104574.
-
44.
McGovern RA, Sheth SA. Role of the dorsal anterior cingulate cortex in obsessive-compulsive disorder: converging evidence from cognitive neuroscience and psychiatric neurosurgery. J Neurosurg. 2017;126(1):132-47. [PubMed ID: 27035167]. https://doi.org/10.3171/2016.1.JNS15601.
-
45.
Kosova E, Pajuelo D, Gregus D, Brunovsky M, Stopkova P, Fajnerova I, et al. Glutamatergic abnormalities in the pregenual anterior cingulate cortex in obsessive-compulsive disorder using magnetic resonance spectroscopy: A controlled study. Psychiatry Res Neuroimaging. 2023;335:111721. [PubMed ID: 37832259]. https://doi.org/10.1016/j.pscychresns.2023.111721.
-
46.
Meek BP, Fotros A, Abo Aoun M, Modirrousta M. Improvements in error-monitoring and symptoms following low-frequency rTMS of dorsal anterior cingulate cortex in obsessive compulsive disorder; a randomized, sham-controlled study. Brain Cogn. 2021;154:105809. [PubMed ID: 34619574]. https://doi.org/10.1016/j.bandc.2021.105809.