Abstract
Background:
Ischemic brain injury is among the most common causes of death and disability in humans worldwide. Recent findings suggest that neural precursors in the adult mammalian brain can be a therapeutic target in ischemic brain injuries. Curcumin stimulates neurogenesis and reduces oxidative stress in the brain.Objectives:
The present study compared the neuroprotective effects of different concentrations of curcumin in various regions of the brain in a rat model of transient global ischemia (TGI).Materials and Methods:
Forty-eight adult male Wistar rats were randomly chosen as control, sham (animals only underwent TGI), treatment (100 and 300 mg/kg curcumin following TGI), and vehicle groups. The animals underwent global ischemia imposed by a 20 minute ligation of the bilateral common carotid arteries. Cell death and apoptosis in different areas of brain were evaluated after 3 or 4 weeks by Nissl staining and TUNEL assay respectively.Results:
The number of dark neurons and apoptotic cells significantly increased after TGI. The number of TUNEL-positive cells after TGI was significantly higher in the temporal neocortex than in different regions within the hippocampus. Treatment with curcumin at a high dose reduced the numbers of dark neurons and apoptotic cells. Lower concentrations of curcumin showed a neuroprotective effect in the neocortex, whereas higher doses prevented cell death and apoptosis in the neocortex and in different regions of the hippocampus.Conclusions:
Regional differences were evident with respect to the neuroprotective effects of curcumin in the temporal cortex and in the different parts of hippocampus following TGI. Further studies are needed to explore the mechanisms underlying these regional differences.Keywords
Apoptosis Cell death Curcumin Ischemia Neuroprotective Effects
1. Background
Ischemic brain injury is one of the most common causes of death and disability throughout the world (1) and is triggered by a variety of mechanisms. Recent studies show that damage to neurons after ischemia/reperfusion may occur through oxidative stress and/or mitochondrial impairment and may culminate in the activation of an apoptotic stage. For these reasons, antioxidant and anti-apoptotic agents have been the focus of studies for the development of neuroprotective drugs for use in stroke therapy. Various regions of the brain, including the hippocampus and neocortex, are extremely vulnerable to ischemia. Despite advances in the understanding of the pathophysiology of cerebral ischemia, therapeutic options remain limited (2).
The term neuro protection encompassesa series of mechanisms that protect against neuronal injury or degeneration in the central nervous system (CNS).These mechanisms prevent oxidative stress, mitochondrial dysfunction, excitotoxicity, inflammatory changes, iron accumulation, and protein aggregation. Enhanced production of reactive oxygen species (ROS) and subsequent oxidative stressare thought to play pivotal roles in neuronal death induced by ischemia/reperfusion (3). The goal of neuro protection is to limit neuronal dysfunction or neuronal death after CNS injury and to maintain cellular integrity. The mechanisms of endogenous neuro protection, which are triggered upon neural damage to overcome or limit its consequences, include gene expression alterations and the production of neurotrophic factors (4).
Curcumin is the principal curcuminoid of the popular spice turmeric, which is derived from (Terra merita (merited earth)) Cucurma longa, a member of the ginger family (Zingiberaceae). Some studies report that curcumin attenuates endoplasmic reticulum (ER) stress by controlling inflammation in brain injury and prevents neurotoxicity through suppression of oxidative stress (5, 6). It positively regulates adenosine monophosphate-activated protein kinase (AMPK) activity and this action contributes to the management of metabolic disorders (7).
Curcumin shows anti-inflammatory, antioxidant, anti-protein-aggregation, and anti tumoral activities (8, 9). Curcumin also prevents ultraviolet irradiation-induced apoptotic changes, including the loss of the mitochondrial membrane potential, mitochondrial release of cytochrome c, and increases in ROS (10). Several animal studies showed that administration of curcumin after brain ischemia had protective effects and significantly diminished lipid peroxidation, mitochondrial dysfunction, and glial activation, as well as infarct volume; moreover, it improved cognitive deficits, neurological scores, and locomotor activity (11-19). However, no studies have been reported that compare the antioxidant effects of curcumin on various regions of the brain.
2. Objectives
The aim of the present study was to compare the neuroprotective effects of different concentrations of curcumin in various regions of the brain after transient global ischemia (TGI).
3. Materials and Methods
3.1. Study Design
Forty-eight adult male Wistar rats (250 - 280g) were randomly divided into eight groups. All animals, except the control group, underwent brain ischemia. Under pentobarbital anesthesia, the cervical vessels were exposed through a medio-ventral cervical incision and the bilateral common carotid arteries were ligated for 20 minutes (3). The animals of the sham groups underwent global ischemia only and were decapitated after 3 (Isch 3W) or 4 weeks (Isch 4W). Curcumin (Sigma, USA) was dissolved in dimethyl sulfoxide (DMSO) and administered intraperitoneally (i.p., two doses). Four groups were treated with curcumin at concentrations of 100 or 300 mg/kg, at the onset of reperfusion and 24 hours later (14). The rats were decapitated after 3 (Isch + C100 3W, Isch + C300 3W) or 4 (Isch + C100 4W, Isch + C300 4W) weeks. The last group of animals was treated with vehicle (DMSO) following ischemia. The animals were allowed free access to food and water and were kept in a twelve-hour light-dark cycle. The animal protocol was approved by the Tehran University of Medical Sciences.
3.2. Preparation of Brain Sections
The rats were decapitated under anesthesia (100 mg/kg ketamine and 10 mg/kg xylazine; 10:1) and tissue was fixed by transcardial perfusion with 0.9% saline, followed by 4% paraformaldehyde in PBS. Brains were removed, fixed in 4% paraformaldehyde for 24 hours, and then stored in a 30% sucrose solution (prepared with 0.1 M PBS, pH 7.2 - 7.4) at 4ºC for 24 - 48 h. The brains were cut into 3 - 5 mm sections, dehydrated in an ethanol series, cleared in xylene, and infiltrated and embedded in paraffin. Paraffin embedded tissues were prepared on slides for Nissl and TUNEL staining.
3.3. Cresyl Violet and TUNEL Staining
The dark neurons were visualized by staining for the histological features of neuronal injury with cresyl violet (Nissl staining). The terminal deoxynucleotidyl transferase-mediated dUTP nick-end labelling (TUNEL) assay was also performed to stain for DNA fragmentation after apoptotic cell death (Cell Death Detection Kit, Roche, Mannheim, Germany). Briefly, three 5 μm-thick tissue sections from each block were dewaxed and dehydrated by heating to 60ºC, followed by washing in xylene, and rehydration through a diluted alcohol series. The sections were then washed with 10 mM Tris-HCl (pH 7.6), followed by incubation in methanol containing 0.3% H2O2 for 10 minutes to inhibit endogenous peroxidase activity. The sections were then treated with proteinase K (Roche, 20 µg/ml in Tris buffer) at 37ºC for 30 minutes. The sections were incubated in the TUNEL reaction mixture (450 µl labeling solution and 50 µl enzyme solution) at 37ºC for 60 minutes and in POD solution for 30 minutes. The color reaction was developed in 3 3’ diaminobenzidine (DAB, Roche; 0.5 µl DAB and 1.5µl peroxide buffer) for 5 - 10 minutes, and the sections were counterstained with hematoxylin.
3.4. Cell Quantification
For each animal, the cells were counted from the injured area (equivalent adult rat bregma coordinates -2.52 to -4.56 mm) in the dorsal part of the hippocampus cornu ammonis (CA1, CA2, and CA3 areas) and DG, as well as the temporal cortex. At least three brain sections were selected from each animal (a total of 18 from each group) for each staining type. Cell counting was performed twice for each section. Sections were observed on an Olympus microscope (CX31, Tokyo, Japan) with a 40× objective lens. Images were captured using a digital camera (Olympus, Japan) and displayed on a computer monitor. In Nissl stained sections, the number of dark neurons counted was used as an index of cell death (20). The number of TUNEL-positive neurons was considered as an apoptosis index and calculated by counting 500 cells in each specimen (five visual fields / specimen) (21).
Statistical analysis
Results were expressed as the mean ± SEM. Differences between means were determined by one-way analysis of variance (ANOVA), followed by a post-hoc test using the Least Significant Difference method or non-parametric tests (Kruskal-Wallis). P < 0.05 was considered statistically significant.
4. Results
4.1. Evaluation of Dark Neurons
The Isch 3W, Isch 4W, and vehicle groups, when compared to control rats, showed significantly increased numbers of dark cells in all investigated brain regions (the temporal neocortex and the hippocampal CA1, CA2, and CA3 areas, as well as the hippocampal DG) after TGI (P < 0.05). Treatment with 100 mg/kg curcumin following ischemia significantly decreased the number of dark neurons in the hippocampal CA3 area and in the temporal neocortex after 4 weeks, when compared to the Isch 4W group (P < 0.05). No significant differences were not observed between the Isch + C100 3W and Isch 3W groups with respect to the number of dark cells in any of the tested brain regions. Treatment with 300 mg/kg curcumin after ischemia decreased the number of dark cells in the hippocampal CA1 and CA2 regions and in the temporal neocortex after 3 weeks, when compared to the Isch 3W group (P < 0.05). The number of dark neurons in all investigated brain areas was significantly lower in the Isch + C300 4W group than in the Isch 4 W group (P < 0.05; Figures 1 and 2).
The Effect of Different Concentrations of Curcumin on the Number of Dark Neurons in the Temporal Neocortex, as Well as in the Hippocampal DG, CA1, CA2, and CA3 Areas, Following Transient Global Ischemia in Rats
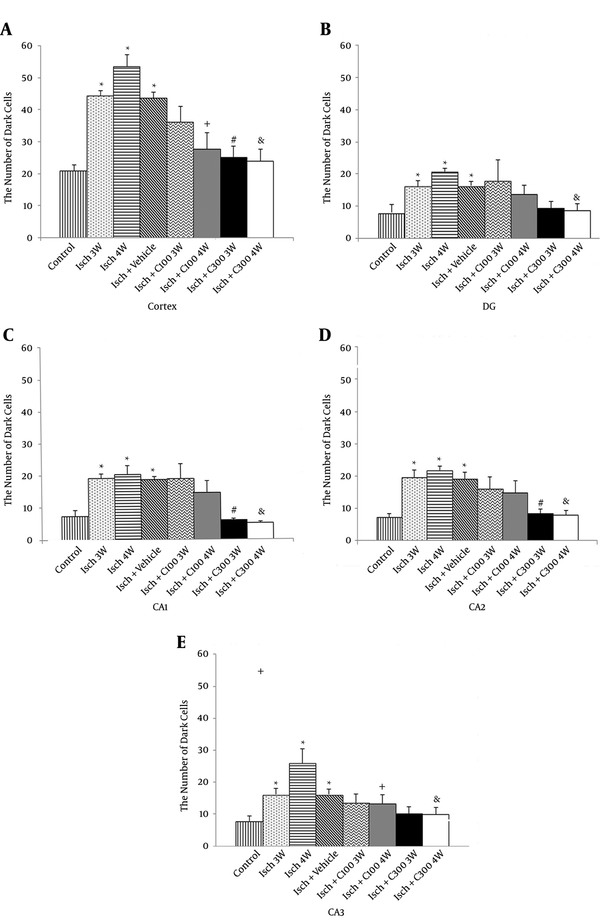
Photomicrographs Showing the Morphological Features of the Hippocampal DG, CA1, CA2, and CA3 Areas and the Temporal Neocortex in the Different Groups Following Cresyl Violet Staining
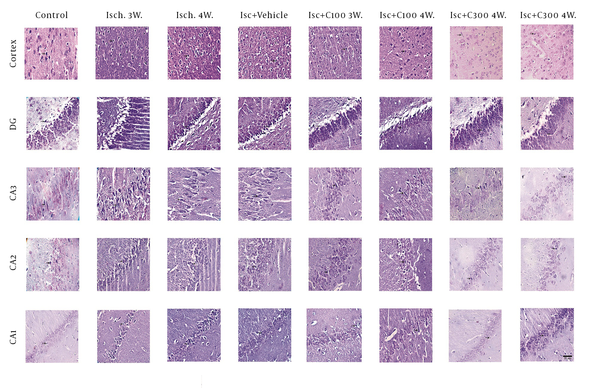
4.2. Assessment of TUNEL-positive cells
An increased number of apoptotic cells appeared in the Isch 3W, Isch 4W, and vehicle groups in all the assessed brain regions when compared to the control group (P < 0.05). Treatment with 100 mg/kg curcumin in the Isch + C100 3W group significantly decreased the TUNEL positive cells compared to the Isch 3W group, but only in the temporal neocortex (P < 0.05). The Isch + C100 4W group displayed a reduction in the number of apoptotic cells in the hippocampal CA2 area and in the temporal neocortex when compared to the Isch 4W group (P < 0.05). A significant decrease in the number of TUNEL positive neurons was observed in all investigated brain areas in the Isch + C300 4W group when compared to the Isch 4 W group (P < 0.05; Figures 3 and 4).
The Effect of Different Concentrations of Curcumin on Apoptotic Cell Death in the Temporal Neocortex, as Well as in the Hippocampal DG, CA1, CA2, and CA3 Areas, Following Transient Global Ischemia in Rats
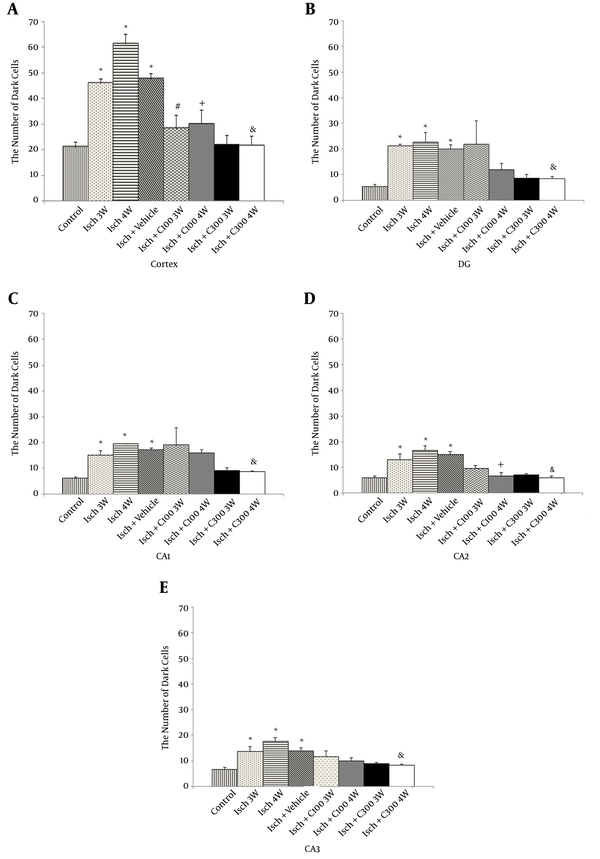
Photomicrographs of the Different Groups Showing the Morphological Features of the Hippocampal DG, CA1, CA2, and CA3 Areas and the Temporal Neocortex Following TUNEL Staining
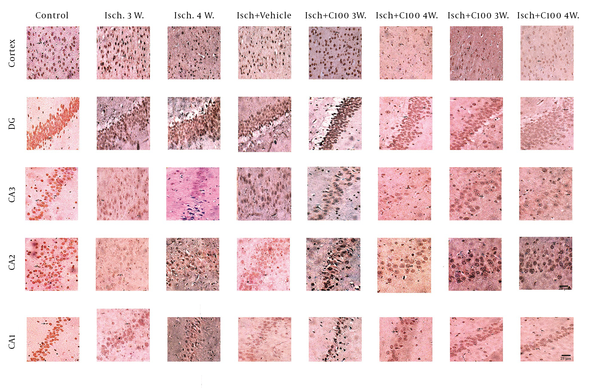
5. Discussion
The results of this study confirmed that treatment with curcumin at a high dose reduced cell death and apoptotic processes in the temporal cortex and in different parts of the hippocampus of rats after TGI. A lower concentration of curcumin showed a neuroprotective effect in the neocortex, whereas the higher dose prevented cell death and apoptosis in both the neocortex and in the different regions of the hippocampus. The administered drug doses and ischemia duration in each experiment were chosen based on a pilot study and previous work (22-25).
Extensive neuronal injury and death have been reported in the lateral striatum, hippocampus, and neocortex after TGI (23). Our data indicated that apoptosis was significantly higher in the temporal neocortex than in the different regions of the hippocampus after TGI. By contrast, several previous studies have indicated that the hippocampus is more sensitive to transient ischemia than is the neocortex (26, 27). Yang et al. have shown that curcumin promoted the synthesis and secretion of monoamine neurotransmitters in hippocampal tissue, and this led to the improvement of neurological functions. Curcumin also inhibited ROS production in the hippocampus by changing the ER stress markers and by positive regulation of AMPK (28, 29).This discrepancy in regional responses may be attributed to the different durations of ischemia, the experimental procedures, the animal species used, or the different times of injury evaluation. Our study also indicated a reduction in the number of dark neurons in response to the higher curcumin dose, as well as a suppression of the DNA fragmentation induced by TGI. Curcumin treatment dose-dependently reduced the infarct volume in a focal cerebral ischemia model induced in rats by middle cerebral artery occlusion (MCAO) (24).
A pharmacokinetic study conducted after i.p. administration of curcumin (100 mg/kg) in mice showed a plasma concentration of 2.25 µg/ml during the first 15 min of treatment. However, only traces (0.41 µg/g) were observed in the brain one hour after administration (30). Therefore, low levels of curcumin may possibly be insufficient to protect the highly sensitive hippocampal neurons. However, further studies are required to resolve this issue.
Curcumin is thought to exert its anti-apoptotic and neuroprotective actions through multiple effects, including strong anti-inflammatory, radical scavenging, and neuromodulating activities (31). Damage to cells may occur through oxidative stress and/or mitochondrial impairment and may trigger activation cascades leading to apoptosis. Many studies have demonstrated that apoptosis occurring during ischemia/reperfusion plays a major role in stroke-associated brain injury (32). Apoptosis may therefore bring about cell death following cerebral ischemia.
Caspase-3 is activated in the apoptotic cell, both by extrinsic (death ligand) and intrinsic (mitochondrial) pathways. Both the expression level and the number of positive neurons expressing the activated caspase-3 were reduced in the same brain areas by curcumin treatment (33). A low dosage of curcumin was safe and reversed Cu (II)-induced neuronal damage by scavenging intracellular ROS. At high dosages, however, curcumin facilitated the generation of free radicals by Cu and exacerbated neuronal damage (34). The protective effect of curcumin observed in the present study may reflect its antioxidant and anti-inflammatory activities at low doses (35). At higher doses, curcumin can act as an alkylating agent, there by stimulating antioxidant response element-mediated expression of protective genes (36).
In conclusion, the results of this study demonstrated regional differences in the neuroprotective effects of curcumin in different brain regions in the rat following TGI. Further experiments are needed to investigate the mechanisms and to determine the functional implications of these regional differences in curcumin effects. Curcumin is currently undergoing human clinical trials for the treatment of inflammatory-linked diseases and several types of cancer (37). Our results indicate that the dosage of curcumin should be controlled if it is intended to counter neurological disorders in clinical therapy.
References
-
1.
Liu XQ, Sheng R, Qin ZH. The neuroprotective mechanism of brain ischemic preconditioning. Acta Pharmacol Sin. 2009;30(8):1071-80. [PubMed ID: 19617892]. https://doi.org/10.1038/aps.2009.105.
-
2.
Donnan GA, Baron JC, Ma H, Davis SM. Penumbral selection of patients for trials of acute stroke therapy. The Lancet Neurology. 2009;8(3):261-9. https://doi.org/10.1016/S1474-4422(09)70041-9.
-
3.
Bayat M, Azami Tameh A, Hossein Ghahremani M, Akbari M, Mehr SE, Khanavi M, et al. Neuroprotective properties of Melissa officinalis after hypoxic-ischemic injury both in vitro and in vivo. Daru. 2012;20(1):42. [PubMed ID: 23351182]. https://doi.org/10.1186/2008-2231-20-42.
-
4.
Wang R, Li YB, Li YH, Xu Y, Wu HL, Li XJ. Curcumin protects against glutamate excitotoxicity in rat cerebral cortical neurons by increasing brain-derived neurotrophic factor level and activating TrkB. Brain Res. 2008;1210:84-91. [PubMed ID: 18420184]. https://doi.org/10.1016/j.brainres.2008.01.104.
-
5.
Chhunchha B, Fatma N, Kubo E, Rai P, Singh SP, Singh DP. Curcumin abates hypoxia-induced oxidative stress based-ER stress-mediated cell death in mouse hippocampal cells (HT22) by controlling Prdx6 and NF-kappaB regulation. Am J Physiol Cell Physiol. 2013;304(7):C636-55. [PubMed ID: 23364261]. https://doi.org/10.1152/ajpcell.00345.2012.
-
6.
Wu J, Li Q, Wang X, Yu S, Li L, Wu X, et al. Neuroprotection by curcumin in ischemic brain injury involves the Akt/Nrf2 pathway. PLoS One. 2013;8(3). ee59843. [PubMed ID: 23555802]. https://doi.org/10.1371/journal.pone.0059843.
-
7.
Soetikno V, Sari FR, Sukumaran V, Lakshmanan AP, Harima M, Suzuki K, et al. Curcumin decreases renal triglyceride accumulation through AMPK-SREBP signaling pathway in streptozotocin-induced type 1 diabetic rats. J Nutr Biochem. 2013;24(5):796-802. [PubMed ID: 22898567]. https://doi.org/10.1016/j.jnutbio.2012.04.013.
-
8.
Hamaguchi T, Ono K, Yamada M. REVIEW: Curcumin and Alzheimer's disease. CNS Neurosci Ther. 2010;16(5):285-97. [PubMed ID: 20406252]. https://doi.org/10.1111/j.1755-5949.2010.00147.x.
-
9.
Ringman JM, Frautschy SA, Cole GM, Masterman DL, Cummings JL. A potential role of the curry spice curcumin in Alzheimer's disease. Curr Alzheimer Res. 2005;2(2):131-6. [PubMed ID: 15974909].
-
10.
Chan WH, Wu CC, Yu JS. Curcumin inhibits UV irradiation-induced oxidative stress and apoptotic biochemical changes in human epidermoid carcinoma A431 cells. J Cell Biochem. 2003;90(2):327-38. [PubMed ID: 14505349]. https://doi.org/10.1002/jcb.10638.
-
11.
Yang C, Zhang X, Fan H, Liu Y. Curcumin upregulates transcription factor Nrf2, HO-1 expression and protects rat brains against focal ischemia. Brain Res. 2009;1282:133-41. [PubMed ID: 19445907]. https://doi.org/10.1016/j.brainres.2009.05.009.
-
12.
Al-Omar FA, Nagi MN, Abdulgadir MM, Al Joni KS, Al-Majed AA. Immediate and delayed treatments with curcumin prevents forebrain ischemia-induced neuronal damage and oxidative insult in the rat hippocampus. Neurochem Res. 2006;31(5):611-8. [PubMed ID: 16770732]. https://doi.org/10.1007/s11064-006-9059-1.
-
13.
Shukla PK, Khanna VK, Ali MM, Khan MY, Srimal RC. Anti-ischemic effect of curcumin in rat brain. Neurochem Res. 2008;33(6):1036-43. [PubMed ID: 18204970]. https://doi.org/10.1007/s11064-007-9547-y.
-
14.
Wang Q, Sun AY, Simonyi A, Jensen MD, Shelat PB, Rottinghaus GE, et al. Neuroprotective mechanisms of curcumin against cerebral ischemia-induced neuronal apoptosis and behavioral deficits. J Neurosci Res. 2005;82(1):138-48. [PubMed ID: 16075466]. https://doi.org/10.1002/jnr.20610.
-
15.
Huang S, Wang B, Zhang ZQ, Meng ZY, Cao H, Lian QQ, et al. [Effect of curcumin on the expression of high mobility group box 1 and apoptotic neurons in hippocampus after global cerebral ischemia reperfusion in rats]. Zhonghua Yi Xue Za Zhi. 2011;91(19):1340-3. [PubMed ID: 21756762].
-
16.
Zheng YY, Yu LS, Zhang YG, Ye GH, Yi JP. [Effects of curcumin on malondialdehyde and c-fos protein in hypoxia ischemia brain tissue in rats]. Fa Yi Xue Za Zhi. 2009;25(1):6-8. [PubMed ID: 19397204].
-
17.
Zhuang R, Lin MX, Song QY, Li J. Effects of curcumin on the expression of nuclear factor-kappaB and intercellular adhesion molecular 1 in rats with cerebral ischemia-reperfusion injury. J. South. Med. Univ. 2009;29(6):1153-5.
-
18.
Yu G, Liu L, Zhang P, Li YB. Protective effect of Curcumin on chronic cerebral ischemia by altering expression of α-synuclein in 2VO model. Molecular neurodegeneration. 2012;7(1):1.
-
19.
Liu L, Zhang P, Li Y, Yu G. Curcumin protects brain from oxidative stress through inducing expression of UCP2 in chronic cerebral hypoperfusion aging-rats. Molecular neurodegeneration. 2012;7(Suppl 1):S10.
-
20.
Ooigawa H, Nawashiro H, Fukui S, Otani N, Osumi A, Toyooka T, et al. The fate of Nissl-stained dark neurons following traumatic brain injury in rats: difference between neocortex and hippocampus regarding survival rate. Acta Neuropathol. 2006;112(4):471-81. [PubMed ID: 16858608]. https://doi.org/10.1007/s00401-006-0108-2.
-
21.
Darzynkiewicz Z, Galkowski D, Zhao H. Analysis of apoptosis by cytometry using TUNEL assay. Methods. 2008;44(3):250-4. [PubMed ID: 18314056]. https://doi.org/10.1016/j.ymeth.2007.11.008.
-
22.
Lee SR, Tsuji K, Lee SR, Lo EH. Role of matrix metalloproteinases in delayed neuronal damage after transient global cerebral ischemia. J Neurosci. 2004;24(3):671-8. [PubMed ID: 14736853]. https://doi.org/10.1523/JNEUROSCI.4243-03.2004.
-
23.
Liu J, Solway K, Messing RO, Sharp FR. Increased neurogenesis in the dentate gyrus after transient global ischemia in gerbils. J Neurosci. 1998;18(19):7768-78. [PubMed ID: 9742147].
-
24.
Thiyagarajan M, Sharma SS. Neuroprotective effect of curcumin in middle cerebral artery occlusion induced focal cerebral ischemia in rats. Life Sci. 2004;74(8):969-85. [PubMed ID: 14672754].
-
25.
Zhao J, Zhao Y, Zheng W, Lu Y, Feng G, Yu S. Neuroprotective effect of curcumin on transient focal cerebral ischemia in rats. Brain Res. 2008;1229:224-32. [PubMed ID: 18640105]. https://doi.org/10.1016/j.brainres.2008.06.117.
-
26.
Pulsinelli WA, Brierley JB, Plum F. Temporal profile of neuronal damage in a model of transient forebrain ischemia. Ann Neurol. 1982;11(5):491-8. [PubMed ID: 7103425]. https://doi.org/10.1002/ana.410110509.
-
27.
Yoshimine T, Yanagihara T. Regional cerebral ischemia by occlusion of the posterior communicating artery and the middle cerebral artery in gerbils. J Neurosurg. 1983;58(3):362-7. [PubMed ID: 6827320]. https://doi.org/10.3171/jns.1983.58.3.0362.
-
28.
Li Y, Li J, Li S, Li Y, Wang X, Liu B, et al. Curcumin attenuates glutamate neurotoxicity in the hippocampus by suppression of ER stress-associated TXNIP/NLRP3 inflammasome activation in a manner dependent on AMPK. Toxicol Appl Pharmacol. 2015;286(1):53-63. [PubMed ID: 25791922]. https://doi.org/10.1016/j.taap.2015.03.010.
-
29.
Yang J, Song S, Li J, Liang T. Neuroprotective effect of curcumin on hippocampal injury in 6-OHDA-induced Parkinson's disease rat. Pathol Res Pract. 2014;210(6):357-62. [PubMed ID: 24642369]. https://doi.org/10.1016/j.prp.2014.02.005.
-
30.
Pan MH, Huang TM, Lin JK. Biotransformation of curcumin through reduction and glucuronidation in mice. Drug Metab Dispos. 1999;27(4):486-94. [PubMed ID: 10101144].
-
31.
Rinwa P, Kumar A, Garg S. Suppression of neuroinflammatory and apoptotic signaling cascade by curcumin alone and in combination with piperine in rat model of olfactory bulbectomy induced depression. PLoS One. 2013;8(4). ee61052. [PubMed ID: 23613781]. https://doi.org/10.1371/journal.pone.0061052.
-
32.
Nadia Sharifi Z, Movassaghi S, Mohamadzadeh F, Soleimani Asl S, Pourheydar B, Mehdizadeh M. Reduction in ischemic brain injury following the administration of pentoxifylline after transient global ischemia/ reperfusion in a rat model. Med J Islam Repub Iran. 2015;29:193. [PubMed ID: 26157711].
-
33.
Curic S, Wu Y, Shan B, Schaaf C, Utpadel D, Lange M, et al. Curcumin acts anti-proliferative and pro-apoptotic in human meningiomas. J Neurooncol. 2013;113(3):385-96. [PubMed ID: 23666203]. https://doi.org/10.1007/s11060-013-1148-9.
-
34.
Huang HC, Lin CJ, Liu WJ, Jiang RR, Jiang ZF. Dual effects of curcumin on neuronal oxidative stress in the presence of Cu(II). Food Chem Toxicol. 2011;49(7):1578-83. [PubMed ID: 21501647]. https://doi.org/10.1016/j.fct.2011.04.004.
-
35.
Dhandapani KM, Mahesh VB, Brann DW. Curcumin suppresses growth and chemoresistance of human glioblastoma cells via AP-1 and NFkappaB transcription factors. J Neurochem. 2007;102(2):522-38. [PubMed ID: 17596214]. https://doi.org/10.1111/j.1471-4159.2007.04633.x.
-
36.
Dikshit P, Goswami A, Mishra A, Nukina N, Jana NR. Curcumin enhances the polyglutamine-expanded truncated N-terminal huntingtin-induced cell death by promoting proteasomal malfunction. Biochem Biophys Res Commun. 2006;342(4):1323-8. [PubMed ID: 16516148]. https://doi.org/10.1016/j.bbrc.2006.02.104.
-
37.
Hatcher H, Planalp R, Cho J, Torti FM, Torti SV. Curcumin: from ancient medicine to current clinical trials. Cell Mol Life Sci. 2008;65(11):1631-52. [PubMed ID: 18324353]. https://doi.org/10.1007/s00018-008-7452-4.