Abstract
Background:
Bisphenol A (BPA) has been acknowledged as an estrogenic chemical able to interact with estrogen receptors. Bisphenol A at high concentration inhibited the GABAA receptor mediated response.Objectives:
The study was conducted to investigate the effect of BPA on GABAAα1 receptors density in amygdala and cerebellum of adult rats and the possible modulatory effect of passive avoidance learning.Methods:
Thirty male Sprague-Dawley rats weighting 200 to 220 g were used. Bisphenol A 5 and 50 mg kg-1 day-1 were administrated by oral intake for 15 days. Learning and memory tasks were performed by shuttle-box. Densities of GABAAα1 receptor were investigated by immunohistochemical procedure.Results:
Rats with learning had significantly higher GABAAα1 receptor as compared to control rats. The administration of BPA at both low and high doses significantly reduced receptor density in a dose-dependent manner in comparison with control rats. Learning significantly increased receptor density in rats treated with BPA as compared to unlearned BPA treated rats, however, it could not completely reverse this parameter and these rats still had lower receptor density than controls.Conclusions:
In conclusion, BPA induces dose-dependent down-regulation of GABAAα1 receptors in the amygdala and Purkinje cell layer of cerebellum of adult rats, which could be partially compensated by learning.Keywords
GABAAα1 Receptors Density Bisphenol A Learning Amygdala Cerebellum
1. Background
Endocrine disruptors are synthetic or natural substances with the ability to affect the function of endogenous hormones by mimicking or blocking their actions and may also modify synthesis, transport, metabolism or elimination of natural hormones (1). Recently, the general public has received alarming reports regarding the reproduction and health hazards of endocrine disrupting chemicals in the environment (2). One of the most common endocrine disruptors is Bisphenol A (BPA) (3). Bisphenol A is a well-known ‘environmental endocrine disrupter’ with mixed estrogen agonist/antagonist properties (4). This substance has two phenol rings with structural homology with a ring of ß-estradiol and is suspected to bind to estrogen receptors, which leads to adverse effects on humans and wild animals (5).
Furthermore, γ-Amino Butyric Acid (GABA) is a major inhibitory neurotransmitter throughout the central nervous system that acts on GABAA, GABAB and GABAC receptors. The receptors of GABAA mainly mediate fast inhibitory synaptic transmissions in the whole brain, and thus they are important targets for many pharmacological agents such as synthetic and endogenous steroids (6). McCabe et al. (2001) reported that in vitro GABA release in intermediate and medial hyper striatum ventral slices of chick brain increases following training (7). There is a profound difference in affinity of GABAA receptors of different brain regions. The use of molecular biological techniques has revealed that a single amino acid is responsible for this difference in affinity and the function of the receptor. The presence of a histidine residue at position 101 of the primary amino acid sequence of the α subunit produces α1 subunit, which confers high affinity for binding of diazepam (8). Behavioral examination of genetically modified animals has suggested that the α1 subunit-containing GABAA receptors comprise the memory modulating population of these receptors (9). Savic et al. (2005) showed that α1 subunit-containing GABAA receptors have an important role in modulation of memory acquisition (10).
Several studies have shown that exposure of animals to BPA in prenatal and neonatal stages as well as in adulthood can induce impairment of learning and memory (2, 11, 12). In 2007, Choi et al. reported that BPA could act through GABAA receptors. However, it is not likely that BPA acts on the GABA binding sites of GABAA receptors to open Cl- channels, since it did not induce any GABAA receptor-mediated currents in dorsal root ganglion neurons, which express functional GABA receptors. Instead, BPA may bind to the modulatory sites located close to the GABAA receptor Cl- channel complex, and thus affects GABAA receptor function (11).
As far as we know, the effect of BPA on GABAA receptor density and the possible modulatory effect of learning have not been addressed yet.
2. Objectives
The present study was conducted to investigate the effect of short-term repeated dose administration of BPA at two doses (high and low) on GABAAα1 receptors density in amygdala and cerebellum of adult rats and the possible modulatory effect of passive avoidance learning.
3. Methods
3.1. Animals and Experimental Design
Thirty adult male Sprague Dawley rats 10 - 12 weeks old and weighing 200 - 220g were used. Food and water were available ad libitum during the study. The rats were housed under a 12-hour light/dark (lights on at 6 am) and controlled temperature (20 ± 4°C) condition.
After a week of adaptation, animals were randomly allocated to six equal groups (n = 5 each) and treated as follows: group1 (sham l): received sesame oil on a daily basis by oral gavages in a volume similar to BPA administered to rats with the same weight for 15 days; group 2 (sham 2): received sesame oil as above accompanied by learning; group 3 (experimental 1): received BPA 5 mg kg-1 day-1 by oral gavages (12) for 15 days; group 4: (experimental 2) received BPA 50 mg kg-1 day-1 by oral gavages for 15 days; group 5 (experimental 3): received BPA 5 mg kg-1 day-1 by oral gavages for 15 days with learning and group 6 (experimental 4): received BPA 50 mg kg-1 day-1 by oral gavages for 15 days with learning. Bisphenol A was purchased from sigma-Aldrich Co. and dissolved in sesame oil.
3.2. Learning Procedure
A two-way shuttle-box with acrylic walls and steel floor bars was used. The box, 44 × 20 × 19 cm, was bisected by a vertical partition with an opening in the middle that allows the animal to move freely from one compartment to other, including light and dark compartments. In the light compartment, the animal was safe while in the dark compartment it received a foot shock of 0.6 mA for one second, with a latent period of one second. On the first day, all animals were individually subjected to two minutes of adaptation to the shuttle box, in which the rat could explore the light compartment and move about freely. At this stage since the rat prefers the dark compartment, if the rat did not move to the dark compartment after 120 seconds, it was excluded from the study. This adaptation was repeated 30 minutes later. On the second day, the rats were placed in the light compartment box and one second after entering the dark compartment received a 0.6 mA foot shock for one second, this session was considered as initial latency. On the third day, the procedure was similar with the second day, and was considered as the learning session. On the fourth day, as memory consolidation, the procedure was like the learning days without foot shock and was considered as the consolidation session. On the fifth day, which was the memory retention session, the procedure was similar to the fourth day. The rats were thought to have completely learned the adaptation, if they did not move to the dark compartment after 120 second during third, fourth and fifth sessions of the experiment.
All the procedures involving animal subjects were reviewed and approved by the Institutional Research Ethics Committee of the School of Veterinary Medicine of Shiraz University.
3.3. Sample Preparation
At the end of the experiment, rats from all groups were anesthetized by sodium thiopental and their hearts were perfused with 10% formaldehyde. Then the whole brain and cerebellum were removed and washed with normal saline and fixed for 24 hours in 10% formaldehyde in 0.1 M Phosphate Buffer (pH 7.4), which was followed by post-fixing in 4% formaldehyde in 0.1 M phosphate buffer (pH 7.4). Tissue processing was accomplished in an automated tissue processor and paraffin blocks were prepared. Furthermore, 5 μm serial sections of amygdala and cerebellum were made and mounted on 25% L-lysine coated glass slides.
3.4. Immunohistochemical Study
The slide-mounted sections were dried and subsequently stored in a -20°C freezer until use for antibody labeling. After removal from the freezer, slide-mounted sections were brought to room temperature, washed and outlined with a liquid-repellent slide marker pen. Sections were incubated over night with primary antibodies against GABAAα1 (abcam, UK) (with a dilution of 1 × 10-3). The next day, sections were rinsed with PBS and incubated in secondary antibody (DAKO Co Denmark) (envision) and further washed in PBS. Finally, the sections were rinsed in PBS three times for 10 minutes and reacted with cromogen (Dab) (DAKO Co Denmark) followed by a rinse in PBS. Then the sections were placed in hematoxylin for nucleus staining. Negative control sections were incubated with PBS in the absence of primary antibody and no immunoreactivity was detected in amygdala and purkinje cell layer of cerebellum. Figure 1 shows the negative control of cerebellum and amygdala sections. After preparing digital images from slides, density of GABAAα1 receptors was assayed by using the Image Analyzer (version 1.33) software. This program determines the density of receptors, according to three characters including hue, saturation and intensity, and shows a number that has reverse relation with the receptor density; this means that higher receptor density was represented by lower number in the program.
Photograph of Negative Control of Brain A) Purkinje Cell Layer of Cerebellum and B) Amygdala (Magnification 40 × 10)
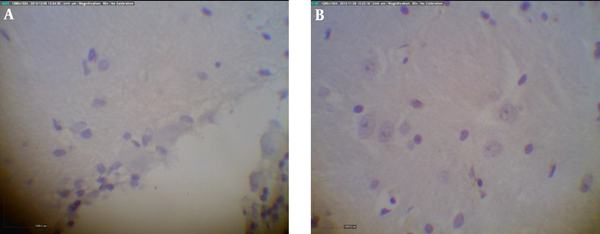
3.5. Statistical Analysis
Statistical analysis was performed using the SPSS (version 18) software. One-way analysis of variance (ANOVA) method and Tukey’s test as the post hoc were used to determine the difference between groups. P < 0.05 was considered as the level of significance and data was reported as mean ± standard error of the mean (SEM).
4. Results
According to our data, exposure to BPA with doses of 5 and 50 mg kg-1 day-1 for 15 days without learning, significantly (P < 0.05) decreases immunoreactive GABAAα1 receptor density in Purkinje cell of cerebellum and amygdala in comparison to sham 1 (Figures 2, 3, 4, 5).
Color intensity (mean and SEM) of immunohistochemically stained amygdala slides of rats in different groups, which inversely demonstrates GABAAα1 receptor density; different letters show significant differences (P < 0.05).
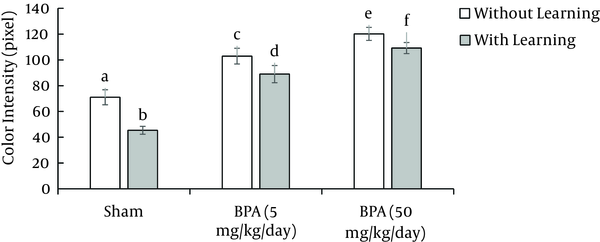
Photograph of the Effect of Bisphenol A on GABAAα1 Receptor Density in Amygdala of Male Rats in Different Groups (Magnification 40 × 10)
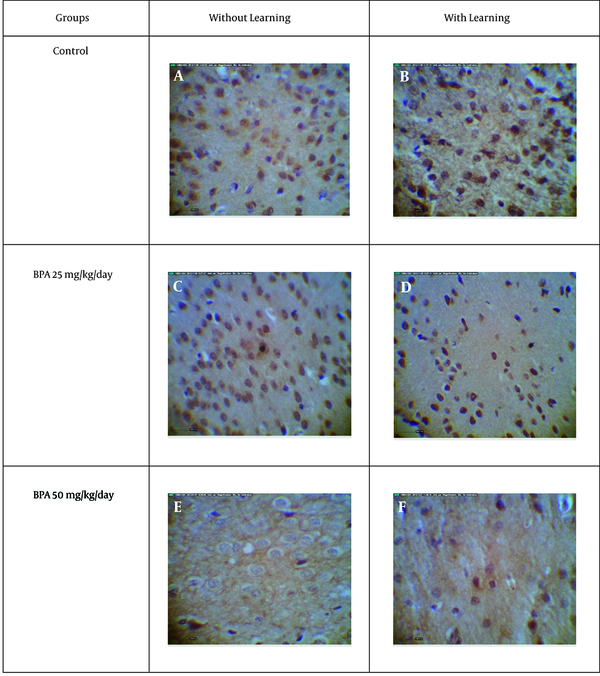
Color intensity (mean and SEM) of immunohistochemically stained cerebellum slides of rats in different groups which inversely demonstrates GABAAα1 receptor density; different letters indicate significant differences (P < 0.05).
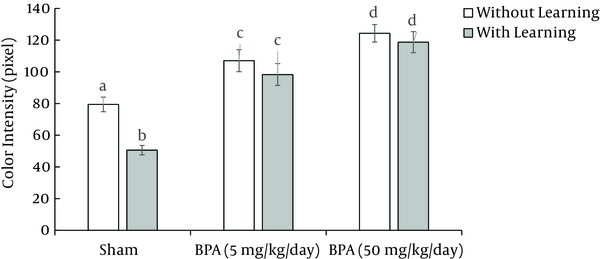
Photograph of Effect of Bisphenol A on GABAAα1 Receptor Density in Purkinje Cell Layer of Cerebellum of Male Rats in Different Groups (Magnification 40 × 10)
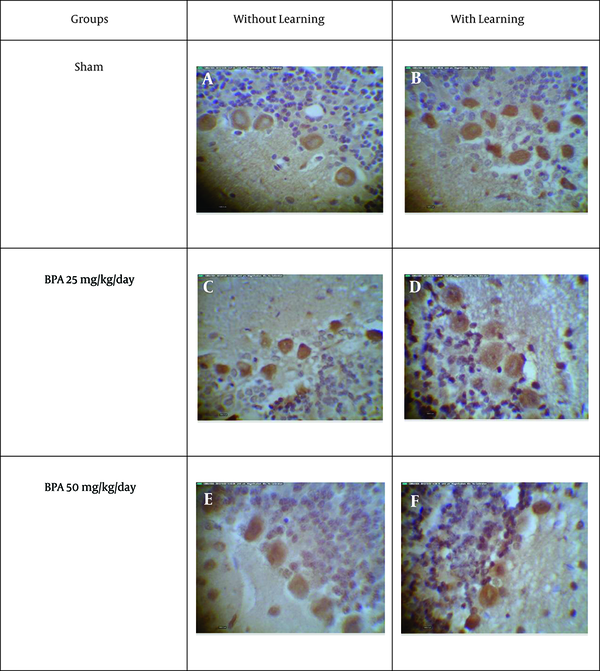
On the other hand, exposure to BPA at doses 5 and 50 mg kg-1 day-1 in 15 days with learning also significantly (P < 0.05) decreases immunoreactive GABAAα1 receptor density in Purkinje cell of cerebellum and amygdala in comparison to sham 2 (Figures 2, 3, 4, 5). There was a significant difference (P < 0.05) between sham 1 and sham 2 that is, in sham 2 group immunoreactive GABAAα1 receptor density in Purkinje cell of cerebellum and amygdala was higher than sham 1; so learning led to an increase in immunoreactive GABA Aα1 receptor density in Purkinje cell of cerebellum and amygdala (Figures 2, 3, 4, 5).
However, comparison between with and without learning groups that were exposed to BPA at two doses, revealed that the immunoreactive GABAAα1 receptor density was significantly (P < 0.05) lower in without learning groups relative to groups with learning in amygdala but not in Purkinje cell of cerebellum (Figures 2, 3, 4, 5). This means that learning can compensate the effect of BPA on GABAAα1 receptor density in amygdala but not in Purkinje cell of cerebellum.
5. Discussion
One of the main findings of the present study was that short-term repeated dose treatment with BPA induces dose-dependent down-regulation of GABAAα1 receptors in the amygdala and Purkinje cell layer of cerebellum of adult rats. Noriega et al., (2009) observed that 17 β-estradiol administration to ovariectomized rhesus macaque reduces GABAAα1 expression in amygdala as compared to controls. Regarding the fact that BPA mimics the action of the natural hormone 17 β-estradiol (13), it is speculated that the decreased GABAAα1 receptor density in our study may be the result of decreased expression of its gene due to estradiol-mimicking effects of BPA. Although this hypothesis needs to be investigated in future studies. The role of GABAA receptors in learning has been clearly demonstrated previously. Zheng et al. (2007) reported that both the protein and mRNA of GABAA receptor a1 subunit in hippocampus and prefrontal cortex of rat brain were affected by acute restraint stress. They suggest GABAA receptor could play a role in the regulation of learning and memory (14). It was demonstrated that inhibitory transmission mediated by GABAAα1 receptors plays a critical role in amygdala plasticity and fear learning. Lech et al., (2001) reported that learning-associated activation of the cerebral cortex increases expression of GABAAα1 receptor mRNA after a short post-training delay. Consistent with this, another interesting finding of our study was that learning has a compensatory effect and mitigates receptor loss due to BPA administration. The increased density of GABAAα1 can be caused either by increased synthesis of receptors or by changes in subunit composition. Changes in subunit composition of GABAA receptors in the hippocampus were found in the kindling and kainic acid induced epilepsy in rats and subunit switching was also found in hypothalamic oxytocin neurons in lactation–pregnancy cycle (15).
In conclusion, short-term repeated dose treatment with BPA induces dose-dependent down-regulation of GABAAα1 receptors in the amygdala and Purkinje cell layer of cerebellum of adult rats, which could be partially compensated by learning.
Acknowledgements
References
-
1.
Hanet N, Lancon A, Delmas D, Jannin B, Chagnon MC, Cherkaoui-Malki M, et al. Effects of endocrine disruptors on genes associated with 17beta-estradiol metabolism and excretion. Steroids. 2008;73(12):1242-51. [PubMed ID: 18634814]. https://doi.org/10.1016/j.steroids.2008.06.005.
-
2.
Miyagawa K, Narita M, Narita M, Akama H, Suzuki T. Memory impairment associated with a dysfunction of the hippocampal cholinergic system induced by prenatal and neonatal exposures to bisphenol-A. Neurosci Lett. 2007;418(3):236-41. [PubMed ID: 17467901]. https://doi.org/10.1016/j.neulet.2007.01.088.
-
3.
Welshons WV, Nagel SC, vom Saal FS. Large effects from small exposures. III. Endocrine mechanisms mediating effects of bisphenol A at levels of human exposure. Endocrinology. 2006;147(6 Suppl):S56-69. [PubMed ID: 16690810]. https://doi.org/10.1210/en.2005-1159.
-
4.
Diamanti-Kandarakis E, Bourguignon JP, Giudice LC, Hauser R, Prins GS, Soto AM, et al. Endocrine-disrupting chemicals: an Endocrine Society scientific statement. Endocr Rev. 2009;30(4):293-342. [PubMed ID: 19502515]. https://doi.org/10.1210/er.2009-0002.
-
5.
Corrieri L, Della Seta D, Canoine V, Fusani L. Developmental exposure to xenoestrogen enhances spatial learning in male rats. Horm Behav. 2007;51(5):620-5. [PubMed ID: 17428485]. https://doi.org/10.1016/j.yhbeh.2007.03.002.
-
6.
Whiting PJ. GABA-A receptor subtypes in the brain: a paradigm for CNS drug discovery? Drug Discov Today. 2003;8(10):445-50. [PubMed ID: 12801796].
-
7.
McCabe BJ, Horn G, Kendrick KM. GABA, taurine and learning: release of amino acids from slices of chick brain following filial imprinting. Neuroscience. 2001;105(2):317-24. [PubMed ID: 11672599].
-
8.
Wieland HA, Luddens H, Seeburg PH. A single histidine in GABAA receptors is essential for benzodiazepine agonist binding. J Biol Chem. 1992;267(3):1426-9. [PubMed ID: 1346133].
-
9.
Crestani F, Assandri R, Tauber M, Martin JR, Rudolph U. Contribution of the alpha1-GABA(A) receptor subtype to the pharmacological actions of benzodiazepine site inverse agonists. Neuropharmacology. 2002;43(4):679-84. [PubMed ID: 12367613].
-
10.
Savic MM, Obradovic DI, Ugresic ND, Cook JM, Yin W, Bokonjic DR. Bidirectional effects of benzodiazepine binding site ligands in the passive avoidance task: differential antagonism by flumazenil and beta-CCt. Behav Brain Res. 2005;158(2):293-300. [PubMed ID: 15698896]. https://doi.org/10.1016/j.bbr.2004.09.011.
-
11.
Choi IS, Cho JH, Park EJ, Park JW, Kim SH, Lee MG, et al. Multiple effects of bisphenol A, an endocrine disrupter, on GABA(A) receptors in acutely dissociated rat CA3 pyramidal neurons. Neurosci Res. 2007;59(1):8-17. [PubMed ID: 17574696]. https://doi.org/10.1016/j.neures.2007.05.003.
-
12.
Goncalves CR, Cunha RW, Barros DM, Martinez PE. Effects of prenatal and postnatal exposure to a low dose of bisphenol A on behavior and memory in rats. Environ Toxicol Pharmacol. 2010;30(2):195-201. [PubMed ID: 21787652]. https://doi.org/10.1016/j.etap.2010.06.003.
-
13.
Noriega NC, Eghlidi DH, Garyfallou VT, Kohama SG, Kryger SG, Urbanski HF. Influence of 17beta-estradiol and progesterone on GABAergic gene expression in the arcuate nucleus, amygdala and hippocampus of the rhesus macaque. Brain Res. 2010;1307:28-42. [PubMed ID: 19833106]. https://doi.org/10.1016/j.brainres.2009.10.011.
-
14.
Zheng G, Zhang X, Chen Y, Zhang Y, Luo W, Chen J. Evidence for a role of GABAA receptor in the acute restraint stress-induced enhancement of spatial memory. Brain Res. 2007;1181:61-73. [PubMed ID: 17916335]. https://doi.org/10.1016/j.brainres.2007.08.077.
-
15.
Lech M, Skibinska A, Kossut M. Delayed upregulation of GABAA alpha1 receptor subunit mRNA in somatosensory cortex of mice following learning-dependent plasticity of cortical representations. Mol brain Res. 2001;96:82-6.