Abstract
Context:
Osteopontin (OPN) is a matrix phosphoprotein expressed by a variety of tissues and cells, including the immune system and the nervous system. Previous studies have shown that OPN may have a role in neurodegenerative diseases, including multiple sclerosis, Parkinson’s disease, and Alzheimer’s disease.Objectives:
The present study aimed to systematically review studies investigating the role of OPN in amyotrophic lateral sclerosis (ALS) patients or the disease animal model.Evidence Acquisition:
We searched the Cochrane Library, PubMed, Web of Science, and Scopus to find relevant articles published up to January 20, 2019. Both human and animal model studies of ALS were considered.Results:
A total of nine articles (four human studies and five animal model studies) were included. Two of the human studies reported that the CSF levels of OPN were higher among ALS patients compared to controls. The other two human studies found that OPN levels in cortical neurons did not differ significantly between ALS cases and the non-neurological control group. One of the studies found that the expression level of OPN in astrocytes was similar between ALS patients and the control group, but the level of microglial OPN significantly increased in ALS cases. Four of the animal model studies reported that the expression of OPN mRNA in spinal cord microglia significantly increased during the disease progression. The remaining animal model study found that OPN was selectively expressed by fast fatigue-resistant and slow motor neurons (MNs), which are resistant to ALS, and that the OPN expression was low among fast-fatigable MNs.Conclusions:
Prompt microglial activation is a hallmark pathology of ALS, and OPN is among the most widely expressed proteins by these activated glial cells. Therefore, OPN might have a role in ALS pathogenesis. The existing evidence is not sufficient to justify whether OPN has a neurotoxic or neuroprotective role in ALS. We encourage researchers to investigate the role of OPN in ALS pathogenesis more extensively.Keywords
Amyotrophic Lateral Sclerosis Osteopontin SPP1 Systematic Review
1. Context
Amyotrophic lateral sclerosis (ALS) is a devastating, progressive neurodegenerative disease that causes muscle weakness, atrophy, spasticity, and eventually death. Degeneration of both upper motor neurons and lower motor neurons with astrogliosis and microgliosis is the pathological hallmark of ALS (1). The exact cause of ALS is still unknown. Based on the available evidence, genetic factors, oxidative stress, mitochondrial dysfunction, neurofilament dysfunction, excitotoxicity, apoptosis, and proinflammatory cytokines contribute to ALS pathogenesis (2).
Growing evidence indicates that neuroinflammation plays an important role in the pathogenesis of neurodegenerative diseases, including ALS (3-7). Activated glial cells, particularly microglia and astrocytes, and T lymphocytes are the major cell populations involved in neuroinflammation (3, 8).
Osteopontin (OPN) is a matrix phosphoprotein expressed by a variety of tissues and cells, including the immune system and the nervous system. Immune cells, such as macrophages and T lymphocytes, are important sources of OPN during inflammatory processes. Previous studies have shown that OPN may have a role in neurodegenerative diseases, including multiple sclerosis (MS), Parkinson’s disease, Alzheimer’s disease, and frontotemporal dementia (9, 10). It was reported that OPN was selectively expressed in alpha motor neurons (α-MNs), which are the most vulnerable neurons in ALS (11, 12). Therefore, it may also play a role in the pathogenesis of ALS.
2. Objectives
Few studies have evaluated the OPN expression in ALS patients or the ALS experimental model and compared them with controls. Here, we aimed to systematically review studies investigating the role of OPN in ALS or its animal model.
3. Search Strategy
We searched the Cochrane Library, PubMed, Web of Science, and Scopus to find relevant articles published up to January 20, 2019. The searched terms used in this study were: (ALS OR MND OR motor-neuron-disease OR motor-neurone-disease OR motor-neurone-diseases OR motor-neuron-diseases OR amyotrophic-lateral-sclerosis OR progressive muscular atrophy OR progressive-spinal-muscular-atrophy OR primary-lateral-sclerosis OR motor neuronopath* OR motor neuronopathy OR lou-gehrig's-disease) AND (“osteopontin” OR “OPN” OR “bone sialoprotein I” OR “BSP-1” OR “BSP 1” OR “BSP1” OR “BSPI” OR “BNSP” OR “early T-lymphocyte activation” OR “ETA-1” OR “ETA 1” OR “ETA1” OR “ETAI” OR “secreted phosphoprotein 1” OR “SPP-1” OR “SPP 1” OR “SPP1” OR “SPPI” OR “Rickettsia resistance” OR “Ric”). Moreover, we manually screened the reference lists of the included studies and related reviews, and tracked studies that had cited them through the Google Scholar to find eligible articles. Only articles published in English were included. This paper was written according to the preferred reporting items for systematic reviews and meta-analyses (PRISMA) guidelines.
4. Study Selection and Data Extraction
Both human and animal model studies of ALS were considered. The exclusion criteria were: (1) studies on animals other than rodents; (2) studies classified as case reports, case series (if included less than five patients), narrative reviews, systematic reviews, expert opinions, and educational reports, or conference abstracts; and (3) in vitro studies. The following data were extracted from the included studies: first author and year of publication, location, study design, study population characteristics, specimen, method, endpoints, and relevant main findings. The quality of the included human studies was assessed using the Newcastle-Ottawa scale (13). Moreover, the quality of the animal model studies was evaluated using the systematic review center for laboratory animal experimentation (SYRCLE) risk of bias tool (14).
5. Results
Overall, 85 unique articles were retrieved, of which six were selected for full-text eligibility assessment. One study was excluded (had not evaluated osteopontin in ALS patients), and four additional articles were identified via reference screening. Finally, nine articles were included (Figure 1). No study was excluded because of poor quality (Supplementary file appendices). The summary of the included studies is shown in Table 1 and Table 2.
Summary of Human Studies of OPN in ALS
Study | Specimen | ALS | Control | Method | Endpoints | Findings | ||||
---|---|---|---|---|---|---|---|---|---|---|
Type (N) | Age | Gender (M/F) | Type (N) | Age | Gender (M/F) | |||||
Von Neuhoff et. al. 2012 (15) | CSF | Sporadic (23); Sporadic (35) | 55.9 ± 10.3; 59 ± 12.8 | (16/7); (18/17) | Neurological diseases (23) | 55 ± 11.1; 62.4 ± 12.2 | (14/9); (11/12) | ELISA; MS | Identification of disease-specific CSF biomarkers. | OPN levels were higher in ALS-CSF compared to the control CSF, but the results were not statistically significant. |
Varghese et al. 2013 (16) | CSF | Sporadic (16); Sporadic (10) | 47.4 ± 5.4; 47.4 ± 5 | (10/6); (7/3) | Normal (13); Normal (10) | 45.7 ± 7; 47.3 ± 7 | (11/2); (8/2) | ELISA; MS | Identify the toxic factors in ALS-CSF | 1.9-fold increased OPN levels were observed in ALS-CSF compared to the control CSF; Compared to controls, OPN was upregulated 3-fold in ALS-CSF. |
Silva et. al. 2015 (17) | Tissue (occipital lobe) | Sporadic, 5 | 50.4 ± 7.4 | (3/2) | Normal (9); HIV-ANI (5); HIV-NMD/HAD (5) | 47.7 ± 7.4; 43.4 ± 7.9; 40.5 ± 10.5 | (4/5); (4/1); - | IHC | Determine the level of OPN in neurons, astrocytes, and microglia. | In astrocytes, OPN levels did not differ significantly between the ALS group and normal controls or the HIV-ANI group. However, OPN was significantly elevated in HIV-MND/HAD patients compared to ALS cases; Compared to normal controls, OPN levels in microglia significantly increased in ALS cases, but there was no significant difference between the ALS and HIV groups; OPN levels in cortical neurons did not differ significantly between ALS cases and normal controls or HIV-MND/HAD patients, but they were significantly lower compared to HIV-ANI cases. |
Yamamoto et al. 2017 (12) | Tissue (brain and spinal cord) | 7 | 56 - 83 | (5/2) | Normal, 7 | 59-92 | (6/1) | IHC | To determine the OPN expression pattern in the human spinal cord, the primary sensory and motor cortex; To examine how the OPN expression was altered in ALS subjects | In normal subjects, OPN-positive neurons with high immunoreactivity were observed in the spinal ventral horn and pyramidal neurons in the primary motor cortex; The intensity of OPN-positive neurons in the primary motor cortex was not significantly different between ALS patients and normal controls; In the spinal ventral horn of ALS patients, the ratio of OPN-positive neurons was significantly reduced compared to healthy subjects; Compared to controls, the intensity of OPN-immunoreactivity was significantly reduced in large neurons in both the primary motor cortex and the spinal ventral horn. |
Summary of ALS Animal Model Studies of OPN
Study | Animal Model | Intervention | Endpoints | Findings | ||
---|---|---|---|---|---|---|
Type | Target | Method | ||||
Chiu et. al. 2008 (18) | SOD1G93A, SOD1WT and non-Tg mice | Gene expression | Microglia purified from the spinal cord | Real-time PCR | Differences in gene expression during disease progression | In SOD1G93A mice, OPN was upregulated 36.65 fold during the early symptomatic and 67.23 fold during late symptomatic phases; Compared to controls, the OPN expression significantly increased during presymptomatic, early symptomatic, and end-stage phases. |
Chiu et al. 2013 (19) | SOD1G93A, SOD1WT, and non-Tg mice | Gene expression | Microglia purified from the spinal cord | Real-time PCR | Differences in gene expression during disease progression | Compared to controls, the OPN expression significantly increased during the early symptomatic and end-stage phases. |
Nikodemova et. al. 2014 (20) | SOD1G93A and SOD1WT female offsprings | Gene expression | Microglia purified from the brain stem, spinal cord, and cortex | Real-time PCR | Differences in gene expression during disease progression | Compared to controls, the OPN expression significantly increased at disease onset and end-stage disease; At end-stage disease, there was no significant difference between wild-type and SOD1G93A animals in the mRNA levels of OPN in cortical microglia. |
Noristani et. al. 2015 (21) | SOD1G93A | Gene expression | Microglia purified from the spinal cord in the lumbar segment | Real-time PCR | Differences in gene expression during disease progression | OPN was upregulated 16.8 fold during the early symptomatic stage |
Morisaki et. al. 2016 (22) | SOD1G93A, SOD1WT | Gene and protein expression | Spinal cord tissue | Immunostaining; Western blot; Real-time PCR | The OPN expression in alpha motor neurons (α-MNs); The OPN expression during disease progression; Disease onset and progression in SOD1G93A/OPN-/- and SOD1G93A/OPN+/- mice compared to SOD1G93A/OPN+/+ mice. | The OPN expression was high among ALS-resistant MNs (FR and S MNs), but low in FF MNs. (FR and S: OPN-high/MMP9-low, FF: OPN-low/MMP9-high); Remodeled FR or S MNs (OPN-high/MMP9-high) appear during disease progression to compensate for FF MNs loss; Extracellular OPN-positive granules accumulate in the spinal cords of ALS mice during disease progression. A majority of these granules were detected within or attached to Iba1-positive microglia/macrophages; Compared to SOD1G93A/OPN+/+ and SOD1G93A/OPN+/- mice, delayed disease onset and significantly accelerated disease progression was observed in SOD1G93A/OPN-/- mice. |
The PRISMA flow diagram
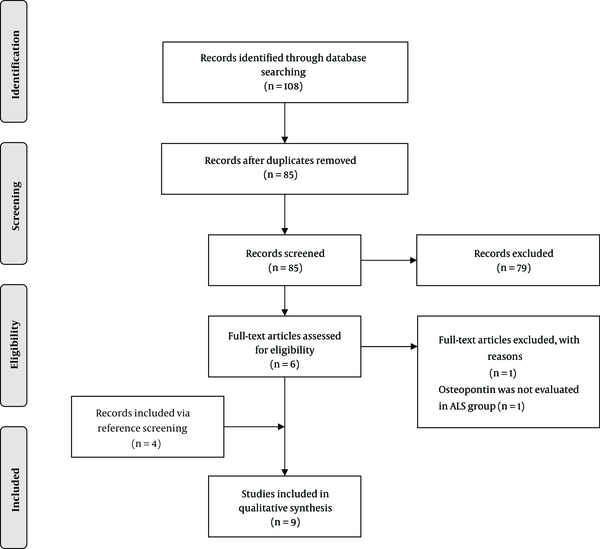
5.1. Human Studies
Four human studies examining OPN in ALS patients and controls were identified (Table 1) (12, 15-17). Two of the human studies measured the level of OPN in the CSF of sporadic ALS patients and controls (15, 16). Both studies reported that the CSF levels of OPN were higher among ALS patients compared to controls. The control group in von Neuhoff et al.’s study included patients with different neurological disorders (15), while none of the control subjects in Varghese et al.’s study had a history of neurological problems (16).
The other two human studies examined tissue samples of the central nervous system with the IHC method (12, 17). Both of these studies found that OPN levels in cortical neurons did not differ significantly between ALS cases and the non-neurological control group (12, 17). Silva et al. found that the expression level of OPN in astrocytes was similar between ALS patients and the control group, but the level of microglial OPN significantly increased in ALS cases (17). Yamamoto et al. investigated the OPN expression in spinal cord neurons and found the ratio of OPN-positive neurons to be significantly reduced in samples from ALS subjects (12).
5.2. Animal Model Studies
OPN was evaluated in five ALS animal model studies (18-22). Four of the studies investigated the OPN expression in spinal cord microglia, of which one also examined the microglial OPN expression in the brain stem and the cortex tissue (Table 2). All four studies reported that the expression of OPN mRNA significantly increased during the disease progression. One of the studies found an increased OPN expression during presymptomatic, early symptomatic, and end-stage disease (18). However, the other three studies reported an increased OPN expression only during symptomatic and end-stage disease (19-21). In Nikodemova et al.’s study (20), the OPN expression in cortical microglia was not significantly different from that in wild-type control mice.
One of the studies examined the OPN expression in the spinal cord tissue of mice, focusing on the expression status of α-MNs (22). The authors found that OPN was selectively expressed by fast fatigue-resistant (FR) and slow (S) MNs, which are resistant to ALS (22). On the other hand, the OPN expression was low among fast-fatigable (FF) MNs (22). Moreover, they realized that extracellular OPN-positive granules gradually began to appear around the time of disease onset, and the number of these extracellular OPN deposits increased significantly at end-stage disease (22). The majority of these granules were detected within or attached to spinal cord microglia (22). They also ablated the OPN gene in ALS mice models to examine whether the role of OPN was neuroprotective or neurotoxic. The authors found that compared to SOD1G93A/OPN+/+ and SOD1G93A/OPN+/- mice, disease onset was delayed while disease progression was accelerated in SOD1G93A/OPN-/- mice (22).
6. Conclusions
Here, we systematically reviewed in vivo studies investigating OPN changes in ALS patients or animal models. Four human and five animal model studies met the inclusion criteria (12, 15-22). Eight of the papers were published after 2012 (12, 15-17, 19-22), and the remaining one was published in 2008 (18). The OPN expression was examined in CSF, glial cells (microglia and astrocytes), and neurons (cortical and spinal cord).
Two of the human studies found higher levels of OPN in the CSF of ALS patients compared to controls (15, 16). The other two human studies indicated that OPN levels remained unchanged in cortical neurons (12, 17). Yamamoto et al. found that OPN-immunoreactivity in each neuron was significantly reduced in both the primary motor cortex and the spinal cord of ALS patients (12). One of the studies found that compared to normal controls, the intensity of OPN-positive neurons was significantly reduced in the spinal ventral horn of ALS patients (12). Another one of the studies reported that microglial OPN levels significantly increased in brain tissues from ALS patients compared to normal samples (17). The same study did not find any significant difference between ALS patients and controls regarding the OPN level in astrocytes (17).
All the animal model studies focused on gene expression differences between SOD1G93A mice and controls (18-22). Three of the animal model studies reported that the level of the OPN expression in spinal cord microglia was significantly higher in SOD1G93A mice than in controls (18-20). All the five animal model studies found that the OPN expression in spinal cord microglia of SOD1G93A mice significantly increased during disease progression (18-22). One of the studies investigating the mRNA levels of OPN in cortical microglia found no significant difference between wild-type and SOD1G93A mice (20). One study had investigated the OPN expression in α-MNs and the role of the OPN gene in disease onset and progression (22). The authors found that the OPN expression was high among ALS-resistant MNs but low in FF MNs, which degenerate early in ALS (22). They also found that the ablation of the OPN gene in SOD1G93A mice delayed disease onset, but accelerated the disease progression and had a minimum impact on the survival rate (22).
Microglia are the resident macrophage cells of the central nervous system and are involved in various physiological and pathological conditions, including neurodegenerative diseases (23). Loss of motor neurons in ALS leads to the prompt activation of microglia and astrocytes (7). Activated microglia can promote neurodegeneration by the expression of reactive oxygen species and several proinflammatory cytokines (23). It can also exert neuroprotective effects by suppressing local inflammation and debris clearance, as well as by producing and releasing a plethora of trophic factors (24, 25).
Both human and animal model studies of ALS showed that compared to controls, OPN levels significantly increased in microglia of the disease group (17-21). An increased OPN expression in microglia can have both inflammatory and anti-inflammatory effects. In vitro studies demonstrated that OPN might promote the survival of microglia under stress conditions (26). OPN reduces the expression of inducible nitric oxide synthase and the NO release from microglia (26-28). It may also act as a T-helper type 1 cytokine and promote inflammation (29).
OPN is selectively expressed in large α-MNs, which are the most vulnerable neurons in ALS (11, 12, 22). Within α-MNs, the OPN expression is low among FF MNs, which degenerate early in ALS (22). Morisaki et al. (22) found that while disease onset was delayed in SOD1G93A/OPN-/- mice, disease progression was significantly accelerated in them compared with SOD1G93A/OPN+/+ and SOD1G93A/OPN+/- mice. Yamamoto et al. (12) realized that within neurons, OPN-immunoreactivity was reduced considerably in MNs and pyramidal neurons of ALS patients compared to normal subjects. These findings indicate that the OPN expression in neurons may have neuroprotective properties. There is some evidence that OPN may enhance the survival and proliferation of neuronal cells (30-33).
Prompt microglial activation is a hallmark pathology of ALS, and OPN is among the most widely expressed proteins by these activated glial cells. Therefore, OPN might have a role in ALS pathogenesis. The existing evidence is not sufficient to justify whether OPN has a neurotoxic or neuroprotective role in ALS. We encourage researchers to investigate the role of OPN in ALS pathogenesis more extensively.
References
-
1.
Philips T, Rothstein JD. Glial cells in amyotrophic lateral sclerosis. Experimental neurology. 2014;262 Pt B:111-20. [PubMed ID: 24859452]. https://doi.org/10.1016/j.expneurol.2014.05.015.
-
2.
Peters OM, Ghasemi M, Brown RH Jr. Emerging mechanisms of molecular pathology in ALS. J Clin Invest. 2015;125(5):1767-79. [PubMed ID: 25932674]. [PubMed Central ID: PMCPmc4463186]. https://doi.org/10.1172/jci71601.
-
3.
Philips T, Robberecht W. Neuroinflammation in amyotrophic lateral sclerosis: role of glial activation in motor neuron disease. Lancet Neurol. 2011;10(3):253-63. [PubMed ID: 21349440]. https://doi.org/10.1016/s1474-4422(11)70015-1.
-
4.
Lyon MS, Wosiski-Kuhn M, Gillespie R, Caress J, Milligan C. Inflammation, Immunity, and amyotrophic lateral sclerosis: I. Etiology and pathology. Muscle Nerve. 2019;59(1):10-22. [PubMed ID: 29979464]. https://doi.org/10.1002/mus.26289.
-
5.
McCauley ME, Baloh RH. Inflammation in ALS/FTD pathogenesis. Acta Neuropathologica. 2019;137(5):715-30. https://doi.org/10.1007/s00401-018-1933-9.
-
6.
Morello G, Spampinato AG, Cavallaro S. Neuroinflammation and ALS: transcriptomic insights into molecular disease mechanisms and therapeutic targets. Mediators of inflammation. 2017;2017.
-
7.
McGeer PL, McGeer EG. Inflammatory processes in amyotrophic lateral sclerosis. Muscle Nerve. 2002;26(4):459-70. [PubMed ID: 12362410]. https://doi.org/10.1002/mus.10191.
-
8.
Malaspina A, Puentes F, Amor S. Disease origin and progression in amyotrophic lateral sclerosis: an immunology perspective. Int Immunol. 2015;27(3):117-29. [PubMed ID: 25344935]. https://doi.org/10.1093/intimm/dxu099.
-
9.
Agah E, Zardoui A, Saghazadeh A, Ahmadi M, Tafakhori A, Rezaei N. Osteopontin (OPN) as a CSF and blood biomarker for multiple sclerosis: A systematic review and meta-analysis. PLoS One. 2018;13(1). e0190252. [PubMed ID: 29346446]. [PubMed Central ID: PMCPmc5773083]. https://doi.org/10.1371/journal.pone.0190252.
-
10.
Carecchio M, Comi C. The role of osteopontin in neurodegenerative diseases. J Alzheimers Dis. 2011;25(2):179-85. [PubMed ID: 21358042]. https://doi.org/10.3233/jad-2011-102151.
-
11.
Misawa H, Hara M, Tanabe S, Niikura M, Moriwaki Y, Okuda T. Osteopontin is an alpha motor neuron marker in the mouse spinal cord. J Neurosci Res. 2012;90(4):732-42. [PubMed ID: 22420030]. https://doi.org/10.1002/jnr.22813.
-
12.
Yamamoto T, Murayama S, Takao M, Isa T, Higo N. Expression of secreted phosphoprotein 1 (osteopontin) in human sensorimotor cortex and spinal cord: Changes in patients with amyotrophic lateral sclerosis. Brain Res. 2017;1655:168-75. [PubMed ID: 27823929]. https://doi.org/10.1016/j.brainres.2016.10.030.
-
13.
Wells GA, Shea B, O'Connell D, Peterson J, Welch V, Losos M, et al. The Newcastle-Ottawa Scale (NOS) for assessing the quality of nonrandomised studies in meta-analyses. 2011.
-
14.
Hooijmans CR, Rovers MM, de Vries RB, Leenaars M, Ritskes-Hoitinga M, Langendam MW. SYRCLE's risk of bias tool for animal studies. BMC medical research methodology. 2014;14:43. [PubMed ID: 24667063]. https://doi.org/10.1186/1471-2288-14-43.
-
15.
von Neuhoff N, Oumeraci T, Wolf T, Kollewe K, Bewerunge P, Neumann B, et al. Monitoring CSF Proteome Alterations in Amyotrophic Lateral Sclerosis: Obstacles and Perspectives in Translating a Novel Marker Panel to the Clinic. PLoS ONE. 2012;7(9). https://doi.org/10.1371/journal.pone.0044401.
-
16.
Varghese AM, Sharma A, Mishra P, Vijayalakshmi K, Harsha HC, Sathyaprabha TN, et al. Chitotriosidase - a putative biomarker for sporadic amyotrophic lateral sclerosis. Clin Proteomics. 2013;10(1):19. [PubMed ID: 24295388]. [PubMed Central ID: PMCPmc4220794]. https://doi.org/10.1186/1559-0275-10-19.
-
17.
Silva K, Hope-Lucas C, White T, Hairston TK, Rameau T, Brown A. Cortical neurons are a prominent source of the proinflammatory cytokine osteopontin in HIV-associated neurocognitive disorders. J Neurovirol. 2015;21(2):174-85. [PubMed ID: 25636782]. [PubMed Central ID: PMCPmc4372685]. https://doi.org/10.1007/s13365-015-0317-3.
-
18.
Chiu IM, Chen A, Zheng Y, Kosaras B, Tsiftsoglou SA, Vartanian TK, et al. T lymphocytes potentiate endogenous neuroprotective inflammation in a mouse model of ALS. Proc Natl Acad Sci U S A. 2008;105(46):17913-8. [PubMed ID: 18997009]. [PubMed Central ID: PMCPmc2581614]. https://doi.org/10.1073/pnas.0804610105.
-
19.
Chiu I, Morimoto EA, Goodarzi H, Liao J, O’Keeffe S, Phatnani H, et al. A Neurodegeneration-Specific Gene-Expression Signature of Acutely Isolated Microglia from an Amyotrophic Lateral Sclerosis Mouse Model. Cell Reports. 2013;4(2):385-401. https://doi.org/10.1016/j.celrep.2013.06.018.
-
20.
Nikodemova M, Small AL, Smith SM, Mitchell GS, Watters JJ. Spinal but not cortical microglia acquire an atypical phenotype with high VEGF, galectin-3 and osteopontin, and blunted inflammatory responses in ALS rats. Neurobiol Dis. 2014;69:43-53. [PubMed ID: 24269728]. [PubMed Central ID: PMCPmc4079765]. https://doi.org/10.1016/j.nbd.2013.11.009.
-
21.
Noristani HN, Sabourin JC, Gerber YN, Teigell M, Sommacal A, dM Vivanco M, et al. Brca1 is expressed in human microglia and is dysregulated in human and animal model of ALS. Molecular Neurodegeneration. 2015;10(1):34. https://doi.org/10.1186/s13024-015-0023-x.
-
22.
Morisaki Y, Niikura M, Watanabe M, Onishi K, Tanabe S, Moriwaki Y, et al. Selective Expression of Osteopontin in ALS-resistant Motor Neurons is a Critical Determinant of Late Phase Neurodegeneration Mediated by Matrix Metalloproteinase-9. Sci Rep. 2016;6:27354. [PubMed ID: 27264390]. [PubMed Central ID: PMCPmc4893611]. https://doi.org/10.1038/srep27354.
-
23.
Hickman S, Izzy S, Sen P, Morsett L, El Khoury J. Microglia in neurodegeneration. Nature Neuroscience. 2018;21(10):1359-69. https://doi.org/10.1038/s41593-018-0242-x.
-
24.
Neumann H, Kotter MR, Franklin RJ. Debris clearance by microglia: an essential link between degeneration and regeneration. Brain. 2009;132(Pt 2):288-95. [PubMed ID: 18567623]. [PubMed Central ID: PMCPmc2640215]. https://doi.org/10.1093/brain/awn109.
-
25.
Kawabori M, Yenari MA. The role of the microglia in acute CNS injury. Metabolic brain disease. 2015;30(2):381-92. [PubMed ID: 24682762]. https://doi.org/10.1007/s11011-014-9531-6.
-
26.
Rabenstein M, Vay SU, Flitsch LJ, Fink GR, Schroeter M, Rueger MA. Osteopontin directly modulates cytokine expression of primary microglia and increases their survival. J Neuroimmunol. 2016;299:130-8. [PubMed ID: 27725111]. https://doi.org/10.1016/j.jneuroim.2016.09.009.
-
27.
Guo H, Cai CQ, Schroeder RA, Kuo PC. Osteopontin is a negative feedback regulator of nitric oxide synthesis in murine macrophages. J Immunol. 2001;166(2):1079-86. [PubMed ID: 11145688].
-
28.
Tambuyzer BR, Casteleyn C, Vergauwen H, Van Cruchten S, Van Ginneken C. Osteopontin alters the functional profile of porcine microglia in vitro. Cell Biol Int. 2012;36(12):1233-8. [PubMed ID: 22974008]. https://doi.org/10.1042/cbi20120172.
-
29.
Ashkar S, Weber GF, Panoutsakopoulou V, Sanchirico ME, Jansson M, Zawaideh S, et al. Eta-1 (osteopontin): an early component of type-1 (cell-mediated) immunity. Science. 2000;287(5454):860-4. [PubMed ID: 10657301].
-
30.
Rabenstein M, Hucklenbroich J, Willuweit A, Ladwig A, Fink GR, Schroeter M, et al. Osteopontin mediates survival, proliferation and migration of neural stem cells through the chemokine receptor CXCR4. Stem cell research & therapy. 2015;6(1):99. [PubMed ID: 25998490]. https://doi.org/10.1186/s13287-015-0098-x.
-
31.
Duan X, Qiao M, Bei F, Kim IJ, He Z, Sanes JR. Subtype-specific regeneration of retinal ganglion cells following axotomy: effects of osteopontin and mTOR signaling. Neuron. 2015;85(6):1244-56. [PubMed ID: 25754821]. [PubMed Central ID: PMCPmc4391013]. https://doi.org/10.1016/j.neuron.2015.02.017.
-
32.
Kalluri HS, Dempsey RJ. Osteopontin increases the proliferation of neural progenitor cells. International Journal of Developmental Neuroscience. 2012;30(5):359-62. https://doi.org/10.1016/j.ijdevneu.2012.04.003.
-
33.
Yan YP, Lang BT, Vemuganti R, Dempsey RJ. Osteopontin is a mediator of the lateral migration of neuroblasts from the subventricular zone after focal cerebral ischemia. Neurochem Int. 2009;55(8):826-32. [PubMed ID: 19686792]. https://doi.org/10.1016/j.neuint.2009.08.007.