Abstract
Background:
Nonencapsulated, nontypeable Haemophilus influenzae (NTHi) is considered an important cause of acute otitis in children and respiratory diseases among adults. The bacteria express several outer membrane proteins, some of which have been studied as vaccine candidates. Protein D is a highly conserved surface lipoprotein, which has been found in strains of both encapsulated and nonencapsulated H. influenzae.Objectives:
Opsonin and protective antibodies against protein D play major roles in the prevention of infections caused by NTHi. Since NTHi can be also an intracellular organism, it needs to be removed through immune-mediated mechanisms.Methods:
In this study, groups of BALB/c mice were subcutaneously immunized with recombinanat truncated D protein and an aluminum hydroxide (alum) adjuvant or protein D combined with an outer membrane vesicle (OMV) adjuvant from Neisseria meningitidis bacteria. Then, opsonophagocytic activities of antibodies were measured in serum samples collected on days 14, 28, and 42. The levels of interleukin-4 (IL-4), IL-10, and interferon-gamma were measured in splenocyte cultures of immunized mice.Results:
The opsonophagocytic activity of antibodies significantly increased in the immunized mice, compared to the control group, particularly on day 42. In addition, the secretion level of cytokines significantly increased in both groups of immunized mice, compared to the control group.Conclusions:
The results of this study demonstrated that recombinant truncated D protein can induce specific immune responses against nonencapsulated H. influenzae. It was suggested that the recombinant truncated D protein, as a vaccine candidate against the nonencapsulated strains of H. influenza, is essential in protection and development of sustainable immunity against infections caused by this bacterium.Keywords
Vaccine Opsonophagocytic Cytokine Assay Nontypeable Haemophilus influenzae
1. Background
Humoral immunity is the most basic protective immune response against extracellular bacteria to inhibit infections. Protective antibodies against outer membrane proteins and capsules play an important role in preventing local and general infections. Immunoglobulin G (IgG) antibodies against capsules can be actively transferred to the fetus through the placenta, thereby protecting the neonate for up to 6 months after birth against invasive infections caused by Haemophilus influenzae. After this age, the level of protective antibodies decreases sharply, and the neonate becomes more prone to invasive and general infections, particularly meningitis (1).
Specific antibody production is activated through a series of biochemical events inside T cells, particularly Th2, which play a major role in the production of antibodies (2, 3). Nonencapsulated H. influenzae can lead to the development of a variety of acute and chronic respiratory tract diseases, such as sinusitis, bronchitis, and pneumonia, besides otitis and conjunctivitis; it is responsible for 20% - 30% of otitis cases and probably a high percentage of recurrent cases (4-6). The initial vaccines against H. influenzae type b (Hib), which only contained polyribosylribitol phosphate (PRP), could not stimulate cell-mediated immunity and T lymphocytes due to their physical and chemical nature and were classified among antigen-independent T cells. Therefore, they exhibited no potential in immunizing infants against H. meningitis (7-9).
Haemophilus vaccines, based on Hib capsular polysaccharides, are effective in reducing meningitis caused by Hib, whereas they fail to protect against other serotypes of encapsulated and nonencapsulated H. influenzae strains. In addition, infectious diseases, caused by nonencapsulated H. influenzae strains, are also on the rise around the world; they are considered the most important human respiratory tract pathogens. On the other hand, vaccine development for nonencapsulated H. influenzae has been postponed due to the high genetic and antigenic heterogeneity among nonencapsulated H. influenza strains. Therefore, researchers are still at the stage of identification and verification of protective antigens (10-14).
Antigenic candidates suitable for vaccine production should be ideally superficial and capable of producing specific antibodies and even T cells in contact with the host immune system. Since the pathogenesis of H. influenzae begins with nasopharyngeal colonization and subsequently spreads to other parts of the middle ear and sinuses, we can prevent the onset of local infection by preventing the initial adhesion of bacteria to the surface of the host cell and ultimately inhibit their spread to other parts of the body (15).
Protein D is a membrane surface lipoprotein with a molecular weight of 42 kDa (16, 17). This protein is substantially hydrophilic and exhibits glycerophosphodiesterase activity (GlpQ), leading to the release of phosphorylcholines from the host epithelial cells and causing damage to the ciliated epithelial cells of the host (18-20). It can be found in all encapsulated and nonencapsulated strains of H. influenzae and is highly conserved antigenically.
Accordingly, protein D has been suggested as a candidate protein for the production of vaccines against infections, caused by H. influenzae strains due to its conserved antigenic properties, establishment on bacterial cell surface, and presence in both encapsulated and nonencapsulated strains (21-26). Therefore, considering the characteristics of protein D and need for the design of appropriate vaccines against nonencapsulated H. influenzae strains, we aimed to propose a recombinant truncated D protein, based on 100% conserved regions among nontypeable H. influenzae (NTHi) strains.
2. Objectives
The aim of the present study was to assess and compare responses induced by humoral and cellular immunity of the designed recombinant protein in the presence of 2 different adjuvants with different origins and to examine the protective level of these responses in an animal model of BALB/c mice.
3. Methods
3.1. Outer Membrane Vesicle (OMV) Extraction from Neisseria Meningitidis Serogroup B
The OMV was extracted, using ultracentrifugation. Briefly, the bacteria were grown in an enriched medium in a 5% CO2 atmosphere to plot the growth curve and identify the time of the subsequent logarithmic phase. Bacterial growth was terminated, and supernatant was separated in a centrifugation process and filtered. Then, the supernatant was exposed to ultrafiltration, and finally, concentration was determined via centrifugation at 150,000 g for 3 hours. The obtained sediment was homogenized in 3% sucrose solution.
3.2. Composition of OMV
The OMV protein content was measured using a Nanodrop spectrophotometer. In addition, SDS-PAGE on 12% gel was applied to obtain the protein pattern.
3.3. Electron Microscopy
Electron microscopy was applied for evaluating the stability and integrity of OMV after the extraction process. For this purpose, the prepared grids were sent to the institute of biochemistry and biophysics, faculty of Tehran, Iran, to obtain an electron micrograph (Zeiss electron microscope, model CEA902-A).
3.4. Immunization in the Animal Model
For this purpose, 50 female BALB/c mice were prepared by Pasteur Institute of Iran and divided into groups (10 mice per group). All mice were kept according to the guidelines for the care and use of laboratory animals under laboratory conditions. Three subcutaneous injections were done every 2 weeks, and blood samples were collected from the corner of the eye at 2 weeks after each injection in order to obtain serum samples; the sera were stored at -70°C. The first group received 50 µg of recombinant truncated D protein, along with an equal volume of industrial aluminum hydroxide (alum) adjuvant, while the second group received 25 µg of recombinant truncated D protein, mixed with 25 µg of OMV adjuvant with a bacterial origin. The third group received OMV, and the fourth and fifth groups as the controls received respectively the alum adjuvant alone and phosphate-buffered saline (PBS).
3.5. Levels of IgG Antibody and Its Subclasses (IgG1 and IgG2a)
The IgG antibody and its subclasses were produced, based on the ELISA method. For this purpose, sterile 96-well petri plates, coated by 10 mg of recombinant protein, were stored at fridge temperature overnight. After an interval, the plates were washed, and the blocking process was carried out by solutions containing 3% bovine serum albumin (BSA). Serial dilutions of antibodies were prepared according to the manufacturer’s protocols and incubated in the wells of plates for 2 hours at room temperature.
Following the washing process, a 1:6000 dilution of HRP-conjugated anti-mouse IgG and a 1:1000 dilution of HRP-conjugated anti-mouse isotype (IgG1 and IgG2a) were individually added to the wells and stored at room temperature for 2 hours. After a certain period, the plates were washed, and a chromogenic substrate was added to the wells; after the end of color reactions, the plates were read at a wavelength of 450 nm.
3.6. Assessment of Interleukin-10 (IL-10), IL-4, and Interferon-γ (INF-γ) Production in the Spleen Supernatant
Fourteen days after the injections, the spleen of mice was extracted. Under sterile conditions, 2 million cells were cultured in each well of sterile 12-well flat-bottom plates in RPMI-1640 medium, containing 10% fetal bovine serum (FBS), 1% Pen/Strep, and 10 mM HEPES. They were then stimulated for 72 hours by the recombinant protein D, recombinant protein D plus OMV, and OMV, respectively and incubated at 37°C in a 5% CO2 atmosphere. Also, 5 µg of concanavalin A (ConA) and medium alone were used as the negative and positive controls, respectively. The ELISA method was used to evaluate the levels of INF-γ, IL-10, and IL-4, based on the manufacturer’s instructions (eBioscience).
3.7. Opsonophagocytosis Assessment
In brief, to evaluate opsonophagocytosis, we used 2 × 107 CFU/mL of bacteria in an enrichment medium, containing 1% BSA and murine macrophages at a concentration of 2 × 107 cell/mL in a complete cell culture medium; finally, baby rabbit serum was produced as a source of external complement. After preparation of serial dilutions and inactivation at 56°C for 30 minutes, 100 µL of bacterial suspension, together with 100 µL of antibody serial dilutions, was incubated at 24 - 25°C for 90 minutes.
After washing, 100 µL of murine macrophages and 100 µL of rabbit complement were added to the mixture, cultured in 48-well plates, and incubated for 90 minutes at 27°C in a 5% CO2 atmosphere. Finally, t0 and t90 samples were cultivated on a chocolate agar medium overnight at 37°C in a 5% CO2 atmosphere. The serum samples of preimmune mice were used as the control. All the experiments were performed in duplicate. The rate of cell death was calculated using the following formula:

3.8. Statistical Analysis
Statistical analysis was carried out in Graphpad Prism (version 5), using t test and ANOVA test. The significance level was set at P < 0.05.
4. Results
4.1. Extraction of OMV from N. Meningitidis Serogroup B
The OMV protein level was estimated at 0.5 mg/mL, using the Nanodrop spectrophotometer. The electron microscopic images showed the integrity and uniformity of OMV structure with a size of 50 - 200 nm (Figure 1), which is consistent with previous studies.
The Scanning Electron Microscopic (SEM) Observation of the Outer Membrane Vesicles (OMV) Released from Neisseria Meningitidis
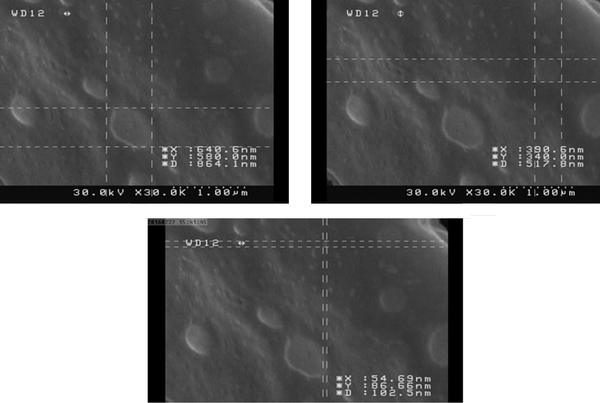
4.2. Evaluation of IgG and Its Subclasses Produced Against Recombinant Proteins In Immunized Mice
Immunization with recombinant truncated D protein combined with the alum adjuvant showed significantly higher levels of IgG, compared to the control group. The highest level of antibody production was observed in the second booster (third injection), which revealed significant differences with the first injection, as well as the first booster (second injection). In addition, immunization with recombinant truncated D protein, combined with OMV, showed high levels of IgG responses with a significant difference from the control group.
The greatest amount of antibody was reported in the second booster (third injection), which indicated significant differences with the control group and the first injection, although the increase in responses was not significantly different from the second injection (first booster) (Figure 2). Comparison of the injection groups also showed that the group receiving the recombinant truncated D protein with alum adjuvant exhibited a higher level of response, compared to the group receiving recombinant truncated D protein with OMV; the reported increase was significant.
Induction of Antitruncated Protein D IgG Responses in an Animal Model
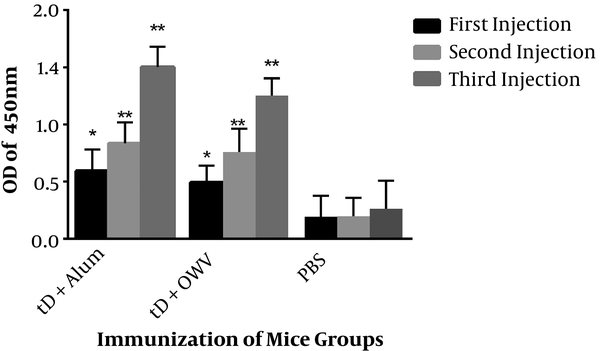
Since IgG1 and IgG2a humoral responses are indirect criteria associated with Th1 and Th2 immune responses, according to the assessment of IgG1 and IgG2a antibodies in each immunized mouse, IgG1 responses shifted towards IgG1 within 3 months after the last injection (second booster) in both groups. In both injection groups, IgG1 response was significantly higher than IgG2a response, representing a shift towards Th2 and humoral immunity. In addition, IgG1 titers in the first group showed a significant increase, compared to the second group. Although the level of IgG2a increased significantly compared to control group and a mixture of IgG1 and IgG2a responses were found, most responses were observed in IgG1 (Figure 3).
The IgG Subclass Antibody Responses Against Recombinant Truncated Protein D Were Determined by ELISA Assay of Individual Serum Samples Collected Over 6 Weeks
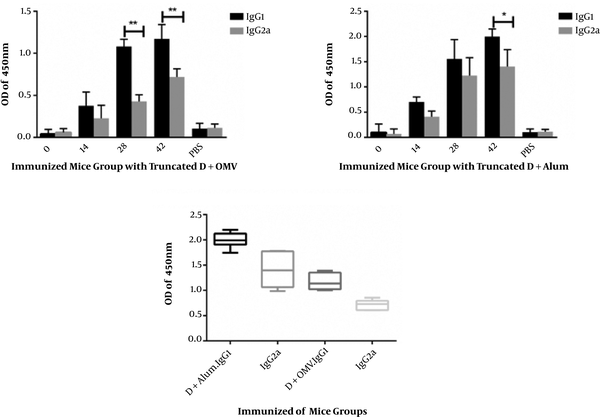
4.3. Induction of Th2 Humoral Responses via Immunization of Mice with the Fusion Protein
The levels of IFN-γ, IL-10, and IL-4 cytokines in the splenocyte supernatant were measured by ELISA method at 2 weeks after the third injection on day 42, followed by further stimulation with antigens for 3 days. Measurement of IFN-γ, secreted by splenocytes in the culture medium as an indicator of Th1 cell activity, showed that injection of recombinant truncated D protein with the alum adjuvant in the first group, as well as the mixture of recombinant truncated D protein and OMV adjuvant in the second group, led to an increase in IFN-γ, compared to the negative controls. Although the level of IFN-γ secretion was higher than the negative control group, it decreased in comparison with the positive controls (ConA antigen) (Figure 4).
The cytokine assay in the presence of truncated D protein and ConA
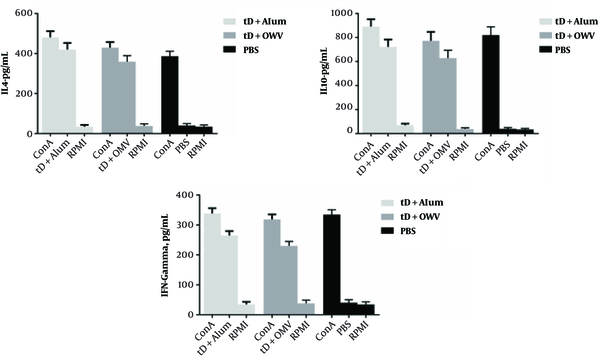
The IFN-γ level was significantly lower than IL-4 and IL-10 levels in both groups of mice, immunized with recombinant truncated D protein. On the other hand, measurement of IL-4 secreted by splenocytes in the culture, as an indicator of Th2 cell activity, revealed similar results to IFN-γ measurements, compared to the negative and positive control groups. Based on these results, the mice immunized with recombinant truncated D protein and alum adjuvant showed a significant increase in IL-4 level, compared to mice immunized with recombinant truncated D protein and OMV adjuvant.
As presented in Figure 4, production of IL-4 and IL-10 to IFN-γ significantly increased in both groups of mice immunized with recombinant truncated D protein. The level of IL-10, as an antiinflammatory and regulatory cytokine, which inhibits the secretion of cytokines such as IFNγ from Th1 lymphocytes, increased in the group of mice immunized with recombinant truncated D protein and alum adjuvant, compared to the negative control group and significantly decreased compared to the positive controls. The level of IL-10 significantly increased compared to IL-4 in both groups of mice immunized with recombinant truncated D protein, but declined in comparison with IFN-γ.
According to our observations, recombinant truncated D protein can stimulate immune responses and increase IFN-γ, IL-4, and IL-10 levels (Figure 4). Since all these cytokines were stimulated in response to recombinant truncated D protein and increased significantly in comparsion with the negative control group, it can be stated that a mixture of cellular (Th1) and humoral (Th2) immune responses was formed. However, the higher levels of IL-4 and IL-10 compared to IFN-γ switched immune responses towards Th2.
4.4. Opsonophagocytic Activity of Serum Antibodies Against Nonencapsulated H. Influenzae
The opsonophagocytic function of antibodies in the serum of mice from the first group (receiving recombinant truncated D protein with alum adjuvant) and the second group (receiving recombinant truncated D protein and OMV as a combined adjuvant) was determined against the standard strains of nonencapsulated H. influenzae bacteria. The greatest opsonophagocytic function of serum antibodies was observed at 2 weeks after the final injection (day 42) at 1:4 and 1:8 dilutions. Then, the sera of groups were compared at these dilutions, which indicated that the opsonophagocytic function reduced in 1:16 and 1:32 dilutions.
The number of viable bacteria decreased by more than 85% in the group receiving recombinant truncated D protein and alum adjuvant (first group) and declined by more than 70% in the group receiving adjuvant recombinant truncated D and OMV (second group). According to Figure 5, the opsonic activity of antibodies in the serum on day 42 (third serum) showed a significant increase in the first group, compared to the second group (P < 0.05). Nevertheless, there was no significant difference in the opsonophagocytic function of antibodies in the sera of day 14 (first serum) or day 28 (second serum) in the groups of immunized mice.
The Bactericidal Titers (BC50) are Expressed as the Reciprocal of the Greatest Serum Dilution Yielding ≥ 50% Bacterial Death, Compared to the Controls
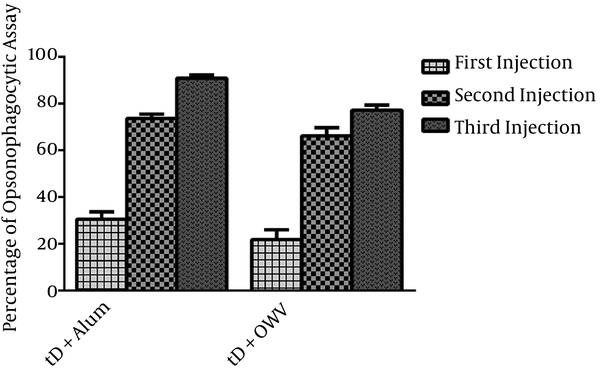
The results revealed that opsonin antibodies significantly increased after each injection of recombinant truncated D protein with different adjuvants in BALB/c mice, compared with the control group. As shown in Figure 5, the opsonophagocytic activity was associated with an increase from day 14 (first serum) to day 42 (third serum) in both groups of immunized mice (P < 0.05). In other words, there was a marked increase in the opsonophagocytic activity on day 42 (third serum) (Figure 5).
5. Discussion
Hib is the most prevalent cause of infection by the genus Haemophilus, which has a global spread and an endemic nature in most cases. It mainly affects children above 2 months, and invasive diseases caused by these bacteria occur in the age range of 3 months to 3 years (27). Although vaccination showed efficacy in 90% of children aged 18 months or above, it was abandoned in 1988 due to lack of immunity in children younger than 18 months, which is the age with the highest incidence of Hib infections (28, 29).
In 1985, Escola et al. introduced the first Hib PRP vaccine, conjugated with diphtheria toxoid, which was approved in the US and introduced to the market (30). During 1990 and 2001, PRP conjugated vaccines with tetanus toxoid (a protein carrier), CRM-197, and meningococcal OMV were also produced and confirmed. Among these vaccines, the conjugate vaccine, containing meningococcal OMV, showed favorable immunogenicity in infants aged 6 months to 2 years (31). However, these vaccines do not protect infants against the nonencapsulated strains; therefore, it is necessary to design an appropriate vaccine.
Development of a hapten-carrier conjugate is one of the main approaches in switching T cell-independent responses to T cell-dependent responses (10-14). Protein D is one of the factors, which contributes to the initial attachment of bacteria to human respiratory epithelial cells (32). Unlike other adhesion proteins of H. influenza, this conserved protein, with great antigenic properties, has the highest homology among all encapsulated and nonencapsulated strains of bacteria. Studies have shown that the NTHi mutant strain, which cannot express protein D, causes fewer infections than its wild type in animal models (23, 24, 26). Accordingly, protein D is essential as a virulence factor in NTHi. In this regard, Geme et al. in 1996 introduced Haemophilus surface fibril (Hsf), which is similar to Hia adhesion (33, 34).
In this study, we attempted to design a recombinant protein, based on 100% conserved regions and sequences among NTHi strains. The findings indicated that this candidate vaccine can induce an appropriate response from the immune system. Furthermore, the results confirmed a shift towards humoral immune responses. Mixed cellular and humoral immune responses were delineated, as expected based on previous studies. The properties of the designed protein, such as exposed surface and conservation degree, make it a suitable vaccine candidate.
In new vaccine-production technologies, which use recombinant proteins, most proteins in the purified form lose their ability to stimulate the immune response potential, and an adjuvant is necessary to boost immune responses. Alum is still recognized as a substance, which is widely used in combination with diphtheria and tetanus toxoids and is the most reliable adjuvant for human consumption (35). Since new processes leading to the stimulation of lymphocytes and formation of memory cells are recognized, considerable efforts have been made to produce better adjuvants, particularly for T cell-mediated immune responses. It should be noted that none of the new adjuvants have been approved for routine use in humans.
It has been recognized that most adjuvants act through mimicking PAMP molecules (36). The OMV of N. meningitidis is among the studied components with a microbial origin, which can be used as an adjuvant. Previous studies have shown that the outer membrane proteins of N. meningitidis are available in Cuban Meningococci B vaccines and have different potentials in inducing the initial immune system responses (37). Accordingly, in the present study, we aimed to use OMV from N. meningitidis bacteria as an adjuvant and to examine the induced immune responses.
OMVs are carriers of common virulence factors for each bacterium, secreted as a complex of proteins and lipids from the outer membrane (38). Therefore, they can stimulate toll-like receptors; this feature can justify the application of these agents as vaccines. Researchers have found that addition of OMVs to vaccines can increase their immunogenicity; in fact, OMV-based vaccines act as an alternative for the treatment of bacterial infections. The results of the present study showed that use of an alum adjuvant produces significantly better responses, compared with the use of an antigen in the presence of OMV; moreover, the results from the second group were significantly better than the control group.
5.1. Conclusion
In conclusion, it can be stated that the designed vaccine may have potential applications as a vaccine candidate. According to the assessments and the results, recombinant truncated D protein can be considered a new vaccine option and a potentially suitable candidate. However, as this candidate is an encapsulated strain, it is proposed to investigate the protective levels and antibody titers against the encapsulated strains in future studies.
Acknowledgements
References
-
1.
Kelly DF, Moxon ER, Pollard AJ. Haemophilus influenzae type b conjugate vaccines. Immunology. 2004;113(2):163-74. [PubMed ID: 15379976]. https://doi.org/10.1111/j.1365-2567.2004.01971.x.
-
2.
Falugi F, Petracca R, Mariani M, Luzzi E, Mancianti S, Carinci V, et al. Rationally designed strings of promiscuous CD4(+) T cell epitopes provide help to Haemophilus influenzae type b oligosaccharide: a model for new conjugate vaccines. Eur J Immunol. 2001;31(12):3816-24. [PubMed ID: 11745403]. https://doi.org/10.1002/1521-4141(200112)31:12<3816::AID-IMMU3816>3.0.CO;2-K.
-
3.
Rocha N, Neefjes J. MHC class II molecules on the move for successful antigen presentation. EMBO J. 2008;27(1):1-5. [PubMed ID: 18046453]. https://doi.org/10.1038/sj.emboj.7601945.
-
4.
Giebink GS. The microbiology of otitis media. Pediatr Infect Dis J. 1989;8(1 Suppl):S18-20. [PubMed ID: 2648288]. https://doi.org/10.1097/00006454-198901001-00008.
-
5.
Nelson JD. Changing trends in the microbiology and management of acute otitis media and sinusitis. Pediatr Infect Dis. 1986;5(6):749-53. [PubMed ID: 3797319].
-
6.
Krasan GP, Cutter D, Block SL, St Geme J3. Adhesin expression in matched nasopharyngeal and middle ear isolates of nontypeable Haemophilus influenzae from children with acute otitis media. Infect Immun. 1999;67(1):449-54. [PubMed ID: 9864255].
-
7.
Farley MM, Stephens DS, Brachman PJ, Harvey RC, Smith JD, Wenger JD. Invasive Haemophilus influenzae disease in adults. A prospective, population-based surveillance. CDC Meningitis Surveillance Group. Ann Intern Med. 1992;116(10):806-12. [PubMed ID: 1314530]. https://doi.org/10.7326/0003-4819-116-10-806.
-
8.
Mason EJ, Kaplan SL, Lamberth LB, Hinds DB, Kvernland SJ, Loiselle EM, et al. Serotype and ampicillin susceptibility of Haemophilus influenzae causing systemic infections in children: 3 years of experience. J Clin Microbiol. 1982;15(4):543-6. [PubMed ID: 6978349].
-
9.
Sansoni A, Rappuoli R, Viti S, Costantino P, Fanti O, Cellesi C. Immunity to Haemophilus influenzae type b on sample population from central Italy. Vaccine. 1992;10(9):627-30. [PubMed ID: 1502841]. https://doi.org/10.1016/0264-410X(92)90445-P.
-
10.
Berthet F, Prehn A, Suter R, Ethevenaux C, Seger RA. Protective immunogenicity of Haemophilus influenzae type b polysaccharide-tetanus protein conjugate vaccine in children who failed to respond to prior invasive H. influenzae type b disease. Pediatr Allergy Immunol. 1998;9(3):156-60. [PubMed ID: 9814731]. https://doi.org/10.1111/j.1399-3038.1998.tb00363.x.
-
11.
Greenberg DP, Vadheim CM, Bordenave N, Ziontz L, Christenson P, Waterman SH, et al. Protective efficacy of Haemophilus influenzae type b polysaccharide and conjugate vaccines in children 18 months of age and older. JAMA. 1991;265(8):987-92. [PubMed ID: 1992212].
-
12.
Heath PT, Bowen-Morris J, Griffiths D, Griffiths H, Crook DW, Moxon ER. Antibody persistence and Haemophilus influenzae type b carriage after infant immunisation with PRP-T. Arch Dis Child. 1997;77(6):488-92. [PubMed ID: 9496180]. https://doi.org/10.1136/adc.77.6.488.
-
13.
Mariotti S, Teloni R, von Hunolstein C, Romagnoli G, Orefici G, Nisini R. Immunogenicity of anti-Haemophilus influenzae type b CRM197 conjugate following mucosal vaccination with oligodeoxynucleotide containing immunostimulatory sequences as adjuvant. Vaccine. 2002;20(17-18):2229-39. [PubMed ID: 12009277]. https://doi.org/10.1016/S0264-410X(02)00113-5.
-
14.
Ward J, Brenneman G, Letson GW, Heyward WL. Limited efficacy of a Haemophilus influenzae type b conjugate vaccine in Alaska Native infants. The Alaska H. influenzae Vaccine Study Group. N Engl J Med. 1990;323(20):1393-401. [PubMed ID: 2233906]. https://doi.org/10.1056/NEJM199011153232006.
-
15.
Loeb MR, Smith DH. Outer membrane protein composition in disease isolates of Haemophilus influenzae: pathogenic and epidemiological implications. Infect Immun. 1980;30(3):709-17. [PubMed ID: 6971807].
-
16.
Janson H, Heden LO, Grubb A, Ruan MR, Forsgren A. Protein D, an immunoglobulin D-binding protein of Haemophilus influenzae: cloning, nucleotide sequence, and expression in Escherichia coli. Infect Immun. 1991;59(1):119-25. [PubMed ID: 1987023].
-
17.
Ruan MR, Akkoyunlu M, Grubb A, Forsgren A. Protein D of Haemophilus influenzae. A novel bacterial surface protein with affinity for human IgD. J Immunol. 1990;145(10):3379-84. [PubMed ID: 2230124].
-
18.
Larson TJ, Ehrmann M, Boos W. Periplasmic glycerophosphodiester phosphodiesterase of Escherichia coli, a new enzyme of the glp regulon. J Biol Chem. 1983;258(9):5428-32. [PubMed ID: 6304089].
-
19.
Tommassen J, Eiglmeier K, Cole ST, Overduin P, Larson TJ, Boos W. Characterization of two genes, glpQ and ugpQ, encoding glycerophosphoryl diester phosphodiesterases of Escherichia coli. Mol Gen Genet. 1991;226(1-2):321-7. [PubMed ID: 1851953]. https://doi.org/10.1007/BF00273621.
-
20.
Wu HC, Tokunaga M. Biogenesis of lipoproteins in bacteria. Curr Top Microbiol Immunol. 1986;125:127-57. [PubMed ID: 3527576]. https://doi.org/10.1007/978-3-642-71251-7_9.
-
21.
Ahren IL, Janson H, Forsgren A, Riesbeck K. Protein D expression promotes the adherence and internalization of non-typeable Haemophilus influenzae into human monocytic cells. Microb Pathog. 2001;31(3):151-8. [PubMed ID: 11500100]. https://doi.org/10.1006/mpat.2001.0456.
-
22.
Fan X, Goldfine H, Lysenko E, Weiser JN. The transfer of choline from the host to the bacterial cell surface requires glpQ in Haemophilus influenzae. Mol Microbiol. 2001;41(5):1029-36. [PubMed ID: 11555284]. https://doi.org/10.1046/j.1365-2958.2001.02571.x.
-
23.
Forsgren A, Riesbeck K, Janson H. Protein D of Haemophilus influenzae: a protective nontypeable H. influenzae antigen and a carrier for pneumococcal conjugate vaccines. Clin Infect Dis. 2008;46(5):726-31. [PubMed ID: 18230042]. https://doi.org/10.1086/527396.
-
24.
Janson H, Carl n B, Cervin A, Forsgren A, Magnusdottir AB, Lindberg S, et al. Effects on the ciliated epithelium of protein D-producing and -nonproducing nontypeable Haemophilus influenzae in nasopharyngeal tissue cultures. J Infect Dis. 1999;180(3):737-46. [PubMed ID: 10438362]. https://doi.org/10.1086/314921.
-
25.
Janson H, Melhus A, Hermansson A, Forsgren A. Protein D, the glycerophosphodiester phosphodiesterase from Haemophilus influenzae with affinity for human immunoglobulin D, influences virulence in a rat otitis model. Infect Immun. 1994;62(11):4848-54. [PubMed ID: 7927765].
-
26.
Munson RJ, Sasaki K. Protein D, a putative immunoglobulin D-binding protein produced by Haemophilus influenzae, is glycerophosphodiester phosphodiesterase. J Bacteriol. 1993;175(14):4569-71. [PubMed ID: 8392514]. https://doi.org/10.1128/jb.175.14.4569-4571.1993.
-
27.
Broome CV. Vaccine efficacy determination. NIPH Ann. 1991;14(2):219-21. discussion 222-4. [PubMed ID: 1812435].
-
28.
Broome CV, Breiman RF. Pneumococcal vaccine--past, present, and future. N Engl J Med. 1991;325(21):1506-8. [PubMed ID: 1944428]. https://doi.org/10.1056/NEJM199111213252109.
-
29.
Centers for Disease Control and Prevention (CDC). Progress toward eliminating Haemophilus influenzae type b disease among infants and children--United States, 1987-1997. MMWR Morb Mortal Wkly Rep. 1998;47(46):993-8. [PubMed ID: 9843325].
-
30.
Maroun JA. Haemophilus b conjugate vaccine. CMAJ. 1988;138(11):1031-2. [PubMed ID: 3370570].
-
31.
St Geme JW. Molecular and cellular determinants of non-typeable Haemophilus influenzae adherence and invasion. Cell Microbiol. 2002;4(4):191-200. [PubMed ID: 11952636]. https://doi.org/10.1046/j.1462-5822.2002.00180.x.
-
32.
Winter LE, Barenkamp SJ. Antibodies specific for the high-molecular-weight adhesion proteins of nontypeable Haemophilus influenzae are opsonophagocytic for both homologous and heterologous strains. Clin Vaccine Immunol. 2006;13(12):1333-42. [PubMed ID: 17021246]. https://doi.org/10.1128/CVI.00221-06.
-
33.
Prymula R, Peeters P, Chrobok V, Kriz P, Novakova E, Kaliskova E, et al. Pneumococcal capsular polysaccharides conjugated to protein D for prevention of acute otitis media caused by both Streptococcus pneumoniae and non-typable Haemophilus influenzae: a randomised double-blind efficacy study. Lancet. 2006;367(9512):740-8. [PubMed ID: 16517274]. https://doi.org/10.1016/S0140-6736(06)68304-9.
-
34.
Pichichero ME, Kaur R, Casey JR, Sabirov A, Khan MN, Almudevar A. Antibody response to Haemophilus influenzae outer membrane protein D, P6, and OMP26 after nasopharyngeal colonization and acute otitis media in children. Vaccine. 2010;28(44):7184-92. [PubMed ID: 20800701]. https://doi.org/10.1016/j.vaccine.2010.08.063.
-
35.
Clementi CF, Murphy TF. Non-typeable Haemophilus influenzae invasion and persistence in the human respiratory tract. Front Cell Infect Microbiol. 2011;1:1. [PubMed ID: 22919570]. https://doi.org/10.3389/fcimb.2011.00001.
-
36.
Dicko A, Santara G, Mahamar A, Sidibe Y, Barry A, Dicko Y, et al. Safety, reactogenicity and immunogenicity of a booster dose of the 10-valent pneumococcal non-typeable Haemophilus influenzae protein D conjugate vaccine (PHiD-CV) in Malian children. Hum Vaccin Immunother. 2013;9(2):382-8. [PubMed ID: 23291945]. https://doi.org/10.4161/hv.22692.
-
37.
Marciani DJ. Vaccine adjuvants: role and mechanisms of action in vaccine immunogenicity. Drug Discov Today. 2003;8(20):934-43. [PubMed ID: 14554157]. https://doi.org/10.1016/S1359-6446(03)02864-2.
-
38.
Huleatt JW, Jacobs AR, Tang J, Desai P, Kopp EB, Huang Y, et al. Vaccination with recombinant fusion proteins incorporating Toll-like receptor ligands induces rapid cellular and humoral immunity. Vaccine. 2007;25(4):763-75. [PubMed ID: 16968658]. https://doi.org/10.1016/j.vaccine.2006.08.013.