Abstract
Background:
Biofilm formation is a vital feature of the pathogenesis in filamentous fungi.Objectives:
Herein, we investigated in vitro antifungal pattern of biofilm of filamentous species keratitis isolates, and the effect of different concentrations of voriconazole on biofilm morphology changes using scanning electron microscopy.Methods:
Here 25 ocular fungal isolates were included (Fusarium solani; (n = 15) and Aspergillus flavus; (n = 10). We determined the biofilm formation of isolates in terms of their susceptibilities to different antifungals on sessile cells. This procedure was performed according to CLSI-M38-3rd. Biofilm morphology changes revealed with scanning electron microscopy (SEM).Results:
Twelve F. solani strains displayed the capacity to form biofilms from patients wearing contact lenses, while 8 A. flavus strains were recovered from cornea scrapings of trauma. Itraconazole, posaconazole and natamycin had the maximum activity against biofilms of all tested filamentous strains (MIC ranging from 0.031 - 0.25 µg/mL, 0.031 - 0.25 µg/mL and 2 - 4 µg/mL), respectively. Three F. solani and one A. flavus strains showed the high MIC values against voriconazole (MIC ≥ 1 µg/mL). Posaconazole revealed the lowest MIC values against biofilms of two strains (MIC ranging from 0.031 - 0.25 µg/mL); however, no significant difference was observed for itraconazole (P > 0.05). The efficacy of 16 µg/mL and 4 µg/mL of voriconazole was confirmed for biofilm of F. solani and A. flavus, respectively. The considerable changes in the morphologies of improved biofilms were seen by SEM.Conclusions:
For the successful treatment of fungal biofilm infections, it was necessary to have knowledge of the mechanism of action, penetration rates, and therapeutic concentrations of drugs.Keywords
1. Background
Corneal opacities, which tend to be caused by infectious keratitis, are the fourth common reason for blindness globally (1). Keratitis is a condition in which the cornea becomes inflamed (2). Fungal keratitis (FK) is an ocular infection that is responsible for 1 - 44% of all infectious keratitis cases, depending on the geographic region (3). The rising cases of FK in 2005, due to the growing number of contact lens wearers, highlighted clinical importance (4).
These patients may develop some severe complications, such as glaucoma, iris atrophy, cataract, cornea perforation, and endophthalmitis (5). In addition to the clinical and paraclinical diagnosis challenges, the management of FK is also difficult (5). Fusarium species (spp.) and Aspergillus spp. are the most common etiological agents of fungal keratitis (6).
Biofilm formation is a vital feature of the pathogenesis, especially in filamentous (7). Fungal cells in biofilms provide such advantages as increased resistance to stresses, protection, and metabolic interaction among the cells (8). Infections related to biofilm development have been reported to be severe clinical challenges (8).
2. Objectives
This research was designed to (A) in vitro antifungal pattern of biofilm of both Fusarium and Aspergillus species keratitis isolates, and (B) the effect of different concentrations of voriconazole (VCZ) on biofilm morphology changes using scanning electron microscopy (SEM).
3. Methods
3.1. Strains Source and Primary Identification
Twenty five ocular fungal isolates were restored from a previously conducted study, including 10 archived Aspergillus flavus isolates and 15 archived Fusarium solani isolates from patients wearing cornea scrapings of trauma and contact lens, respectively. The molecular identification and differentiation of isolates were proved by PCR amplification of translation elongation factor (TEF)-1α (TEF- 1α forward: GTAAGGAGGACAAGACTCACC; TEF- 1α reverse: GTAAGGAGGACAAGACTCACC) and ITS1-5.8S rDNA-ITS2 regions (ITS1-forward: 5’-TCCGTAGGTGAACCTGCGG-3’ and ITS4-reverse: 5’-TCCTCCGCTTAT TGATATGC-3’), respectively (8).
3.2. In vitro Biofilm Formation
Each strain was primarily inoculated onto Sabouraud Dextrose Agar (SDA, Merck, Germany) at room temperature for 4 - 7 days.
As described previously, the biofilm-formation ability of the strains was replicated twice using the crystal violet staining method (9). Briefly, the conidial suspension was adjusted to 1 × 106 CFU/mL in phosphate-buffered saline (PBS). Afterward, 100 µL of any suspension was dropped in a tube containing 1.5 mL of Sabouraud Dextrose Broth (SDB, Merck, Germany) and were incubated for 24 h at 37°C. Subsequently, 200 µL aliquot of diluted suspension (ratio 1:20 in SDB), was inserted to wells of 96-well microtiter plate (Roskilde, Denmark). After incubation of microplate for 24 h at 37°C, it was washed with PBS (3 times) and reversed to drain. At the next stage, 200 µL of crystal violet (1%) was added to each well. After incubation for 15 min at 25°C, the microplate was again rinsed thrice with PBS. Finally, 200 µL of a mixture (ethanol: acetone; 80:20 w/v) was added to each well. The percent transmittance (%T) value (450 nm) was read with a plate reader (Bio-Rad, USA). The biofilm production was scored as + (%Tbloc 5 - 20), ++ (%Tbloc 20 - 50), +++ (%Tbloc > 50) and negative (%Tbloc < 5). Candida parapsilosis (ATCC 22019) with %Tbloc 5 - 20 was regard as a positive quality control (9).
3.3. Determination of Minimum Inhibitory Concentration
Herein, antifungal agents consisted of itraconazole (ICZ), posaconazole (PCZ), and voriconazole (VCZ), natamycin (NAT). All antifungal agents were purchase from Sigma-Aldrich, USA. In vitro susceptibility of the sessile cells was tested according to CLSI-M38-3rd ed (10, 11). The MIC50 and MIC90 values were defined as the MICs required to inhibit the growth of 50% and 90% of the isolates, respectively (10).
To investigate the antifungals agents on biofilms, first, the existence of F. solani and A. flavus biofilms were confirmed on the disks in microplates (11). So, volume of 200 µL of the prepared fungal suspensions (1×106 CFU/mL in PBS) was added and incubated at 37°C for 24 h.
Then PBS was used for removing non-adherent cells. 200 µL of RPMI 1640 medium, containing different antifungal concentrations, was added and incubated at 37°C for 48 h. Dilutions ranging of drugs from 4 to 0.0312 µg/mL were tested. The negative control included a well containing 200 µL of RPMI (11).
The effects of antifungals agents on the in vitro biofilms were determined with MTT (3-[4,5-dimethylthiazol-2-yl]-2,5 diphenyl tetrazolium bromide) assay (Sigma-Aldrich, USA), as previously described (12). 25 µL of RPMI containing MTT (5 mg/mL) was added to each well and incubated at 37°C for 3 h. The content of each well was removed and then 200 mL of isopropanol was added to remove the MTT. The optical density (OD), after a 30-min incubation at 25°C, was measured with a spectrophotometer (PD-303, Netherlands) (12).
3.4. Scanning Electron Microscopy
The morphological changes of strains in the biofilm structure were observed with scanning electron microscope (AIS2100, Seron Technology, South Korea) after exposed to different VCZ concentrations.
All strain biofilms were grown on disks in 12-well microplates for 24 h. After washing with PBS, different VCZ concentrations (1, 4, and 16 µg of VOR/mL of RPMI 1640) were added to disks in well microplates and incubated at 35°C for 48 h. The VCZ concentrations were chosen based on the MIC range results obtained in the susceptibility testing assays. Untreated samples (without drug), as a control, were incubated in RPMI 1640 without VCZ. Then disks were washed with PBS and fixed in 2.5% (v/v) glutaraldehyde (100 mM phosphate buffer solution, pH 7.2) for 3 hours, followed by 1% (w/v) osmium tetroxide for 1 hour. Dehydration of the agar blocks was performed by a graded series of ethanol (30, 50, 60, 70, 80, 90, 95, and 100%; for 15 minutes) and ethanol: isoamyl acetate (3:1, 1:1, 1:3, and 100% isoamyl acetate twice for 30 minutes). The agar blocks were dried with liquid CO2 in a critical-point dryer (Sc7620, Emitech, England) and coated with a gold-coater for 5 minutes. Finally, the coated disks were imagined with an accelerating voltage of 9 kV (13).
3.5. Statistical Analysis
The data were analysed in GraphPad Prism Software (version 8.3.0), using two-tailed test. P-values of ≤ 0.05 were considered significant.
4. Results
4.1. Biofilm Formation
Out of 25 tested isolates, 12 F. solani and 8 A. flavus strains displayed the capacity to form biofilms as +++ (%Tbloc > 50).
4.2. Antifungal Susceptibility Testing
The MIC value (in µg/mL) of filamentous strain biofilms to antifungal agents are shown in Table 1. ICZ, PCZ and NAT had the maximum activity against biofilms of all tested filamentous strains (MIC ranging from 0.031 - 0.25 µg/mL, 0.031 - 0.25 µg/mL and 2 - 4 µg/mL), respectively. As Table 1 summarizes, PCZ showed the lowest MIC values against biofilms of all strains (MIC ranging from 0.031-0.25 µg/mL); however, no significant difference was observed for ICZ in this regard (P > 0.05). However, they tended to be less susceptible to VOR than to the other agents. Three F. solani and one A. flavus strains showed the high MIC values against VCZ (MIC ≥ 1).
Minimum Inhibitory Concentration (µg/mL) of Antifungal Agents Against Fusarium solani (N = 12) and Aspergillus flavus (N = 8) Biofilms a
Isolates | ||
---|---|---|
F. solani | A. flavus | |
Natamycin | ||
MIC range | 2 - 4 | 2 - 4 |
≤ 16 µg/mL | 12 (100) | 8 (100) |
≥ 32 µg/mL | - | - |
MIC50 | 4 | 2 |
MIC90 | 4 | 4 |
Itraconazole | ||
MIC range | 0.125 - 0.25 | 0.125 - 0.25 |
≤ 0.25 µg/mL | 12 (100) | 8 (100) |
≥ 0.5 µg/mL | - | - |
MIC50 | 0.125 | 0.125 |
MIC90 | 0.25 | 0.25 |
Voriconazole | ||
MIC range | 0.25 - 4 | 0.125 - 1 |
≤ 0.5 µg/mL | 9 (75) | 7 (87.5) |
≥ 1 µg/mL | 3 (25) | 1 (12.5) |
MIC50 | 0.5 | 0.5 |
MIC90 | 2 | 0.5 |
Posaconazole | ||
MIC range | 0.125 - 0.25 | 0.031 - 0.25 |
≤ 0.5 µg/mL | 12 (100) | 8 (100) |
≥ 1 µg/mL | - | - |
MIC50 | 0.125 | 0.062 |
MIC90 | 0.25 | 0.25 |
4.3. Biofilms by SEM
The disks were exposed to three different VCZ concentrations (1, 4, and 16 µg/mL) and compared to the controls.
Figures 1 and 2 show SEM pictures of biofilms formed by F. solani and A. flavus, respectively. The biofilm architecture of the F. solani control (untreated) was highly mixed, comprised of a compact layer of yeasts, pseudo hyphae, and hyphal forms. A few typical microconidia, fusion of abundant hyphae with a well-defined septum, and macroconidia containing new conidia aggregated and associated with apical growth are depicted in Figure 1A. After exposure to VCZ, biofilms of F. solani showed fewer conidia, most of the hyphae appeared to be thinner after the 1µg/mL treatment (Figure 1B). Lesser extent hyphae were also observed after the 4µg/mL treatment (Figure 1C). Biofilms exposed to 16 µg/mL VCZ contained substantially fewer conidia (i.e., they were smaller than those observed at 1 and 4 µg/mL) than untreated biofilms; however, seemed completely affected by VCZ treatment (Figure 1D).
Scanning electron micrographs of preformed Fusarium solani biofilms in the presence of different voriconazole concentrations. (A) F. solani biofilm control (without drug); (B through D) F. solani biofilms after exposure to voriconazole at 1 µg/mL, 4 µg/mL, and 16 µg/mL, respectively (1000 and 5000×magnification). (E) Macroscopic and (F) Microscopic observation.
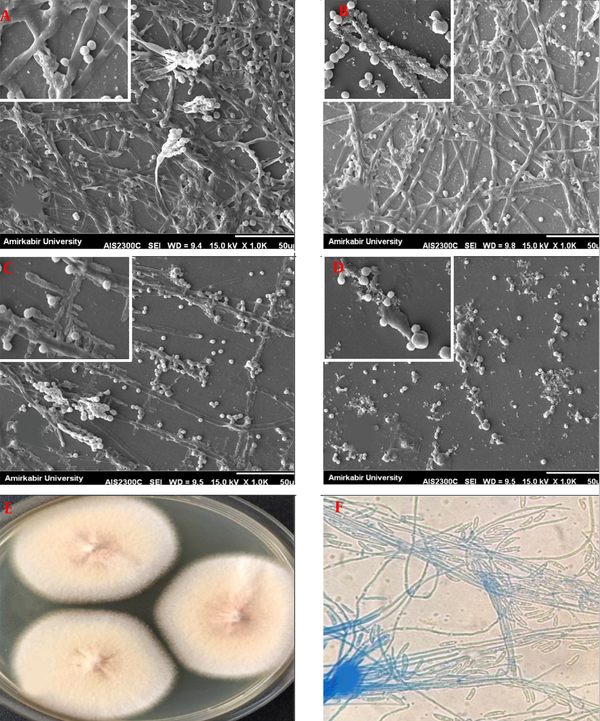
Scanning electron micrographs of preformed Aspergillus flavus biofilms in the presence of different voriconazole concentrations. (A) A. flavus biofilm control (without drug); (B through D) A. flavus biofilms after exposure to voriconazole at 1 µg/mL, 4 µg/mL, and 16 µg/mL, respectively (1000 and 5000×magnification). (E) Macroscopic and (F) Microscopic observation.
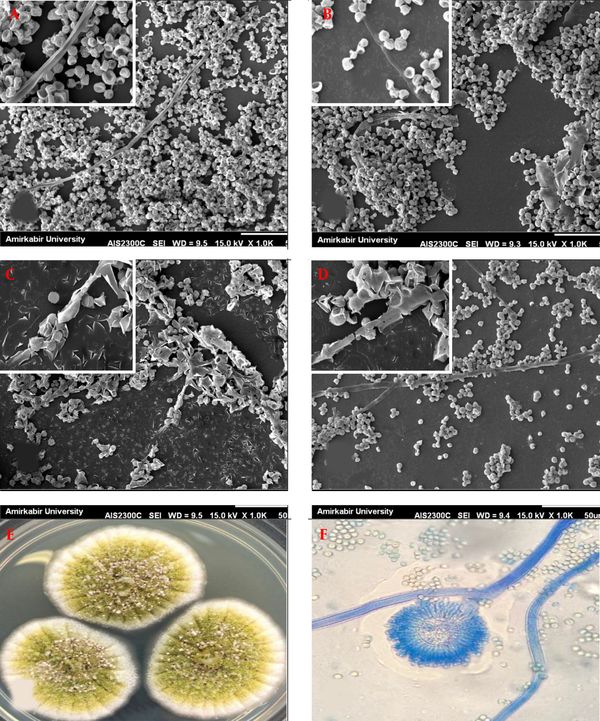
Unlike F. solani, A. flavus exhibited architecture containing abundant conidia (irregular groupings of microconidia) and few filamentous forms in control (Figure 2A). No significant changes in morphology were observed in the presence of 1 µg/mL VCZ (Figure 2B). All of the conidia revealed collapsed cell walls after exposure with 4 µg/mL VCZ, and degenerated hyphae were completely observed (Figure 2C). There was less outcome on conidial and hyphal morphology at 16 µg/mL VCZ and biofilms appeared morphologically highly similar to untreated biofilms; nevertheless, density and accumulation of conidia and hyphae became much lower (Figure 2D).
The growth of Aspergillus niger on polyester mesh squares described biofilm formation by filamentous fungi (14), and the growth of Aspergillus fumigatus was revealed by scanning electron microscopy and laser scanning confocal microscopy (LSCM) (15, 16). Biofilm formation of F. solani and F. oxysporum was analyzed by antibiotic susceptibility and LSCM (17).
Although the resistance mechanisms of filamentous species have not been completely elucidated, a hypothesis proposes that sessile cells are in a biofilm population which permanently bound to a surface and inserted in an exopolymeric background (18). Sessile cells are extremely resistant to the host defense mechanisms and to antifungal agents (19).
In this study, the ability of biofilm formation of F. solani and A. flavus strains, extracted from the fungal keratitis, had been compared with the investigation of their metabolic activity using the crystal violet staining method. The susceptibilities of sessile cells of A. flavus and F. solani were evaluated against the antifungals NAT, ICZ, VCZ, and PCZ.
Based on the results of this study, the biofilm formation of all tested isolates varied to great degrees. Accordingly, the complete biofilm of both filamentous fungi isolates could increase resistance to VCZ. These results are in line with those of a study indicating that the sessile cells of F. solani and F. oxysporum were less sensitive to NAT and VCZ (20). The initial and mature phases of Candida albicans biofilm had a significant difference with the values of the MICs, associated with their differential genes expression and resistance to the evolution of maturation of the biofilm (21).
The findings disclosed that the ability of biofilm formation was higher in F. solani strains than in A. flavus strains. This result is consistent with Chang et al. study that the ability of Fusarium to form biofilms on case wearing contact lenses might play the main role in the outbreak (22).
ICZ, PCZ and NAT had the maximum activity against biofilms of all tested filamentous strains (MIC ranging from 0.031 - 0.25 µg/mL, 0.031 - 0.25 µg/mL and 2 - 4 µg/mL), respectively. PCZ releaved the lowest MIC values against biofilms of all strains (MIC ranging from 0.031 - 0.25 µg/mL); however, no significant difference was observed for ICZ in this regard (P > 0.05).
However, they tended to be less susceptible to VOR than to the other agents. Three F. solani and one A. flavus strains showed the high MIC values against VCZ (MIC ≥ 1).
It was also found out that ICZ and PCZ were more active against sessile cells, while VCZ exhibited less activity against biofilms of strains.
In this respect, VCZ 1% and topical amphotericin B (0.3 - 0.5%) are recommended as alternative drugs. The deep penetration of topical VCZ to ocular is considered a considerable advantage (23). However, there is a studies revealing much better consequences in patients treated with NAT, compared to those of VCZ treatment (24-26). Wu et al. reported that VCZ had an extensive therapeutic scope covering filamentous and yeast fungi. VCZ revealing a suitable penetration to ocular, was used as an adjunct therapy to NAT in case of refractory to topical NAT (27). Although NAT shows poor activity against Aspergillus, NAT and VCZ have recently been the only FDA-approved drugs for the remedy of fungal infections caused by Fusarium spp. (28). Herein, VCZ showed resistance (≥ 1 μg/mL) against Fusarium and Aspergillus biofilms.
Mukherjee et al. predicted that efflux pumps had a vital role in azole resistance in the initial phase of Candida biofilm formation due to an alteration in sterol composition at the middle and complete phases (29). However, the basis for such differences has not been clarified. In another study, it was revealed that the less susceptibility to VCZ in Fusarium biofilms might be attributed to the upregulation of efflux pumps (29).
Based on the results of this study, the role of biofilm formation on resistance to antifungal agents was different, that is to say, although all of them were susceptible to NAT, ICZ, and PCZ, they exhibited different susceptibility to VCZ.
The persister cells are phenotypical variants of the wild type in biofilm structures and able to survive in spite of the presence of different concentration of antifungals (30).
Herein, the researchers used SEM to imagine morphological changes after exposure to VCZ. The value of 16 µg/mL VCZ was approved for sessile cells of F. solani with fewer conidia than untreated biofilms.
The SEM images indicated that 4 µg/mL VCZ caused considerable variations in the morphologies of preformed A. flavus biofilms, with the presence of collapsed conidia and degenerated hyphae. Biofilm morphological changes of A. fumigatus associated with the interaction of Staphylococccus aureus have been described previously (31). However, to the best of our knowledge, this survey prepares the first detailed SEM image analysis of F. solani and A. flavus biofilms adherent to disks. Fungal biofilms are among the dangerous medical problems worldwide since they can adhere to medical devices and acquire resistance to antifungal agents, and turn to infections extremely difficult to be eradicated. The ability of Fusarium strains and Aspergillus strains to form biofilms may lead to difficulty in the clinical management of keratitis. Therefore, it is recommended to know remedial concentrations of VCZ show potent in vitro activity against filamentous fungi biofilm and to have a knowledge of the mechanism of action, penetration rates, and therapeutic concentrations of drugs.
References
-
1.
Austin A, Lietman T, Rose-Nussbaumer J. Update on the Management of Infectious Keratitis. Ophthalmology. 2017;124(11):1678-89. [PubMed ID: 28942073]. [PubMed Central ID: PMC5710829]. https://doi.org/10.1016/j.ophtha.2017.05.012.
-
2.
Ansari Z, Miller D, Galor A. Current Thoughts in Fungal Keratitis: Diagnosis and Treatment. Curr Fungal Infect Rep. 2013;7(3):209-18. [PubMed ID: 24040467]. [PubMed Central ID: PMC3768010]. https://doi.org/10.1007/s12281-013-0150-110.1007/s12281-013-0150-1.
-
3.
Blanco AR, Nostro A, D'Angelo V, D'Arrigo M, Mazzone MG, Marino A. Efficacy of a Fixed Combination of Tetracycline, Chloramphenicol, and Colistimethate Sodium for Treatment of Candida albicans Keratitis. Invest Ophthalmol Vis Sci. 2017;58(10):4292-8. [PubMed ID: 28846777]. https://doi.org/10.1167/iovs.17-22047.
-
4.
Manikandan P, Abdel-Hadi A, Randhir Babu Singh Y, Revathi R, Anita R, Banawas S, et al. Fungal Keratitis: Epidemiology, Rapid Detection, and Antifungal Susceptibilities of Fusarium and Aspergillus Isolates from Corneal Scrapings. Biomed Res Int. 2019;2019:6395840. [PubMed ID: 30800674]. [PubMed Central ID: PMC6360544]. https://doi.org/10.1155/2019/6395840.
-
5.
Kuo MT, Chen JL, Hsu SL, Chen A, You HL. An Omics Approach to Diagnosing or Investigating Fungal Keratitis. Int J Mol Sci. 2019;20(15). [PubMed ID: 31349542]. [PubMed Central ID: PMC6695605]. https://doi.org/10.3390/ijms20153631.
-
6.
Bourcier T, Sauer A, Dory A, Denis J, Sabou M. Fungal keratitis. J Fr Ophtalmol. 2017;40(9):e307-13. [PubMed ID: 28987448]. https://doi.org/10.1016/j.jfo.2017.08.001.
-
7.
Cordova-Alcantara IM, Venegas-Cortes DL, Martinez-Rivera MA, Perez NO, Rodriguez-Tovar AV. Biofilm characterization of Fusarium solani keratitis isolate: increased resistance to antifungals and UV light. J Microbiol. 2019;57(6):485-97. [PubMed ID: 31134579]. https://doi.org/10.1007/s12275-019-8637-2.
-
8.
Soleimani M, Salehi Z, Fattahi A, Lotfali E, Yassin Z, Ghasemi R, et al. Ocular Fungi: Molecular Identification and Antifungal Susceptibility Pattern to Azoles. Jundishapur J Microbiol. 2020;13(3). https://doi.org/10.5812/jjm.99922.
-
9.
Sav H, Rafati H, Oz Y, Dalyan-Cilo B, Ener B, Mohammadi F, et al. Biofilm Formation and Resistance to Fungicides in Clinically Relevant Members of the Fungal Genus Fusarium. J Fungi (Basel). 2018;4(1). [PubMed ID: 29371509]. [PubMed Central ID: PMC5872319]. https://doi.org/10.3390/jof4010016.
-
10.
Manikandan P, Varga J, Kocsube S, Anita R, Revathi R, Nemeth TM, et al. Epidemiology of Aspergillus keratitis at a tertiary care eye hospital in South India and antifungal susceptibilities of the causative agents. Mycoses. 2013;56(1):26-33. [PubMed ID: 22487304]. https://doi.org/10.1111/j.1439-0507.2012.02194.x.
-
11.
Kim DJ, Lee MW, Choi JS, Lee SG, Park JY, Kim SW. Inhibitory activity of hinokitiol against biofilm formation in fluconazole-resistant Candida species. PLoS One. 2017;12(2). e0171244. [PubMed ID: 28152096]. [PubMed Central ID: PMC5289548]. https://doi.org/10.1371/journal.pone.0171244.
-
12.
Meletiadis J, Meis JF, Mouton JW, Donnelly JP, Verweij PE. Comparison of NCCLS and 3-(4,5-dimethyl-2-Thiazyl)-2, 5-diphenyl-2H-tetrazolium bromide (MTT) methods of in vitro susceptibility testing of filamentous fungi and development of a new simplified method. J Clin Microbiol. 2000;38(8):2949-54. [PubMed ID: 10921957]. [PubMed Central ID: PMC87156]. https://doi.org/10.1128/JCM.38.8.2949-2954.2000.
-
13.
Nasrollahi A, Pourshamsian KH, Mansourkiaee P. Antifungal activity of silver nanoparticles on some of fungi. Int J Nano Dimens. 2011.
-
14.
Villena GK, Gutierrez-Correa M. Production of cellulase by Aspergillus niger biofilms developed on polyester cloth. Lett Appl Microbiol. 2006;43(3):262-8. [PubMed ID: 16910929]. https://doi.org/10.1111/j.1472-765X.2006.01960.x.
-
15.
Beauvais A, Schmidt C, Guadagnini S, Roux P, Perret E, Henry C, et al. An extracellular matrix glues together the aerial-grown hyphae of Aspergillus fumigatus. Cell Microbiol. 2007;9(6):1588-600. [PubMed ID: 17371405]. https://doi.org/10.1111/j.1462-5822.2007.00895.x.
-
16.
Mowat E, Lang S, Williams C, McCulloch E, Jones B, Ramage G. Phase-dependent antifungal activity against Aspergillus fumigatus developing multicellular filamentous biofilms. J Antimicrob Chemother. 2008;62(6):1281-4. [PubMed ID: 18819968]. https://doi.org/10.1093/jac/dkn402.
-
17.
Imamura Y, Chandra J, Mukherjee PK, Lattif AA, Szczotka-Flynn LB, Pearlman E, et al. Fusarium and Candida albicans biofilms on soft contact lenses: model development, influence of lens type, and susceptibility to lens care solutions. Antimicrob Agents Chemother. 2008;52(1):171-82. [PubMed ID: 17999966]. [PubMed Central ID: PMC2223913]. https://doi.org/10.1128/AAC.00387-07.
-
18.
Serrano-Fujarte I, Lopez-Romero E, Reyna-Lopez GE, Martinez-Gamez MA, Vega-Gonzalez A, Cuellar-Cruz M. Influence of culture media on biofilm formation by Candida species and response of sessile cells to antifungals and oxidative stress. Biomed Res Int. 2015;2015:783639. [PubMed ID: 25705688]. [PubMed Central ID: PMC4331161]. https://doi.org/10.1155/2015/783639.
-
19.
Lewis K. Multidrug tolerance of biofilms and persister cells. Curr Top Microbiol Immunol. 2008;322:107-31. [PubMed ID: 18453274]. https://doi.org/10.1007/978-3-540-75418-3_6.
-
20.
Mukherjee PK, Chandra J, Yu C, Sun Y, Pearlman E, Ghannoum MA. Characterization of fusarium keratitis outbreak isolates: contribution of biofilms to antimicrobial resistance and pathogenesis. Invest Ophthalmol Vis Sci. 2012;53(8):4450-7. [PubMed ID: 22669723]. [PubMed Central ID: PMC3394686]. https://doi.org/10.1167/iovs.12-9848.
-
21.
Chandra J, Kuhn DM, Mukherjee PK, Hoyer LL, McCormick T, Ghannoum MA. Biofilm formation by the fungal pathogen Candida albicans: development, architecture, and drug resistance. J Bacteriol. 2001;183(18):5385-94. [PubMed ID: 11514524]. [PubMed Central ID: PMC95423]. https://doi.org/10.1128/JB.183.18.5385-5394.2001.
-
22.
Chang DC, Grant GB, O'Donnell K, Wannemuehler KA, Noble-Wang J, Rao CY, et al. Multistate outbreak of Fusarium keratitis associated with use of a contact lens solution. JAMA. 2006;296(8):953-63. [PubMed ID: 16926355]. https://doi.org/10.1001/jama.296.8.953.
-
23.
Srinivasan M. Fungal keratitis. Curr Opin Ophthalmol. 2004;15(4):321-7. [PubMed ID: 15232472]. https://doi.org/10.1097/00055735-200408000-00008.
-
24.
Thiel MA, Zinkernagel AS, Burhenne J, Kaufmann C, Haefeli WE. Voriconazole concentration in human aqueous humor and plasma during topical or combined topical and systemic administration for fungal keratitis. Antimicrob Agents Chemother. 2007;51(1):239-44. [PubMed ID: 17060517]. [PubMed Central ID: PMC1797668]. https://doi.org/10.1128/AAC.00762-06.
-
25.
Sharma S, Das S, Virdi A, Fernandes M, Sahu SK, Kumar Koday N, et al. Re-appraisal of topical 1% voriconazole and 5% natamycin in the treatment of fungal keratitis in a randomised trial. Br J Ophthalmol. 2015;99(9):1190-5. [PubMed ID: 25740805]. https://doi.org/10.1136/bjophthalmol-2014-306485.
-
26.
Prajna NV, Krishnan T, Mascarenhas J, Rajaraman R, Prajna L, Srinivasan M, et al. The mycotic ulcer treatment trial: a randomized trial comparing natamycin vs voriconazole. JAMA Ophthalmol. 2013;131(4):422-9. [PubMed ID: 23710492]. [PubMed Central ID: PMC3769211]. https://doi.org/10.1001/jamaophthalmol.2013.1497.
-
27.
Wu J, Zhang W, Zhao J, Zhou H. Review of clinical and basic approaches of fungal keratitis. Int J Ophthalmol. 2016;9(11):1676-83.
-
28.
Prajna NV, Mascarenhas J, Krishnan T, Reddy PR, Prajna L, Srinivasan M, et al. Comparison of natamycin and voriconazole for the treatment of fungal keratitis. Arch Ophthalmol. 2010;128(6):672-8. [PubMed ID: 20547942]. [PubMed Central ID: PMC3774126]. https://doi.org/10.1001/archophthalmol.2010.102.
-
29.
Mukherjee PK, Chandra J, Kuhn DM, Ghannoum MA. Mechanism of fluconazole resistance in Candida albicans biofilms: phase-specific role of efflux pumps and membrane sterols. Infect Immun. 2003;71(8):4333-40. [PubMed ID: 12874310]. [PubMed Central ID: PMC165995]. https://doi.org/10.1128/IAI.71.8.4333-4340.2003.
-
30.
LaFleur MD, Kumamoto CA, Lewis K. Candida albicans biofilms produce antifungal-tolerant persister cells. Antimicrob Agents Chemother. 2006;50(11):3839-46. [PubMed ID: 16923951]. [PubMed Central ID: PMC1635216]. https://doi.org/10.1128/AAC.00684-06.
-
31.
Ramirez Granillo A, Canales MG, Espindola ME, Martinez Rivera MA, de Lucio VM, Tovar AV. Antibiosis interaction of Staphylococccus aureus on Aspergillus fumigatus assessed in vitro by mixed biofilm formation. BMC Microbiol. 2015;15:33. [PubMed ID: 25880740]. [PubMed Central ID: PMC4335557]. https://doi.org/10.1186/s12866-015-0363-2.