Abstract
Background:
In recent years, science and industry have focused on preparing nanoparticles (NPs). Due to the resistance of bacteria to antibiotics, there is an overwhelming need to find effective antimicrobials with fewer side effects.Objectives:
The aim of this study was to synthesize silver and iron oxide NPs and investigate their antimicrobial effects on bacteria isolated from urinary stones.Methods:
Cases of urolithiasis from 45 patients with staghorn stones, extracted through percutaneous nephrolithotomy (PCNL), were included in the study. Urinary stone cultures were performed, and the isolation and identification of bacteria were done using standard microbiological techniques. Silver and iron oxide NPs were synthesized, and the minimum bactericidal concentration (MBC) and minimum inhibitory concentration (MIC) were evaluated for the isolates.Results:
Seventeen (60.7%) out of 28 positive cultured cases of the isolated bacteria were gram-negative, and 11 (39.3%) were gram-positive. The most abundant isolated bacterium was Escherichia coli, with 13 cases (46.4%). The antibacterial effects of silver and iron oxide NPs revealed that the maximum inhibitory zone for Ag NPs at 1000 ppm was 23 mm, and for Fe3O4 NPs, it was 18.5 mm. The MIC was 180 µg/mL for Ag and 250 µg/mL for Fe3O4. The MBC was 228 µg/mL for Ag and 300 µg/mL for Fe3O4.Conclusions:
Nanoparticles exhibited antibacterial effects on the bacteria studied in a concentration-dependent manner. Ag NPs showed a more pronounced bactericidal effect than Fe3O4 NPs. As a result, these two NPs demonstrated effective antibacterial activity against both gram-positive and gram-negative bacteria.Keywords
Urinary Stones Antibiogram Antibacterial Effects Nanoparticles (NPs) MBC MIC
1. Background
Urinary stones are a fundamental problem in the urinary system, influenced by various factors such as nutrition, weather, gender, genetics, and urease-positive bacteria. Most infectious stones in the urinary tract are caused by microorganisms that synthesize the urease enzyme (1). The hydrolysis of urea by urease produces ammonia, making the urine highly alkaline and leading to the formation of carbonated apatite. The entrapment of infectious microorganisms within the forming stone creates an infection that is usually resistant to antimicrobial treatment, as antibiotics cannot penetrate the stone, often resulting in persistent bacterial infections (2).
Antibiotic resistance and the indiscriminate use of drugs in human pathogenic bacteria, along with the side effects of these drugs, pose significant challenges in the medical field. Therefore, identifying antimicrobial compounds with effective antibacterial properties is a priority in medical and pharmaceutical research (3).
Nanoparticles (NPs) play a crucial role in addressing environmental and microbial contamination in nanotechnology. Nanoparticles have wide applications in various fields, including packaging, fuel additives, pharmaceuticals, and medicine (4). Due to their small size, NPs exhibit changes in reactivity and characteristics, impacting their antibacterial efficacy. Smaller NPs have a high surface-to-volume ratio, enhancing their antibacterial activity (5).
There are two main types of NPs: Metallic and non-metallic. The ratio of surface area to volume of metal oxide nanoparticles (MONPs) affects their antibacterial properties. Metal oxide nanoparticles such as Fe3O4, TiO2, CuO, and ZnO are well known for effectively suppressing a wide range of bacteria and reducing the development of resistance (6). However, concerns about their toxicity to cells may limit their practical applications. Polymetallic oxides are becoming increasingly attractive for biological applications due to their ability to produce synergistic effects and combine the beneficial physicochemical characteristics of their individual components (7). Addressing microbial resistance to antibiotics is critical, and inorganic nanomaterials, particularly MONPs, are considered a potential remedy due to their durability, lower toxicity, and better stability and selectivity compared to organic materials (8).
Silver nanoparticles (AgNPs) are smaller than microorganisms, allowing them to bind to cells and disrupt membranes. The toxicity level of AgNPs is influenced by factors such as size, concentration, environmental pH, and exposure duration to pathogens (9). The antibacterial properties of AgNPs are effective against various microorganisms. Iron oxide NPs, including Fe3O4 and oxidized Fe2O3, range in size from 1 to 100 nm and have notable paramagnetic characteristics, making them attractive for biological and medical applications (10). Iron oxide NPs offer greater stability, long life, safety, and resistance to a wide range of microbes (11). Zinc oxide NPs are also widely used and known for their high chemical stability and antibacterial properties, making them strong competitors for antibiotics (12).
Given the importance of addressing drug resistance, particularly antibiotic resistance in pathogens, and reducing the use of broad-spectrum antibiotics that contribute to resistance and adverse effects, NPs can enhance the efficacy of antibiotics against pathogenic agents (13).
2. Objectives
This research employs nanotechnology to inhibit antibiotic-resistant bacterial infections in large urinary stones using silver and iron oxide NPs. Due to the size of these stones, antibiotics cannot penetrate and destroy the core, which harbors the bacteria.
3. Methods
3.1. Isolation and Processing of Urinary Stones
In this hospital study, the urinary stones of 45 patients at Shahid Labbafinejad Hospital were removed during percutaneous nephrolithotomy (PCNL). Participants in the research ranged from 22 to 73 years old, with men accounting for 49% and women for 51%. All patients provided written consent before the collection of stones. The project received approval from the Ethics Committee of Azad University, Tehran Branch, Tehran, Iran, and was designated with an ethics code (IR.IAU.CTB.REC.1400.065).
The stones were transported in sterile normal saline to the university's microbiology laboratory. In the laboratory, each stone was washed five times with physiological serum, crushed into a powder using a sterilized mortar, and suspended in 5cc of physiological serum.
3.2. Identification of Isolated Bacteria of Urinary Stones
Stone suspension (0.001 mL) was incubated on eosin methylene blue agar and blood agar media at 37°C for 24 - 48 hours under sterile conditions. The morphology of grown colonies, their size, and gram staining were analyzed. Differential media such as TSI, simmons citrate, SIM, and MR-VP were used to identify the gram-negative bacilli. Catalase tests were performed to detect gram-positive cocci. If the test was positive, mannitol, DNase, coagulase, alkaline phosphatase, and urease fermentation tests were implemented. An antibiotic sensitivity test was conducted using the Kirby-Bauer method with mueller hinton culture medium, 0.5 McFarland standard microbial suspension, and antibiotic discs, including ciprofloxacin (5 µg), amikacin (20 µg), ceftriaxone (30 µg), gentamicin (10 µg), nalidixic acid (30 µg), and vancomycin (30 µg).
3.3. Preparation of Nanoparticles
Iron oxide NPs were created using the co-precipitation method, involving water, sodium hydroxide, and ferric iron chloride as solvents. Specifically, 0.21 grams of ferric chloride and 2 mmol of sodium hydroxide were combined and heated to 60°C for 45 minutes to produce the NPs. Simultaneously, a stabilizer was prepared by mixing 4 mmol of sodium dodecyl sulfate surfactant with 10 mL of methanol solvent at 60°C for 45 minutes in a separate container. The contents of both containers were then combined and placed into a 60-watt ultrasonic bath at 60°C. The resulting precipitate was separated, washed of any impurities using double-distilled water and ethanol, and then dried for 24 hours at 60°C in an oven (14).
To make AgNPs, a solution containing 100 mL of 10-3 M silver nitrate (AgNO3) and 300 mL of 10-3 M sodium borohydride (NaBH4) was prepared. In a water bath, NaBH4 was heated, and AgNO3 was gradually added while being rapidly stirred. An ultrasonic bath was used simultaneously. Throughout the procedure, the solution changed color from yellow to dark. Dynamic light scattering (DLS), scanning electron microscopy (SEM), and zeta potential were used to characterize both the silver and iron oxide NPs in terms of size and shape (15).
3.4. Effect of Nanoparticles on Isolated Bacteria
A 24-hour pure culture of each bacterium was prepared in trypticase broth liquid medium. A standard suspension with turbidity equal to that of 0.5 McFarland's solution was then prepared in sterile physiological serum. This turbidity has a light absorption equal to 1, and according to the standard of 0.5 McFarland, the bacterial count is 1.5 × 108 colony-forming units per milliliter. Each bacterium was cultured separately in mueller hinton Agar medium. Concentrations of 25, 50, 100, 500, and 1000 ppm of NPs were prepared, and 4 mm diameter wells were placed in the mueller hinton Agar culture medium. Then, 20 µL of the prepared NP dilutions were poured into the wells. Solvent (H2O) was used as a negative control, and the results of antibiotic sensitivities were used as positive controls. After the necessary preparations, the plates were incubated at 37°C for 24 hours. After incubation, the inhibition zones around the wells were recorded in millimeters.
3.5. Minimum Inhibitory Concentration (MIC) and Minimum Bactericidal Concentration (MBC) Determination
A single colony was created on the eosin methylene blue agar medium and incubated for 24 hours at 37°C. A loopful was then transferred from this medium to the nutrient broth medium and incubated for another 24 hours. To determine minimum inhibitory concentration (MIC) and minimum bactericidal concentration (MBC), a series of 8 tubes was prepared. Six tubes were used for dilution, 1 tube as a positive control (containing culture medium and NPs), and 1 tube as a negative control (containing bacterial suspension and culture medium). One mL of nutrient broth (N.B.) culture medium was poured into each tube, and 1 mL of NPs with the highest concentration was added to the first tube. Then, 1 mL from the first tube was removed and added to the second tube, and this process continued until the last tube. Fifty µL of bacterial suspension were added to all tubes. The tubes were incubated for 24 hours, and after incubation, the first tube without turbidity was considered the MIC for each sample. Mueller hinton Agar medium was used to culture the tubes that showed no turbidity. The lowest bacterial concentration at which growth was hindered was identified as the MBC.
3.6. Statistical Analysis
Analysis of variance (ANOVA) was employed to analyze the MBC results, using mean and standard deviation. The MBC of the NPs was assessed by applying Tukey's post hoc analysis. A predetermined level of significance for all statistical tests was specified as P < 0.05.
4. Results
In the total cultured stones, there were 28 positive cultures, including 11 (39.2%) gram-positive and 17 (60.7%) gram-negative bacteria. The most abundant bacterium isolated was Escherichia coli, with a frequency of 13 (46.4%), while the lowest frequency was related to Enterobacter aerogenes, Enterobacter pyrinus, Staphylococcus kohni, and Staphylococcus aureus, each with a frequency of 2 (7.14%) (Table 1). The percentage of antibiotic resistance among bacteria isolated from kidney stones of patients is summarized in Table 2.
Frequency of Bacteria Isolated from Stone Cultures Isolated from all Patients
Type of Bacteria | Isolates (%) |
---|---|
Escherichia coli | 13 (46.42) |
Enterobacter aerogenes | 2 (7.14) |
Enterobacter pyrinus | 2 (7.14) |
Staphylococcus kohni | 2 (7.14) |
Staphylococcus aureus | 2 (7.14) |
Staphylococcus caprae | 7 (25) |
Total | 28 (100) |
Antibiotic Resistance Percentage of Bacteria Isolated from Kidney Stones of Patient on the Measurement of the Inhibition Zone Diameter
Bacteria | Ciprofloxacin | Amikacin | Gentamycin | Nalidixic Acid | Vancomycin | Ceftriaxone |
---|---|---|---|---|---|---|
Escherichia coli | 38.5 | 5.29 | 27.2 | 54.4 | - | 40 |
Staphylococcus kohni | 26.4 | 16.7 | 37.5 | 47.2 | 9.72 | 23.2 |
Enterobacter aerogenes | 42.2 | 12.5 | 37.5 | 45.6 | 25 | 53.6 |
Staphylococcus aureus | 37.5 | 13.5 | 23.6 | 44.7 | 16.5 | 31.3 |
Enterobacter pyrinus | 65.6 | 23.4 | 41.5 | 64.3 | 12.5 | 62.5 |
Staphylococcus caprae | 25 | 14.6 | 32.6 | 50 | 11.6 | 33.3 |
Figure 1 shows the use of a SEM to identify and analyze the iron oxide and AgNPs on a microscopic scale. Dynamic light scattering and SEM analyses showed that the size of iron oxide NPs was about 80 nm and AgNPs were less than 10 nanometers (Figure 2).
Scanning electron microscope (SEM) image of A, iron oxide nanoparticles; and B, silver nanoparticles
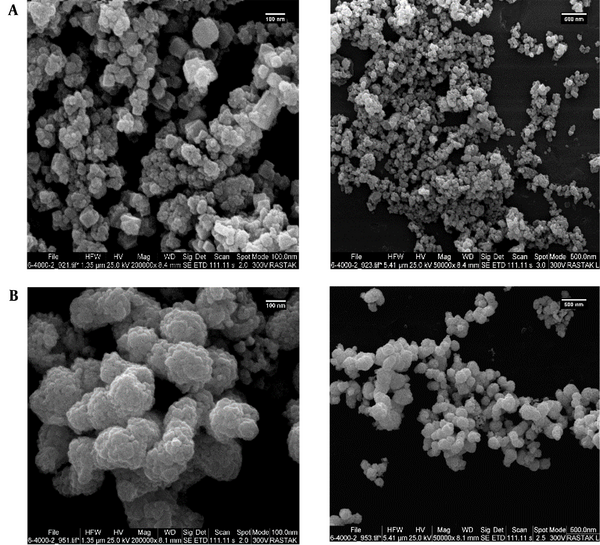
Dynamic light scattering (DLS) test showed the size of iron oxide nanoparticles (A); and silver nanoparticles (B).
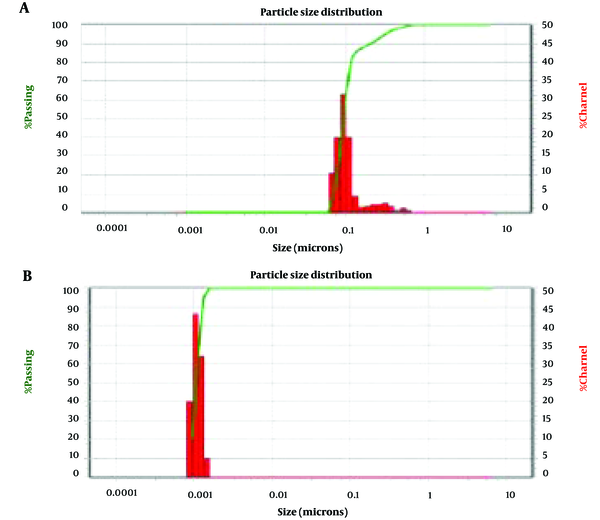
The most important role of zeta potential in NPs’ behavior is its effect on the stability and durability of very fine particles dispersed in water environments. The degree of repulsion between particles with similar or nearby charges depends on the zeta potential of the particles in a solution. Smaller particles must have a higher zeta potential to be stable because they have a higher tendency to aggregate and accumulate. Additionally, many researchers have reported that negatively charged surfaces positively affect the germination of dross in a simulated body solution, while positively charged surfaces prevent germination. This phenomenon is believed to be due to the accumulation of ions near negatively charged surfaces, leading to the initiation of Ca2+ germination.
The zeta potential of particles (surface charge) was calculated using the laser Doppler electrophoresis (LDE) method to evaluate the stability of particles in different environments. Measurements were made in water three times consecutively. The zeta potential of NPs in water showed a negative value (-16.9 for Ag NPs and -17.9 for Fe3O4 NPs). The negative zeta potential has an important biological effect on cell behavior in in-vivo conditions, stimulating cell attachment, multiplication, and proliferation.
The effect of different concentrations of Ag and Fe3O4 NPs on bacteria isolated from the stones was identified based on the measurement of the inhibition zone diameter. The maximum inhibitory zone of Ag NPs was 23 mm at 1000 ppm (Table 3), and for Fe3O4 NPs, it was 18.5 mm for E. coli at the same concentration (Table 4).
Investigating the Effect of Different Concentrations of Ag Nanoparticles (NPs) on Bacteria Isolated from the Stone Based on the Measurement of the Inhibition Zone Diameter (mm)
Nanoparticle Concentration (ppm) Bacteria | 25 | 50 | 100 | 500 | 1000 |
---|---|---|---|---|---|
Escherichia coli | 10.5 | 15.5 | 14 | 18.5 | 23 |
Enterobacter aerogenes | 11 | 13 | 14.5 | 17.5 | 19 |
Enterobacter pyrinus | 12 | 14.5 | 14 | 16.5 | 19 |
Staphylococcus kohni | 10 | 11 | 11.5 | 14 | 17 |
Staphylococcus aureus | 10 | 12 | 12.5 | 15 | 18.5 |
Staphylococcus caprae | 9 | 11 | 11 | 13.5 | 17 |
Investigating the Effect of Different Concentrations of Fe3O4 Nanoparticles (NPs) on Bacteria Isolated from the Stone Based on Measurement of the Inhibition Zone Diameter (mm)
Nanoparticle Concentration (ppm) Bacteria | 25 | 50 | 100 | 500 | 1000 |
---|---|---|---|---|---|
Escherichia coli | 11 | 12 | 12.5 | 16 | 18.5 |
Enterobacter aerogenes | 10 | 11 | 11 | 13.5 | 15 |
Enterobacter pyrinus | 7 | 10 | 13 | 15.5 | 17 |
Staphylococcus kohni | 8 | 10 | 11.5 | 13 | 15.5 |
Staphylococcus aureus | 11 | 13 | 13 | 15 | 17.5 |
Staphylococcus caprae | 10 | 12 | 12.5 | 14.5 | 16.5 |
By measuring the dimensions of the zones where bacterial growth was inhibited, the effects of Ag and Fe3O4 NPs on bacteria isolated from stones were assessed. Both types of NPs had an inhibitory effect on the isolated bacteria depending on their concentration. Across all bacterial isolates, Ag NPs demonstrated a greater capacity to kill bacteria than Fe3O4 NPs, especially at lower concentrations. Ag NPs showed the highest level of inhibition on E.coli at a concentration of 180 µg/mL-1, whereas Fe3O4 NPs showed the highest level of inhibition at a concentration of 250 µg/mL-1 (Table 5). These findings suggest that NPs alter the activity of enzymes found in bacterial membranes to exert an antibacterial effect proportional to their concentration.
Minimum inhibitory concentration (MIC) and minimum bactericidal concentration (MBC) Results from the Effect of Ag Nanoparticles (NPs) and Fe3O4 NPs on Bacteria in µg/mL
Bacteria | Fe3O4 Nanoparticles | Ag Nanoparticles | ||
---|---|---|---|---|
MIC | MBC | MIC | MBC | |
Escherichia coli | 250 | 300 | 180 | 228 |
Enterobacter aerogenes | 400 | 550 | 220 | 380 |
Enterobacter pyrinus | 320 | 400 | 218 | 238 |
Staphylococcus kohni | 410 | 550 | 318 | 343 |
Staphylococcus aureus | 390 | 380 | 238 | 284 |
Staphylococcus caprae | 450 | 520 | 320 | 352 |
5. Discussion
As revealed by the results, 28 (62.2%) of the isolated stones were contaminated. The isolated bacteria included 6 different strains, with gram-positive strains (S. kohni, S. caprae, S. aureus) and gram-negative strains (E. aerogenes, E. coli, and E. pyrinus). The highest antibiotic sensitivity in gram-negative bacteria was related to ciprofloxacin, nalidixic acid, and cceftriaxone, while the highest resistance was to amikacin. In gram-positive bacteria, the highest sensitivity was to nalidixic acid, and the highest resistance was to vancomycin.
Research and development in various fields of nanotechnology have been expanding, encompassing diverse scientific disciplines. Numerous investigations have examined the antimicrobial properties of metal NPs to determine their effectiveness against bacteria and fungi (16-19). In recent years, drug resistance has become a global concern because the inappropriate and indiscriminate use of antimicrobial drugs fosters the development of drug-resistant microbes, leading to prolonged illness and increased risk of death (20). Nanomaterials are important due to their high surface-to-volume ratio and reactive activity. Additionally, they remain active under unfavorable conditions, such as high sterilization temperatures, which leads to the inactivation of antibiotics (21).
The aim of the current investigation was to evaluate the effectiveness of iron and silver oxide NPs as antibacterial and inhibitory agents against bacteria isolated from kidney stones. The results showed that even at low concentrations, both gram-negative and gram-positive bacteria were sensitive to the growth-inhibitory and bactericidal effects of iron oxide NPs (60 nm) and silver oxide NPs (10 nm). Reactive oxygen species (ROS) production, lipid peroxidation, and cell wall structure were found to impact bacterial sensitivity to NPs (22). The surface-to-volume ratio of MONPs affects their antibacterial efficacy. The study discovered that as the concentration of NPs increases, so does the degree of bacterial elimination.
Silver ions, with a size between 1 and 10 nanometers, are used in nano-silver technology because they are more stable than other solutions (23). The antimicrobial properties of Fe3O4 NPs can be explained by different mechanisms, including the production of ROS such as superoxide radicals (O2-), hydroxide radicals (OH-), and singlet oxygen. The result is that NPs have noticeable antibacterial effects on the tested bacteria. The differing effects of Ag NPs and Fe3O4 NPs on bacterial growth could be attributed to differences in how bacteria and NPs interact. Since NPs can closely interact with the bacterial membrane, enter the cell, and deactivate it, their small size may enhance their antibacterial activity (24).
According to published research, MONPs including iron, magnesium, and zinc oxide are considered less toxic to humans than silver and copper oxide NPs. However, due to their powerful ability to kill both gram-negative and gram-positive bacteria, as well as their affordability, NPs hold significant potential for treating infections.
5.1. Conclusions
In the present investigation, the comparison of MBC and MIC of iron oxide and AgNPs showed that AgNPs have a stronger antibacterial effect than iron oxide. Generally, both NPs can be suitable choices to control pathogenic microbes, and a direct relationship can be concluded between the concentration percentage of NPs and the removal of bacteria. Accordingly, they exhibited stronger antibacterial properties at higher concentrations.
Acknowledgements
References
-
1.
Prezioso D, Strazzullo P, Lotti T, Bianchi G, Borghi L, Caione P, et al. Dietary treatment of urinary risk factors for renal stone formation. A review of CLU Working Group. Arch Ital di Urol Androl. 2015;87(2):105. https://doi.org/10.4081/aiua.2015.2.105.
-
2.
Zhang D, Li S, Zhang Z, Li N, Yuan X, Jia Z, et al. Urinary stone composition analysis and clinical characterization of 1520 patients in central China. Sci Rep. 2021;11(1). https://doi.org/10.1038/s41598-021-85723-3.
-
3.
Nirumand MC, Hajialyani M, Rahimi R, Farzaei MH, Zingue S, Nabavi SM, et al. Dietary Plants for the Prevention and Management of Kidney Stones: Preclinical and Clinical Evidence and Molecular Mechanisms. Int J Mol Sci. 2018;19(3). [PubMed ID: 29518971]. [PubMed Central ID: PMC5877626]. https://doi.org/10.3390/ijms19030765.
-
4.
Moslemi MK, Saghafi H, Joorabchin SMA. Evaluation of biochemical urinary stone composition and its relationship to tap water hardness in Qom province, central Iran. International Journal of Nephrology and Renovascular Disease. 2011;4:145-8. https://doi.org/10.2147/ijnrd.s25006.
-
5.
Díez-Pascual AM. Antibacterial Action of Nanoparticle Loaded Nanocomposites Based on Graphene and Its Derivatives: A Mini-Review. Int J Mol Sci. 2020;21(10):3563. https://doi.org/10.3390/ijms21103563.
-
6.
Zhang S, Gao H, Bao G. Physical Principles of Nanoparticle Cellular Endocytosis. ACS Nano. 2015;9(9):8655-71. https://doi.org/10.1021/acsnano.5b03184.
-
7.
Mirzaei A, Janghorban K, Hashemi B, Bonavita A, Bonyani M, Leonardi S, et al. Synthesis, Characterization and Gas Sensing Properties of Ag@α-Fe2O3 Core–Shell Nanocomposites. Nanomater. 2015;5(2):737-49. https://doi.org/10.3390/nano5020737.
-
8.
Jin S, Lee H, Yim S. Enhanced capacitive properties of all-metal-oxide-nanoparticle-based asymmetric supercapacitors. RSC Adv. 2019;9(55):31846-52. https://doi.org/10.1039/c9ra06066a.
-
9.
Hoshyar N, Gray S, Han H, Bao G. The Effect of Nanoparticle Size on In Vivo Pharmacokinetics and Cellular Interaction. Nanomed. 2016;11(6):673-92. https://doi.org/10.2217/nnm.16.5.
-
10.
Zhang X, Liu Z, Shen W, Gurunathan S. Silver Nanoparticles: Synthesis, Characterization, Properties, Applications, and Therapeutic Approaches. Int J Mol Sci. 2016;17(9):1534. https://doi.org/10.3390/ijms17091534.
-
11.
Liao C, Li Y, Tjong SC. Bactericidal and Cytotoxic Properties of Silver Nanoparticles. Int J Mol Sci. 2019;20(2):449. https://doi.org/10.3390/ijms20020449.
-
12.
Rhazouani A, Gamrani H, El Achaby M, Aziz K, Gebrati L, Uddin MS, et al. Synthesis and Toxicity of Graphene Oxide Nanoparticles: A Literature Review of In Vitro and In Vivo Studies. BioMed Res Int. 2021;2021:1-19. https://doi.org/10.1155/2021/5518999.
-
13.
Agban Y, Mugisho OO, Thakur SS, Rupenthal ID. Characterization of Zinc Oxide Nanoparticle Cross-Linked Collagen Hydrogels. Gels. 2020;6(4):37. https://doi.org/10.3390/gels6040037.
-
14.
Amreddy N, Babu A, Muralidharan R, Panneerselvam J, Srivastava A, Ahmed R, et al. Recent Advances in Nanoparticle-Based Cancer Drug and Gene Delivery. In: Tew KD, Fisher PB, editors. Advances in Cancer Research. 137. Amsterdam, Netherlands: Elsevier; 2018. p. 115-70. https://doi.org/10.1016/bs.acr.2017.11.003.
-
15.
Phan M, Alonso J, Khurshid H, Lampen-Kelley P, Chandra S, Stojak Repa K, et al. Exchange Bias Effects in Iron Oxide-Based Nanoparticle Systems. Nanomater. 2016;6(11):221. https://doi.org/10.3390/nano6110221.
-
16.
Aqeel T, Bumajdad A. Facile and Direct Preparation of Ultrastable Mesoporous Silica with Silver Nanoclusters: High Surface Area. Chem Open. 2020;9(1):87-92. https://doi.org/10.1002/open.201900275.
-
17.
Jędrzejczyk RJ, Turnau K, Jodłowski PJ, Chlebda DK, Łojewski T, Łojewska J. Antimicrobial Properties of Silver Cations Substituted to Faujasite Mineral. Nanomater. 2017;7(9):240. https://doi.org/10.3390/nano7090240.
-
18.
Qindeel M, Barani M, Rahdar A, Arshad R, Cucchiarini M. Nanomaterials for the Diagnosis and Treatment of Urinary Tract Infections. Nanomater. 2021;11(2):546. https://doi.org/10.3390/nano11020546.
-
19.
Shakerimoghaddam A, Ghaemi EA, Jamalli A. Zinc oxide nanoparticle reduced biofilm formation and antigen 43 expressions in uropathogenic Escherichiacoli. Iran J Basic Med Sci. 2017;20(4):451-6. [PubMed ID: 28804616]. [PubMed Central ID: PMC5425929]. https://doi.org/10.22038/ijbms.2017.8589.
-
20.
Mukherjee A, Rahuman HBH, Dhandapani R, Palanivel V, Thangavelu S, Paramasivam R, et al. Bioengineered phytomolecules-capped silver nanoparticles using Carissa carandas leaf extract to embed on to urinary catheter to combat UTI pathogens. Plos One. 2021;16(9). e0256748. https://doi.org/10.1371/journal.pone.0256748.
-
21.
Liao S, Zhang Y, Pan X, Zhu F, Jiang C, Liu Q, et al.
Antibacterial activity and mechanism of silver nanoparticles against multidrug-resistant Pseudomonas aeruginosa
. Int J Nanomed. 2019;Volume 14:1469-87. https://doi.org/10.2147/ijn.s191340. -
22.
Song W, Ge S. Application of Antimicrobial Nanoparticles in Dentistry. Mol. 2019;24(6):1033. https://doi.org/10.3390/molecules24061033.
-
23.
Luo J, Zhang Y, Zhu S, Tong Y, Ji L, Zhang W, et al. The application prospect of metal/metal oxide nanoparticles in the treatment of osteoarthritis. Naunyn-Schmiedeberg's Arch Pharmacol. 2021;394(10):1991-2002. https://doi.org/10.1007/s00210-021-02131-0.
-
24.
Chausov DN, Smirnova VV, Burmistrov DE, Sarimov RM, Kurilov AD, Astashev ME, et al. Synthesis of a Novel, Biocompatible and Bacteriostatic Borosiloxane Composition with Silver Oxide Nanoparticles. Mater. 2022;15(2):527. https://doi.org/10.3390/ma15020527.