Abstract
Background:
Klebsiella pneumoniae is a formidable nosocomial pathogen increasingly known for its antibiotic resistance, posing a significant global health challenge. The emergence of carbapenem-resistant strains has necessitated the exploration of alternative therapeutic strategies.Objectives:
This study aimed to evaluate the synergistic effects of combining nisin with colistin against K. pneumoniae isolates and to determine whether this combination enhances antibacterial efficacy compared to colistin alone.Methods:
Ten K. pneumoniae isolates were collected from hospitalized patients in Iran. Antimicrobial susceptibility testing was conducted using the Kirby-Bauer disk diffusion method. Minimum inhibitory concentration (MIC) assays were performed to determine the MICs of nisin and colistin. Synergistic activity testing, biofilm formation assays, and time-kill assays were also carried out to assess the effectiveness of the nisin-colistin combination.Results:
The study demonstrated that the nisin-colistin combination exhibited a synergistic effect, significantly reducing colony counts over 24 hours for select isolates. However, the combination did not show statistically significant superiority in antibiofilm activity compared to colistin alone. The time-kill assay confirmed the synergistic effect, showing a notable reduction in colony counts within 24 hours. These findings underscored the complexities of biofilm structures and their resistance mechanisms, highlighting the necessity for further research to optimize treatment outcomes.Conclusions:
This study provides a novel perspective on addressing antibiotic resistance in K. pneumoniae by evaluating the synergistic potential of the nisin-colistin combination. The findings contribute to the development of innovative therapeutic approaches to manage infections caused by this challenging pathogen.Keywords
Klebsiella pneumoniae Antibiotic Resistance Colistin Nisin Biofilm
1. Background
Klebsiella pneumoniae represents a significant adversary in clinical settings, recognized for its role as an opportunistic, nosocomial pathogen capable of causing a range of infectious conditions, including pneumonia, septicemia, and urinary tract infections (UTIs). The organism's increasing resistance to antibiotics, particularly within the Enterobacteriaceae family, highlights a critical global health challenge (1, 2). The emergence of carbapenem-resistant strains—carbapenems being the cornerstone antibiotics for treating multidrug-resistant K. pneumoniae infections—has intensified the search for alternative therapeutic options (3-5).
With the declining efficacy of carbapenems, polymyxins, especially colistin and polymyxin B, have been revisited as viable treatments for infections unresponsive to conventional antibiotics. However, the heightened clinical and agricultural use of Polymyxins has accelerated the dissemination of resistance mechanisms (6, 7). Further complicating the eradication of K. pneumoniae is its ability to form biofilms—structured bacterial communities adhering to surfaces and encased in a protective matrix. Biofilms not only promote bacterial persistence in the host by colonizing various anatomical sites but also hinder the penetration of antimicrobial agents, complicating treatment strategies (8).
In response to these challenges, recent research has explored the synergistic potential of combining Polymyxins with other antimicrobial agents, such as antimicrobial peptides, to enhance treatment outcomes (9). Among these peptides, nisin has gained considerable attention for its broad-spectrum antibacterial activity, particularly against gram-positive bacteria. Nisin functions by binding to lipid-II, a critical component in bacterial cell wall synthesis, disrupting membrane integrity and inhibiting peptidoglycan synthesis (10). While its efficacy against gram-positive pathogens is well-established (11), the outer membrane of Gram-negative bacteria typically restricts nisin's activity. Nonetheless, emerging evidence suggests that nisin, when combined with antibiotics, can exhibit synergistic effects against gram-negative organisms, including K. pneumoniae (12).
Promising research has demonstrated that combinations of nisin with ceftriaxone or cefotaxime enhance activity against Salmonella species (13). Additionally, in vitro studies have highlighted synergistic interactions between nisin and Polymyxin B against standard strains of pathogens such as Pseudomonas aeruginosa, Escherichia coli, and K. pneumoniae (14-16). Encouraging findings have also emerged from evaluations of nisin and polymyxin B against extensively drug-resistant (XDR) and pan-drug-resistant (PDR) clinical isolates of Acinetobacter baumannii (15, 17).
This background lays the foundation for investigating the synergistic potential of the nisin-colistin combination versus colistin alone in combating K. pneumoniae isolates, addressing a critical gap in the therapeutic strategies against gram-negative bacterial infections.
The primary aim of this study is to evaluate the synergistic effects of combining nisin with colistin against K. pneumoniae isolates. Specifically, the study seeks to determine whether this combination enhances antibacterial efficacy compared to colistin alone.
2. Objectives
Through comprehensive in vitro experimentation, this research aspires to offer novel insights into combating antibiotic resistance in K. pneumoniae, potentially paving the way for innovative therapeutic approaches to address infections caused by this challenging pathogen.
3. Methods
3.1. Bacterial Strains and Growth Conditions
Ten K. pneumoniae isolates were collected from hospitalized patients in Iran in August 2018. The isolates were obtained from various clinical specimens, including wounds, urine, blood, and tracheal aspirates. Species identification was conducted based on a series of biochemical tests, including SH2/indole/motility reactions (17), triple sugar iron (TSI) agar reactions, urease production on urea agar, growth on Simmons' citrate agar medium, the methyl red/voges-proskauer (MR/VP) test, and the ornithine decarboxylase (OD) test (18). Upon confirmation, the isolates were stored in tryptic soy broth (TSB) supplemented with 20% glycerol at -70°C. Out of the 52 confirmed isolates, ten were randomly selected for subsequent assays.
3.2. Nisin Preparation
Nisin powder, obtained from Sigma Aldrich (St. Louis, MO, USA), was dissolved in distilled water, and the resulting solution was stored at 4°C.
3.3. Antimicrobial Susceptibility Testing
The antimicrobial susceptibility of all K. pneumoniae isolates was determined using the Kirby-Bauer disk diffusion method on Cation-Adjusted Mueller Hinton agar (Merck, Germany). The results were interpreted according to the Clinical and Laboratory Standards Institute (CLSI) 2018 or Food and Drug Administration (FDA) breakpoints guidelines (17, 19). The antibiotics used for the antibiogram determination of the collected strains are listed in Table 1.
Antibiotic Susceptibility of Klebsiella pneumoniae Isolates a
Antibiotics | Sensitive | Intermediate | Resistant |
---|---|---|---|
Gentamicin (10 μg) | 1(10) | 1(10) | 8 (80) |
Pirlimycin (10 μg) | 0 (0) | 0 (0) | 10 (100) |
Levofloxacin (5 μg) | 1 (10) | 0 (0) | 9 (90) |
Ceftriaxone (30 μg) | 0 (0) | 0 (0) | 10 (100) |
Doripenem (10 μg) | 1 (10) | 0 (0) | 9 (90) |
Ampicillin (10 μg) | 0 (0) | 0 (0) | 10 (100) |
Cefepime (30 μg) | 0 (0) | 0 (0) | 10 (100) |
Minocycline (30 μg) | 5 (50) | 2 (20) | 3 (30) |
Azithromycin (30 μg) | 0 (0) | 1 (10) | 9 (90) |
Imipenem (10 μg) | 1 (10) | 0 (0) | 9 (90) |
Amikacin (30 μg) | 2 (20) | 0 (0) | 8 (80) |
Piperacillin-tazobactam (100/10 μg) | 1 (10) | 0 (0) | 9 (90) |
Ertapenem (10 μg) | 1 (10) | 0 (0) | 9 (90) |
Meropenem (10 μg) | 1 (10) | 0 (0) | 9 (90) |
Ciprofloxacin (5 μg) | 0 (0) | 0 (0) | 10 (100) |
Ceftazidime (30 μg) | 0 (0) | 1 (10) | 9 (90) |
3.4. Minimum Inhibitory Concentration () Assays
The minimum inhibitory concentrations (MICs) of nisin and colistin were determined in triplicate (with the mean value used) using the broth microdilution method in sterile 96-well polystyrene microplates with Muller-Hinton broth (MHB) medium, adhering to CLSI guidelines (20). Serial dilutions of nisin and colistin were prepared, starting at a concentration of 128 μg/mL, in a volume of 100 μL per well. The target strains were grown overnight under appropriate conditions, and the bacterial turbidity was adjusted to a 0.5 McFarland standard, equivalent to approximately 1.5 × 10⁸ CFU/mL. The adjusted bacterial suspensions were then diluted 1:20 in MHB, and 10 μL of the diluted suspension (5 × 10⁴ CFU/well) was added to each well, followed by incubation for 18 hours at 35°C.
Minimum inhibitory concentrations were determined as the first clear well with no visible bacterial growth. Quality control was conducted using K. pneumoniae ATCC 700603, following CLSI guidelines (21).
3.5. Synergistic Activity Testing
To evaluate the synergistic activity between nisin and colistin, wells were filled with 50 μL of each antimicrobial at equal concentrations, starting at 128 μg/mL. Serial dilutions were prepared, and the appropriate bacterial suspension, as previously described, was added to each well. The first clear well with no visible bacterial growth was recorded and compared to the MIC of each antimicrobial tested individually.
3.6. Biofilm Formation Assay
The antibiofilm activity of nisin and colistin, both individually and in combination, was assessed using the microtiter plate method at a concentration of 1/2 MIC as determined previously (22). Log-phase cells were introduced to achieve a starting inoculum of 105 CFU/mL, with all wells seeded in triplicate for a single isolate. The plates were incubated for 18 hours at 37°C, followed by three washes with phosphate-buffered saline (PBS). After fixation with methanol for 15 minutes, the plates were stained with 1% crystal violet, rinsed with PBS, and acetic acid was added to solubilize the dye. The absorbance was measured at 570 nm using the Synergy HTX Multi-Mode Reader (Agilent BioTek, USA). Data were averaged from triplicate measurements. The cut-off OD (ODc) was defined as three standard deviations above the mean OD of the negative control. Based on this, isolates were classified into four categories: OD ≤ ODc (non-adherent), ODc < OD ≤ 2 × ODc (weakly adherent), 2 × ODc < OD ≤ 4 × ODc (moderately adherent), and 4 × ODc < OD (strongly adherent).
3.7. Time-Kill Assay
To evaluate the antibacterial activity of the nisin-colistin combination compared to colistin alone against K. pneumoniae isolates, a time-kill assay was performed over a 24-hour period. An overnight culture in Mueller-Hinton broth, adjusted to an approximate concentration of 105 CFU/mL, served as the starting inoculum in 10 mL universal tubes (23). Concentrations equal to the MIC for nisin and colistin were added to the broth. The cultures were incubated at 37°C with continuous agitation for 24 hours. At intervals of 0, 2, 4, 6, and 24 hours post-inoculation, 100 μL samples were collected and plated onto Mueller-Hinton agar. The plates were incubated at 37°C for 24 hours, after which colony counts were recorded (24). Time-kill curves (CFU/mL vs. time) were generated using GraphPad Prism 9 software.
4. Results
4.1. Antimicrobial Susceptibility Testing
The antimicrobial susceptibility profiles of the 10 isolates were determined using the disk diffusion agar method, with the results summarized in Table 1. All isolates, except for K72, which was classified as multidrug-resistant (MDR), exhibited resistance to meropenem, imipenem, and ertapenem, categorizing them as XDR isolates.
4.2. Antibacterial and Biofilm Eradication Assay
The results from the microdilution broth method are summarized in Table 2. All isolates demonstrated resistance to nisin at concentrations exceeding 1024 μg/mL. However, combination therapy with colistin in isolates K40, K41, and K51 significantly reduced the MIC of colistin by half. Nevertheless, when assessing quantitative biofilm determination using the microtiter assay, the nisin-colistin combination did not show statistically significant superiority in antibiofilm activity compared to colistin alone (Table 3).
Minimum Inhibitory Concentration of Nisin and Colistin Alone or in Combination
Strains | Nisin MIC (μg/mL) | Colistin MIC (μg/mL) | Nisin-Colistin Combination (μg/mL) | Reduction in Colistin MIC |
---|---|---|---|---|
K2 | > 1024 | 2 | 2/2 | - |
K3 | > 1024 | 4 | 4/4 | - |
K4 | > 1024 | 4 | 4/4 | - |
K40 | > 1024 | 4 | 2/2 | + |
K41 | > 1024 | 4 | 0.5/0.5 | + |
K45 | > 1024 | 64 | 64/64 | - |
K51 | > 1024 | 128 | 64/64 | + |
K56 | > 1024 | 64 | 64/64 | - |
K58 | > 1024 | 64 | 64/64 | - |
K72 | > 1024 | 0.5 | 0.5/0.5 | - |
Biofilm Formation Assay by Microtiter in Four Conditions of Antibacterial
Strains | Control | Nisin | Colistin | Nisin + Colistin |
---|---|---|---|---|
K2 | +1 | +1 | +1 | +1 |
K3 | +3 | +3 | +3 | +3 |
K4 | +3 | +3 | +3 | +3 |
K40 | +2 | +2 | +2 | +2 |
K41 | +3 | +3 | +3 | +3 |
K45 | +3 | +3 | +1 | +1 |
K51 | +2 | +1 | +1 | +1 |
K56 | +3 | +3 | +1 | +1 |
K58 | +3 | +3 | +1 | +1 |
K72 | +1 | +1 | +1 | +1 |
4.4 Time-Kill Assay
The time-kill assay was conducted to evaluate the efficacy of the nisin-colistin combination against isolates K58 and K72, with each agent used at a concentration equal to the MIC. The results, as shown in Figure 1, demonstrated that the nisin-colistin combination exhibited a synergistic effect, resulting in a significant reduction in colony counts over 24 hours for both isolates.
Time-kill assay curve and plates for K58 (A) and K72 (B) after exposure to antibacterial agents compared to control. The results demonstrated that the nisin-colistin combination exhibited a synergistic effect, leading to a significant reduction in colony counts over 24 hours for both isolates
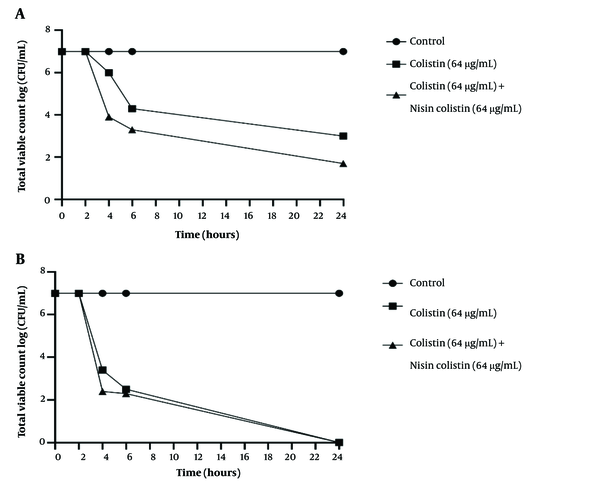
5. Discussion
The widespread emergence of antibiotic resistance has become a critical global concern in recent years. One organism of particular concern is K. pneumoniae, which has demonstrated a remarkable ability to develop resistance to carbapenems, a class of antibiotics often considered a last resort for treating severe infections (19).
Combination therapy may prove effective when existing antibiotics are paired with safe, antibacterial agents like nisin. Nisin binds to lipid II, a vital component of the bacterial cell wall, disrupting its structural integrity. This interaction compromises the cell wall's ability to form and maintain an effective barrier, rendering the inner membrane more accessible (25).
The efficacy of nisin against gram-negative bacteria has been demonstrated in previous studies, both as a standalone agent and in combination with colistin or polymyxin B. In this study, we investigated the synergistic effects of combining nisin with colistin on XDR and MDR clinical isolates of K. pneumoniae. A comparative analysis between the singular use of antibacterial agents and their combined application revealed a significant reduction in the MIC of colistin when combined with nisin in select isolates, highlighting a clear synergistic effect.
Consistent with our findings, nisin has shown limited inhibitory effects on E. coli RR1 and Salmonella choleraesuis ATCC 14028, even at high concentrations (26). Similarly, in Singh’s research, no inhibition of growth was observed at concentrations up to 400 μg/mL against Salmonella enterica serovar Typhimurium NCTC74. However, when combined with colistin, nisin significantly enhanced antibiotic efficacy (13). This lack of standalone activity is commonly attributed to the gram-negative cell wall, which acts as a barrier preventing nisin from accessing the inner membrane (18). Colistin disrupts this outer membrane, allowing nisin to penetrate and interact with the cell wall. This disruption enhances the permeability of nisin, resulting in increased antimicrobial activity (20). Thus, colistin serves as an effective adjuvant for amplifying the antimicrobial effects of nisin.
Our observations are consistent with those of Jahangiri et al., who reported synergistic effects of nisin combined with colistin against P. aeruginosa and A. baumannii (21).
However, our results diverge in biofilm eradication, where the nisin-colistin combination did not surpass the efficacy of colistin alone in our study. This discrepancy could stem from differences in experimental conditions, such as the concentration of nisin, the duration of treatment, and the bacterial strain used. Additionally, it is possible that the concentration of colistin employed in other studies was more effective at eradicating biofilms than the concentration used in our study (21). The observation that all isolates demonstrated resistance to nisin at concentrations exceeding 1024 μg/mL, yet exhibited reduced MICs for colistin when combined with nisin in certain isolates, suggests a partial synergistic effect. This aligns with prior research indicating that combining antimicrobial peptides (AMPs) like nisin with antibiotics can enhance antibacterial efficacy (14, 22).
The intricacies of biofilm formation, influenced by bacterial species and their inherent resistance mechanisms, contribute to the nuanced response observed in our study. This finding is consistent with the work of Jamal et al., who emphasized the multifaceted nature of biofilm dynamics and the significant challenges associated with eradicating biofilms in bacterial infections (23).
While some studies have reported enhanced effects of AMP-antibiotic combinations against gram-negative bacteria, others have highlighted challenges in achieving consistent results, particularly against biofilms (24, 27). Variations in outcomes could be attributed to several factors, including differences in the genetic makeup of bacterial strains, experimental conditions, and the specific endpoints measured. The varied efficacy of the nisin-colistin combination in biofilm eradication assays underscores the complexity of biofilm structures and their associated resistance mechanisms. This suggests the necessity of further studies to deepen our understanding of biofilm dynamics and to explore additional strategies for improving treatment outcomes.
Furthermore, our time-kill assay demonstrated a significant reduction in colony counts over 24 hours for isolates treated with the nisin-colistin combination. This finding is consistent with reports by other researchers, which support the enhanced bactericidal activity of nisin in combination with polymyxin B against gram-negative bacteria (14, 15, 28). Our results align with the concept that combining antimicrobial peptides (AMPs) with traditional antibiotics can disrupt bacterial cells more effectively than either agent alone, particularly by increasing membrane permeability and facilitating the antibiotic's entry into bacterial cells (29).
Comparisons with published studies highlight the diverse outcomes of nisin-antibiotic synergy against different bacterial species. The variations in these outcomes across studies underscore the importance of considering experimental conditions, bacterial strains, and the specific combinations of nisin with different antibiotics. This variability highlights the need for further research to explore the full potential of nisin-antibiotic combinations.
One limitation of this study is its focus on isolates from specific hospitals in Iran, which may affect the generalizability of the findings. Future research could include a broader geographic sampling to evaluate the efficacy of the nisin-colistin combination across diverse K. pneumoniae strains globally. Additionally, the varied efficacy of the nisin-colistin combination in biofilm eradication assays emphasizes the complexity of biofilm structures and their associated resistance mechanisms. Further studies are needed to better understand biofilm dynamics and to explore additional strategies for enhancing treatment outcomes.
It is also important to note that our research primarily focuses on in vitro analyses. Translating these findings into clinical success will require in vivo studies and clinical trials to ascertain the safety, optimal dosages, and real-world efficacy of the nisin-colistin combination. Moreover, a deeper understanding of the dynamics of synergistic interactions could pave the way for developing tailored strategies to combat antibiotic-resistant bacteria more effectively.
5.1. Conclusions
This study provides valuable insights into the potential of nisin-antibiotic combinations for combating drug-resistant K. pneumoniae, contributing to the growing body of evidence supporting their efficacy. While our findings align with those of certain published studies, the observed variations in outcomes highlight the necessity for tailored approaches in addressing multidrug-resistant infections. Further research employing standardized methodologies and incorporating a broader range of bacterial strains is essential to better elucidate the full spectrum of nisin-antibiotic interactions against K. pneumoniae. Such efforts will enhance the translational potential of these findings and inform the development of more effective strategies for managing drug-resistant bacterial infections.
Acknowledgements
References
-
1.
Pitout JD, Laupland KB. Extended-spectrum beta-lactamase-producing Enterobacteriaceae: an emerging public-health concern. Lancet Infect Dis. 2008;8(3):159-66. [PubMed ID: 18291338]. https://doi.org/10.1016/S1473-3099(08)70041-0.
-
2.
Tzouvelekis LS, Markogiannakis A, Psichogiou M, Tassios PT, Daikos GL. Carbapenemases in Klebsiella pneumoniae and other Enterobacteriaceae: an evolving crisis of global dimensions. Clin Microbiol Rev. 2012;25(4):682-707. [PubMed ID: 23034326]. [PubMed Central ID: PMC3485753]. https://doi.org/10.1128/CMR.05035-11.
-
3.
Nordmann P, Dortet L, Poirel L. Carbapenem resistance in Enterobacteriaceae: here is the storm!. Trends Mol Med. 2012;18(5):263-72. [PubMed ID: 22480775]. https://doi.org/10.1016/j.molmed.2012.03.003.
-
4.
Sameni F, Ghazi M, Dadashi M, Bostanshirin N, Al-Dahmoshi HO, Khosravi-Dehaghi N, et al. [Global distribution, genotypes and prevalent sequence types of colistin-resistant Klebsiella pneumoniae isolated from clinical samples; A systematic review]. Gene Rep. 2022;28. FA. https://doi.org/10.1016/j.genrep.2022.101635.
-
5.
Shivaee A, Shahbazi S, Soltani A, Ahadi E. [Evaluation of the prevalence of broad-spectrum beta-lactamases (ESBLs) and carbapenemase genes in Klebsiella pneumoniae strains isolated from burn wounds in patients referred to Shahid Motahari Hospital in Tehran]. Med Sci J Islamic Azad Univ. 2019;29(3):232-9. FA. https://doi.org/10.29252/iau.29.3.232.
-
6.
Nasiri MJ, Mirsaeidi M, Mousavi SMJ, Arshadi M, Fardsanei F, Deihim B, et al. Prevalence and Mechanisms of Carbapenem Resistance in Klebsiella pneumoniae and Escherichia coli: A Systematic Review and Meta-Analysis of Cross-Sectional Studies from Iran. Microb Drug Resist. 2020;26(12):1491-502. [PubMed ID: 32348701]. https://doi.org/10.1089/mdr.2019.0440.
-
7.
Olaitan AO, Morand S, Rolain JM. Mechanisms of polymyxin resistance: acquired and intrinsic resistance in bacteria. Front Microbiol. 2014;5:643. [PubMed ID: 25505462]. [PubMed Central ID: PMC4244539]. https://doi.org/10.3389/fmicb.2014.00643.
-
8.
Hoiby N, Bjarnsholt T, Givskov M, Molin S, Ciofu O. Antibiotic resistance of bacterial biofilms. Int J Antimicrob Agents. 2010;35(4):322-32. [PubMed ID: 20149602]. https://doi.org/10.1016/j.ijantimicag.2009.12.011.
-
9.
Zhu Y, Hao W, Wang X, Ouyang J, Deng X, Yu H, et al. Antimicrobial peptides, conventional antibiotics, and their synergistic utility for the treatment of drug-resistant infections. Med Res Rev. 2022;42(4):1377-422. [PubMed ID: 34984699]. https://doi.org/10.1002/med.21879.
-
10.
Breukink E, de Kruijff B. Lipid II as a target for antibiotics. Nat Rev Drug Discov. 2006;5(4):321-32. [PubMed ID: 16531990]. https://doi.org/10.1038/nrd2004.
-
11.
Shivaee A, Rajabi S, Farahani HE, Imani Fooladi AA. Effect of sub-lethal doses of nisin on Staphylococcus aureus toxin production and biofilm formation. Toxicon. 2021;197:1-5. [PubMed ID: 33838179]. https://doi.org/10.1016/j.toxicon.2021.03.018.
-
12.
Field D, Begley M, O'Connor PM, Daly KM, Hugenholtz F, Cotter PD, et al. Bioengineered nisin A derivatives with enhanced activity against both Gram positive and Gram negative pathogens. PLoS One. 2012;7(10). e46884. [PubMed ID: 23056510]. [PubMed Central ID: PMC3466204]. https://doi.org/10.1371/journal.pone.0046884.
-
13.
Singh AP, Prabha V, Rishi P. Value addition in the efficacy of conventional antibiotics by Nisin against Salmonella. PLoS One. 2013;8(10). e76844. [PubMed ID: 24116175]. [PubMed Central ID: PMC3792866]. https://doi.org/10.1371/journal.pone.0076844.
-
14.
Field D, Seisling N, Cotter PD, Ross RP, Hill C. Synergistic Nisin-Polymyxin Combinations for the Control of Pseudomonas Biofilm Formation. Front Microbiol. 2016;7:1713. [PubMed ID: 27833601]. [PubMed Central ID: PMC5080341]. https://doi.org/10.3389/fmicb.2016.01713.
-
15.
Thomas VM, Brown RM, Ashcraft DS, Pankey GA. Synergistic effect between nisin and polymyxin B against pandrug-resistant and extensively drug-resistant Acinetobacter baumannii. Int J Antimicrob Agents. 2019;53(5):663-8. [PubMed ID: 30880230]. https://doi.org/10.1016/j.ijantimicag.2019.03.009.
-
16.
Chi H, Holo H. Synergistic Antimicrobial Activity Between the Broad Spectrum Bacteriocin Garvicin KS and Nisin, Farnesol and Polymyxin B Against Gram-Positive and Gram-Negative Bacteria. Curr Microbiol. 2018;75(3):272-7. [PubMed ID: 29058043]. [PubMed Central ID: PMC5809525]. https://doi.org/10.1007/s00284-017-1375-y.
-
17.
Karyne R, Curty Lechuga G, Almeida Souza AL, Rangel da Silva Carvalho JP, Simoes Villas Boas MH, De Simone SG. Pan-Drug Resistant Acinetobacter baumannii, but Not Other Strains, Are Resistant to the Bee Venom Peptide Mellitin. Antibiotics (Basel). 2020;9(4). [PubMed ID: 32295149]. [PubMed Central ID: PMC7235889]. https://doi.org/10.3390/antibiotics9040178.
-
18.
Li Q, Montalban-Lopez M, Kuipers OP. Increasing the Antimicrobial Activity of Nisin-Based Lantibiotics against Gram-Negative Pathogens. Appl Environ Microbiol. 2018;84(12). [PubMed ID: 29625984]. [PubMed Central ID: PMC5981070]. https://doi.org/10.1128/AEM.00052-18.
-
19.
Chung PY. The emerging problems of Klebsiella pneumoniae infections: carbapenem resistance and biofilm formation. FEMS Microbiol Lett. 2016;363(20). [PubMed ID: 27664057]. https://doi.org/10.1093/femsle/fnw219.
-
20.
Li Q, Cebrian R, Montalban-Lopez M, Ren H, Wu W, Kuipers OP. Outer-membrane-acting peptides and lipid II-targeting antibiotics cooperatively kill Gram-negative pathogens. Commun Biol. 2021;4(1):31. [PubMed ID: 33398076]. [PubMed Central ID: PMC7782785]. https://doi.org/10.1038/s42003-020-01511-1.
-
21.
Jahangiri A, Neshani A, Mirhosseini SA, Ghazvini K, Zare H, Sedighian H. Synergistic effect of two antimicrobial peptides, Nisin and P10 with conventional antibiotics against extensively drug-resistant Acinetobacter baumannii and colistin-resistant Pseudomonas aeruginosa isolates. Microb Pathog. 2021;150:104700. [PubMed ID: 33346078]. https://doi.org/10.1016/j.micpath.2020.104700.
-
22.
Jorge P, Grzywacz D, Kamysz W, Lourenco A, Pereira MO. Searching for new strategies against biofilm infections: Colistin-AMP combinations against Pseudomonas aeruginosa and Staphylococcus aureus single- and double-species biofilms. PLoS One. 2017;12(3). e0174654. [PubMed ID: 28355248]. [PubMed Central ID: PMC5371341]. https://doi.org/10.1371/journal.pone.0174654.
-
23.
Jamal M, Ahmad W, Andleeb S, Jalil F, Imran M, Nawaz MA, et al. Bacterial biofilm and associated infections. J Chin Med Assoc. 2018;81(1):7-11. [PubMed ID: 29042186]. https://doi.org/10.1016/j.jcma.2017.07.012.
-
24.
Batoni G, Maisetta G, Brancatisano FL, Esin S, Campa M. Use of antimicrobial peptides against microbial biofilms: advantages and limits. Curr Med Chem. 2011;18(2):256-79. [PubMed ID: 21110801]. https://doi.org/10.2174/092986711794088399.
-
25.
t Hart P, Oppedijk SF, Breukink E, Martin NI. New Insights into Nisin's Antibacterial Mechanism Revealed by Binding Studies with Synthetic Lipid II Analogues. Biochemistry. 2016;55(1):232-7. [PubMed ID: 26653142]. https://doi.org/10.1021/acs.biochem.5b01173.
-
26.
Naghmouchi K, Baah J, Hober D, Jouy E, Rubrecht C, Sane F, et al. Synergistic effect between colistin and bacteriocins in controlling Gram-negative pathogens and their potential to reduce antibiotic toxicity in mammalian epithelial cells. Antimicrob Agents Chemother. 2013;57(6):2719-25. [PubMed ID: 23571533]. [PubMed Central ID: PMC3716138]. https://doi.org/10.1128/AAC.02328-12.
-
27.
Grassi L, Maisetta G, Esin S, Batoni G. Combination Strategies to Enhance the Efficacy of Antimicrobial Peptides against Bacterial Biofilms. Front Microbiol. 2017;8:2409. [PubMed ID: 29375486]. [PubMed Central ID: PMC5770624]. https://doi.org/10.3389/fmicb.2017.02409.
-
28.
Naghmouchi K, Drider D, Baah J, Teather R. Nisin A and Polymyxin B as Synergistic Inhibitors of Gram-positive and Gram-negative Bacteria. Probiotics Antimicrob Proteins. 2010;2(2):98-103. [PubMed ID: 26781118]. https://doi.org/10.1007/s12602-009-9033-8.
-
29.
Brogden KA. Antimicrobial peptides: pore formers or metabolic inhibitors in bacteria? Nat Rev Microbiol. 2005;3(3):238-50. [PubMed ID: 15703760]. https://doi.org/10.1038/nrmicro1098.