Abstract
Background:
Although Malassezia genusare part of the skin normal flora, under certain conditions, they become pathogenic. Catheter-related fungemia, caused by Malassezia, which is associated with biofilm formation, is considered a nosocomial infection.Objectives:
The aim of this study was to evaluate the ability of Malassezia globosa and Malassezia restricta in biofilm formation.Methods:
Biofilm formation was carried out using catheter segments in 12-well plates. Results were measured using 3-(4, 5- dimethylthiazol-2-yl)-2, 5-diphenyl-tetrazolium bromide (MTT) colorimetric assay in 96-well plates. The data was analyzed using univariate Analysis of Variance (ANOVA) or Repeated Measures ANOVA. P values of ≤ 0.05 were considered statistically significant. Statistical analyses were performed using IBM SPSS Statistics version 22.0 software.Results:
Both M. globosa and M. restricta species were able to form biofilms in vitro. Malassezia restricta was more capable than M. globosa to form biofilms, yet, significant differences were not observed (P = 0.192).Conclusions:
Over time, Malassezia biofilms matured. Due to the above species ability in forming biofilm, they could play an important role in fungemia that should be considered in therapeutic procedures.Keywords
1. Background
Malassezia biofilm is produced by a community of these bacteria, alone or mixed with other microorganisms, which are attached to the living and non-living surfaces and are difficult to separate from surfaces. This structure has physical and chemical resistance, and resistance to antimicrobial agents is almost 2000 times higher compared with planktonic cells. Biofilm of Malassezia is a structure that is organized as single-layer or multi-layer of blastoconidia. Biofilms, with the ability of producing an extracellular matrix, acts as a barrier against the influence of drugs (1-5). Although Malassezia genus is part of the skin normal flora, under certain conditions, becomes pathogenic and in the recent years has been reported as an emerging pathogen (1, 6-9). The ability of these fungi in colonization on the catheter is associated with biofilm formation. Catheters are frequently used in new medical devices, especially at the Intensive Care Unit (ICU) and their use may lead to local or systemic infection (1, 5, 10-12). Since nosocomial infections effect approximately 10% to 30% of patients at the ICU and are associated with significant mortality, it should be noted that a significant proportion of these infections are due to biofilm formation (5, 10-12); however, fungal biofilms have been studied less than bacterial biofilms. Most studies are about Candida (13-16), however, there have been only a few studies on Malassezia biofilms (1, 4, 5, 13, 17).
2. Objectives
The aim of this study was to evaluate the ability of Malassezia globosa and Malassezia restricta species in biofilm formation.
3. Methods
After preparing the standard species of M. restricta (CBS 7877) and M. globosa (CBS 7966) from the Institute (Centraalbureau voor Schimmelcultures, Utrecht,The Netherlands), the study was conducted at the Medical mycology laboratory, faculty of Medical Sciences, Tarbiat Modares University.
3.1. Evaluation of Biofilm Formation
Catheter segments of Certofix® Mono V 420 central venous catheter (B. Braun, Milano, Italy) with 1 × 1 cm dimensions were prepared in 12-well plates (Nalge Nunc International, NY, USA). After suspension of the Malassezia yeast in a concentration of 106 CFU/mL Phosphate Buffered Saline (PBS, PH = 7.2 - 7.4) biofilm was formed using the medium modified Dixon broth (made of 3.6 g malt extract (Merck, Germany), 0.6 g mycological peptone, 2 g dried beef bile (Sigma, UK), 1 mL Tween 40 (Merck, Germany), 0.2 mL glycerol (Merck, Germany), 0.2 mL oleic acid (Merck, Germany), 5 mg chloramphenicol, 50 mg cycloheximide and 100 mL distilled water) (13). The test wells were selected and catheter segments were divided in doubled form and incubated in fetal bovine serum (FBS) for 24 hours (h) at 37°C on a rocker. Next, catheter segments were placed in new 12-well plates and 4 mL of culture medium mDixon broth containing Malassezia yeast at a concentration of 106 CFU/mL, was poured in each well to submerge catheter segments and then they were incubated in a 75 rpm (round per minute) shaking incubator for 24 hours at 32°C for cell attachment to the catheter surface. After a period of time, to separate the loose and non-binding cells (planktonic cells), catheter segments were washed with PBS and 4 mL of culture medium mDixon broth containing 100 mM of glucose was added to the wells (4, 18). After incubation at 32°C for 48, 96, and 144 hours in a 75 rpm shaking incubator (5), catheter segments were removed from wells and formed biofilms on the surfaces were scraped by a plastic spatula (cell scraping) and were poured in 96-well micro-plates (Nalge Nunc International, NY, USA). After addition of 50 µL of distilled water and 10 µL MTT (Sigma Chemical Co., St Louis, MO, USA) at a concentration of 5 mg/mL, they were incubated for 4 hours at 32°C. Finally, by adding 50 µL of Dimethyl Sulfoxide (DMSO) solution to all wells and incubation for 10 minutes at 32°C, the percentage of attached cells on the surface of the catheter segments was measured by using the enzyme linked immunosorbent assay (ELISA) absorbance reader (Stat Fax 2000, USA) at 540 nm. In this method, the well containing catheter segments with mDixon broth medium and without yeast cell suspension was used as a negative control.
3.2. Evaluation of Biofilm Formation on the Catheter Segments Through Scanning Electron Microscopy (SEM)
Two catheter segments with biofilms formed on them after 96 hours were studied with a scanning electron microscope according to a previously described method (4) (Leo, Cambridge, UK).
3.3. Statistical Analysis
Normality of data was assessed using the One-Sample Kolmogorov-Smirnov Test (with Lilliefors Significance Correction). After collecting and classifying the data, they were analyzed using univariate analysis of variance (ANOVA) or repeated measures ANOVA. A value of P ≤ 0.05 was considered statistically significant. Statistical analyses were performed using IBM SPSS Statistics version 22 software (19).
4. Results
Both M. globosa and M. restricta species were able to form in vitro biofilms. At three time points, Malassezia biofilm matured and had a linier increase. Optical density (OD) results of biofilm formation after periods of 48, 96 and 144 hours are shown in Figure 1. Malassezia restricta was more capable than M. globosa to form a biofilm, yet, between them, a significant difference was not observed (P = 0.192). Figure 2 shows the SEM results of two M. globosa and M. restricta species.
Comparison of the Ability to Form Biofilms by Malassezia restricta and Malassezia globosa at Three Time Points
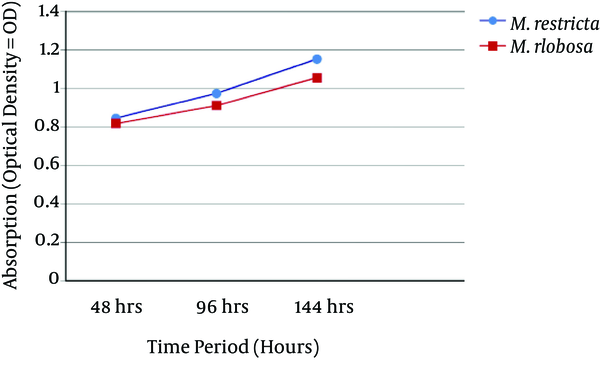
Scanning Electron Microscopy Showing Biofilm Structures of A, M. globosa and B, M. restricta
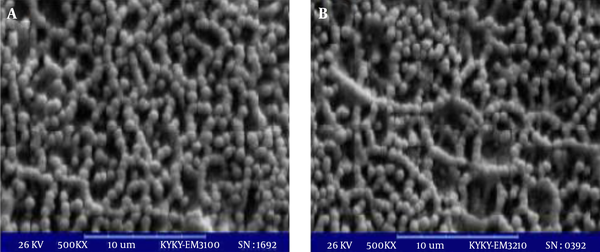
5. Discussion
Catheter-related fungemia caused by Malassezia is reported in premature infants, immune compromised children, adults with parenteral nutrition, and individuals with immune deficiency (13). Reports show the role of Malassezia in catheter-related biofilm formation and its pathogenicity in etiology of infections, such as fungemia (5, 13, 20-22). In this study, M. globosa and M. restricta species were used to evaluate the in vitro ability of biofilm formation on catheter surfaces.
One of the common and standard methods to evaluate biofilm formation is the assessment of cells viability after treatment with antifungal drugs. A vital MTT dye could be used for this purpose. The MTT test is a semi-quantitative colorimetric method to measure cell viability (23, 24). This method was used in the current study.
In this study, both M. globosa and M. restricta species were able to form in vitro biofilms. Malassezia restricta was more capable than M. globosa to form a biofilm and after periods of 48, 96 and 144 hours, the OD results of M. restricta wells were more than M. globosa wells. However, between them, significant differences were not observed (P = 0.192, Figure 1). This study shows that the two M. globosa and M. restricta species could form in vitro biofilm, similar to M. pachydermatis species (4, 5). According to this study and other laboratory and clinical studies (4, 5, 13), it has been concluded that Malassezia species are able to form biofilms in two in vivo and in vitro models and give rise to nosocomial infections in children and adults.
Biofilm formation by microorganisms, including fungi varies with time (5, 10, 18, 25). In this study, biofilm mass (OD value) had a linear increase as time increased, which is in accordance with the study of Cannizzo (5). In comparison with mature biofilms of Candida that are formed within 48 hours, the Malassezia biofilm requires 4 days to mature and the structure of Malassezia biofilm is simpler than Candida. Attachment and nutritional properties of Malassezia may be the cause of this difference (5).
There are a few studies about biofilm formation of Malassezia species and reports are often about M. pachydermatis. However, there is not enough information available regarding other species. In this study, to evaluate biofilm formation, two M. globosa and M. restricta species were used for the pilot study. These species were standard strains and in terms of the ability to form biofilms (OD value) could be different from results of clinical isolates. According to previous studies, most of the isolates (95%), regardless of the area of isolation being a skin lesion or normal skin, were capable to form biofilms, yet their quantity was variable among different strains (4, 5). Also, genotypic studies have shown that the ability to form biofilms and their quantity could be variable among different genotypes (4). Strain dependence of biofilm formation that has been previously suggested for Candida, could be due to physiological and pathological characteristics of yeast and in the case of Malassezia could be dependent on phospholipase activity (4). On the other hand, clinically, Malassezia colonization on the catheter could vary in different wards of the hospital (13). Therefore, due to differences in the ability and quantity of biofilm formation among Malassezia strains and genotypes, although, the in vitro model conditions of biofilm formation are not very different from in vivo situation (26), future comprehensive studies on clinical isolates of Malassezia with more samples are suggested to determine whether colonization and biofilm formation is related to clinical findings of patients.
5.1. Conclusion
Both M. globosa and M. restricta species were able to form in vitro biofilms. Over time, Malassezia biofilms had matured. Due to the two above-mentioned species ability in forming biofilms, they could play an important role in fungemia and this should be considered in therapeutic procedures.
Acknowledgements
References
-
1.
Figueredo LA, Cafarchia C, Otranto D. Antifungal susceptibility of Malassezia pachydermatis biofilm. Med Mycol. 2013;51(8):863-7. [PubMed ID: 23834283]. https://doi.org/10.3109/13693786.2013.805440.
-
2.
Blankenship JR, Mitchell AP. How to build a biofilm: a fungal perspective. Curr Opin Microbiol. 2006;9(6):588-94. [PubMed ID: 17055772]. https://doi.org/10.1016/j.mib.2006.10.003.
-
3.
Chandra J, Zhou G, Ghannoum MA. Fungal biofilms and antimycotics. Curr Drug Targets. 2005;6(8):887-94. [PubMed ID: 16375672]. https://doi.org/10.2174/138945005774912762.
-
4.
Figueredo LA, Cafarchia C, Desantis S, Otranto D. Biofilm formation of Malassezia pachydermatis from dogs. Vet Microbiol. 2012;160(1-2):126-31. [PubMed ID: 22682201]. https://doi.org/10.1016/j.vetmic.2012.05.012.
-
5.
Cannizzo FT, Eraso E, Ezkurra PA, Villar-Vidal M, Bollo E, Castella G, et al. Biofilm development by clinical isolates of Malassezia pachydermatis. Med Mycol. 2007;45(4):357-61. [PubMed ID: 17510859]. https://doi.org/10.1080/13693780701225767.
-
6.
Larocco M, Dorenbaum A, Robinson A, Pickering LK. Recovery of Malassezia pachydermatis from eight infants in a neonatal intensive care nursery: clinical and laboratory features. Pediatr Infect Dis J. 1988;7(6):398-401. [PubMed ID: 3134645]. https://doi.org/10.1097/00006454-198806000-00006.
-
7.
Mickelsen PA, Viano-Paulson MC, Stevens DA, Diaz PS. Clinical and microbiological features of infection with Malassezia pachydermatis in high-risk infants. J Infect Dis. 1988;157(6):1163-8. [PubMed ID: 3373021]. https://doi.org/10.1093/infdis/157.6.1163.
-
8.
Welbel SF, McNeil MM, Pramanik A, Silberman R, Oberle AD, Midgley G, et al. Nosocomial Malassezia pachydermatis bloodstream infections in a neonatal intensive care unit. Pediatr Infect Dis J. 1994;13(2):104-8. [PubMed ID: 8190533]. https://doi.org/10.1097/00006454-199402000-00005.
-
9.
Chryssanthou E, Broberger U, Petrini B. Malassezia pachydermatis fungaemia in a neonatal intensive care unit. Acta Paediatr. 2001;90(3):323-7. [PubMed ID: 11332175]. https://doi.org/10.1080/080352501300067712.
-
10.
Donlan RM, Costerton JW. Biofilms: survival mechanisms of clinically relevant microorganisms. Clin Microbiol Rev. 2002;15(2):167-93. [PubMed ID: 11932229]. https://doi.org/10.1128/CMR.15.2.167-193.2002.
-
11.
Douglas LJ. Medical importance of biofilms in Candida infections. Rev Iberoam Micol. 2002;19(3):139-43. [PubMed ID: 12825991].
-
12.
Lopez-Ribot JL. Candida albicans biofilms: more than filamentation. Curr Biol. 2005;15(12):R453-5. [PubMed ID: 15964263]. https://doi.org/10.1016/j.cub.2005.06.020.
-
13.
Curvale-Fauchet N, Botterel F, Legrand P, Guillot J, Bretagne S. Frequency of intravascular catheter colonization by Malassezia spp. in adult patients. Mycoses. 2004;47(11-12):491-4. [PubMed ID: 15601455]. https://doi.org/10.1111/j.1439-0507.2004.01047.x.
-
14.
Chandra J, Mukherjee PK, Leidich SD, Faddoul FF, Hoyer LL, Douglas LJ, et al. Antifungal resistance of candidal biofilms formed on denture acrylic in vitro. J Dent Res. 2001;80(3):903-8. [PubMed ID: 11379893]. https://doi.org/10.1177/00220345010800031101.
-
15.
Rajendran R, May A, Sherry L, Kean R, Williams C, Jones BL, et al. Integrating Candida albicans metabolism with biofilm heterogeneity by transcriptome mapping. Sci Rep. 2016;6:35436. [PubMed ID: 27765942]. https://doi.org/10.1038/srep35436.
-
16.
Zarei Mahmoudabadi A, Zarrin M, Kiasat N. Biofilm Formation and Susceptibility to Amphotericin B and Fluconazole in Candida albicans. Jundishapur J Microbiol. 2014;7(7). e17105. [PubMed ID: 25368806]. https://doi.org/10.5812/jjm.17105.
-
17.
Kuhn DM, Chandra J, Mukherjee PK, Ghannoum MA. Comparison of biofilms formed by Candida albicans and Candida parapsilosis on bioprosthetic surfaces. Infect Immun. 2002;70(2):878-88. [PubMed ID: 11796623]. https://doi.org/10.1128/IAI.70.2.878-888.2002.
-
18.
Chandra J, Kuhn DM, Mukherjee PK, Hoyer LL, McCormick T, Ghannoum MA. Biofilm formation by the fungal pathogen Candida albicans: development, architecture, and drug resistance. J Bacteriol. 2001;183(18):5385-94. [PubMed ID: 11514524].
-
19.
Released I. IBM SPSS Statistics for Windows. 20. Armonk, NY: IBM Corp; 2013.
-
20.
Weiss SJ, Schoch PE, Cunha BA. Malassezia furfur fungemia associated with central venous catheter lipid emulsion infusion. Heart Lung. 1991;20(1):87-90. [PubMed ID: 1988397].
-
21.
Shparago NI, Bruno PP, Bennett J. Systemic Malassezia furfur infection in an adult receiving total parenteral nutrition. J Am Osteopath Assoc. 1995;95(6):375-7. [PubMed ID: 7615409].
-
22.
Iatta R, Cafarchia C, Cuna T, Montagna O, Laforgia N, Gentile O, et al. Bloodstream infections by Malassezia and Candida species in critical care patients. Med Mycol. 2014;52(3):264-9. [PubMed ID: 24576998]. https://doi.org/10.1093/mmy/myt004.
-
23.
Plumb JA. Cell sensitivity assays: the MTT assay. Cancer Cell Culture: Methods and Protocols. 2004. p. 165-9.
-
24.
Fischer J, Prosenc MH, Wolff M, Hort N, Willumeit R, Feyerabend F. Interference of magnesium corrosion with tetrazolium-based cytotoxicity assays. Acta Biomater. 2010;6(5):1813-23. [PubMed ID: 19837191]. https://doi.org/10.1016/j.actbio.2009.10.020.
-
25.
Ramage G, Vandewalle K, Wickes BL, Lopez-Ribot JL. Characteristics of biofilm formation by Candida albicans. Rev Iberoam Micol. 2001;18(4):163-70. [PubMed ID: 15496122].
-
26.
Coenye T, Nelis HJ. In vitro and in vivo model systems to study microbial biofilm formation. J Microbiol Methods. 2010;83(2):89-105. [PubMed ID: 20816706]. https://doi.org/10.1016/j.mimet.2010.08.018.