Abstract
Background:
Although regular exercise is beneficially acknowledged in promoting general health status, the cardiovascular risk of sudden cardiac death in highly training athletes has been reported. Unfortunately, the primary key factor in inducing cardiac problems in athletic populations is still questioned.Objectives:
In the present study, we aim to determine factors that could affect the relationship between physical activity level and cardiac autonomic function in the Thai population.Methods:
Forty participants were recruited and classified into three groups based on their physical activity level and sport profession, including sedentary men, elite handball players, and professional futsal players. In all participants, we measured predicted maximal oxygen consumption, time- and frequency- domain heart rate variability (HRV) variables at rest.Results:
Results demonstrated a positive correlation between time-domain HRV and estimated maximal oxygen consumption, but not with frequency-domain HRV, in which athletes aged lower than 25 years old (18 - 25 years old) had a significantly higher in the standard deviation of normal RR intervals (SDNN) and root mean square of the successive differences (RMSSD) than that in age-matched sedentary subjects. Interestingly, athletes aged above 25 years old (25 - 32 years old) had lower SDNN and RMSSD with higher low frequency/high-frequency ratio than those athletes aged under 25. Since all athletes aged above 25 years old were world-class national futsal players, stress due to the competitive level might be another factor influencing cardiac autonomic activity in athletes.Conclusions:
In conclusion, the study suggested the importance of age and stress exposure on cardiac autonomic regulation in elite athletes. The observed correlation also reinforced HRV as a potential marker in detecting abnormal changes in cardiac health even in an athletic population.Keywords
Heart Rate Variability Physical Activity Age Aerobic Capacity Cardiac Autonomic Regulation
1. Background
Exercise is an essential part of the daily life of people around the world. The physical and mental health benefits of exercise are well established. However, Intensive physical training is known to add significant load to the cardiovascular system, particularly in individuals affected by cardiovascular disease, and may eventually increase the risk for cardiac problems (1). It has been reported in the United States that sudden death in athletes under 35 years of age is predominantly associated with inherited cardiac conditions; for instance, hypertrophic cardiomyopathy (HCM) and anomalous origins of a coronary artery (1). Although cardiovascular disease risks are increased with age (2), recent evidence shows that young adults e.g. elite athletes are also prone to developing such cardiovascular problems (2, 3). A study from Landry et. al. summarized that sudden cardiac arrest during sport participation has accounted for 74 cases between 2009 - 2014 (4). The potential causes of sudden cardiac arrest are due to acute transient triggers, including acute myocardial ischemia, abrupt hemodynamic changes, and a significant increase in sympathetic stimulation which interact with arrhythmogenic substrates causing a ventricular fibrillation and eventually leads to cardiac arrest (5). Unfortunately, many of these diagnoses may not be clinically apparent and may first present with sudden death. Therefore, distinguishing between adaptive and maladaptive cardiovascular response to training is crucial to prevent the unnecessary termination of an athlete’s career and to minimize the risk of cardiac problems.
Multiple cardiac markers have been thoroughly investigated for the purpose of preventing the onset of SCA, including QT interval dispersion, baroreflex sensitivity as well as heart rate variability analysis (HRV) (6), a non-invasive method in evaluating changes in cardiac autonomic modulation. Not only has HRV been extensively used for clinical purposes assessing cardiac abnormalities, but the method has also been applied to monitor sport performance in athletes following a training adaptation as well (7). It has been reported that HRV was increased in athletes as compared to low physical activity persons, especially in endurance athletes (8, 9). Increased HRV particularly suggests the benefit of exercise and sport training on cardiac costs. In addition, monitoring HRV might helpfully specify the cardiac health in athletes.
At rest, heart rate variability could be affected by various physiological factors, in which the effects of age and stress on cardiac autonomic activity are clearly demonstrated (8, 10, 11). A decrease in HRV starts from middle ages throughout the entire life (11). While the stress during competition could decrease HRV toward more sympathetic activation (10), low HRV has shown to be independently predictive of increased mortality in post-myocardial infarction patients and CHF patients (3). Since exercise and sport training could increase HRV, it is therefore interesting that a proper training program might prevent the reduction due to aging and stress exposure.
Accordingly, in the present study, we aim to investigate the relationship between various physical activity levels and cardiac autonomic activity in elite Thai athletes in order to identify the potential adaptation of the heart. We hypothesized that high physical activity athletes have higher HRV than age-matched low physical activity persons. Persons with higher aerobic fitness might have higher HRV than those with lower aerobic fitness. Age groups between athletes might also affect the HRV quality. In addition, the effect of the competitive level on HRV has also been discussed.
2. Objectives
Intensive sport training programs can lead to either an advantage or maladaptation in cardiovascular performance depending on various factors of each individual athlete. The present study was conducted to evaluate the effect of physical activity level on cardiac autonomic modulation in male elite athletes. Resting heart rate variability was compared between national-youth handball players, world-class national futsal players, and low-activity university students.
3. Methods
3.1. Codes of Ethics
This cross-sectional research was approved by Mahidol University Central Institutional Review Board (MU-CIRB), which is in full compliance with International Guidelines for Human Research Protection such as the Declaration of Helsinki under the COA No. MU-CIRB 2019/173.2910.
3.2. Study Population
Participants were included from three different groups based on their physical activity levels and sport professionals. Low physical activity participants were randomly included from undergraduate and graduate student volunteers. Criteria for sedentary participants with low physical activity (S) were young males who had a physical activity lower than 150 minutes per week (600 METs-minute/week). Sport professional participants were included by purpose selection from the national youth handball team and national futsal team. All participants had reported no history of any cardiovascular, neurological. and metabolic diseases as well as a history of undergoing thoracic or abdominal operations. Participants also have not been under any medical prescription, which may influence the desired physiological system.
3.3. Study Tools
The general information, vital signs, and other study parameters have all been recorded on the study record form. Multiple tools were used to collect the data as followed.
International physical activity questionnaires (IPAQ)-short form Thai version translated by Rattanawiwatpong et al. (12) was used for evaluating the daily physical activity status of the participants based on metabolic equivalent (MET).
Heart rate variability measurement was performed by recording the electrocardiogram (ECG) of standard limb leads using the PowerLab data acquisition system (ADinstruments Ltd) and Labchart Pro analyzing software (ADinstruments Ltd). Both time and frequency domain variables were measured including: Standard deviation of all NN intervals (SDNN) and Root mean square of successive difference of RR intervals (RMSSD) whereas frequency domain includes low-frequency power (LF power), high-frequency power (HF power) and low frequency/high frequency (LF/HF ratio). Time-domain measurement was statistically calculated from the RR intervals and difference of RR intervals whereas frequency domain analysis was calculated with a non-parametric method (Fast Fourier Transform; FFT) provided by the Labchart Pro analyzing software to obtain power spectral analysis as suggested in the standard of measurement of heart rate variability (13).
Astrand-Rhyming test on a cycle ergometer (Monark ergomedic 874E) was performed to predict maximal oxygen consumption (VO2max) using Modified Astrand-Rhyming nomogram (14, 15). Heart rate was monitored during the test by ECG. The intensity level was increased every second if the target heart rate zone, between 120 and 170 bpm, was not achieved. The fifth and sixth minutes of exercise were averaged and used in the nomogram for VO2max prediction.
Bioelectrical impedance analysis (TANITA, MC-780U) was used to measure fat free mass, fat mass, bone density and other relevant body composition parameters via transmitting electrical currents through the body.
3.4. Study Protocol
All participants were contacted and given the IPAQ short form (Thai version) to determine the physical activity level. Relevant information e.g. objective, study procedure, and study equipment was then explained before receiving consent from the participants. Participants were told to follow the guideline and prepare themselves for the appointed date. On the appointed date, body weight, height, fat mass, muscle mass, and total body water were first measured. After 5 minutes rest, baseline blood pressure and heart rate at the left arm were tested in the supine position. In order to avoid bias, the participants were identified by sequential numbering. Individual profiles and IPAQ scores were blinded from investigators during ECG and aerobic capacity measurement. The ECG electrodes were placed at T2 and T5 level, mid-clavicular line of right and left sides of chest respectively; whereas the ground electrode was placed at T2 level, mid-clavicular line of the left side of the chest. Lead II ECG was recorded for another five minutes to determine resting HRV. After ECG measurement, participants were asked to test aerobic capacity with Astrand-Rhyming cycle ergometer protocol following the ACSM’s Guideline for exercise testing and prescription (16). The HRV parameters were analyzed after the aerobic capacity test to elude predisposition.
3.5. Inclusion and Exclusion Criterion
Subjects with a body mass index (BMI) ranging between 18.5 to 24.9 with no history of cardiovascular, kidney, neurological, and metabolic diseases were recruited. Any volunteers with the history of abdominal and thoracic operations, or any medications related to cardiovascular, neurological and muscle functions were excluded. In the sedentary group, participants with low physical activity or insufficiently active profile (less than 600 total METs-minute/week) were included. Athletes were considered as physically active (more than 3000 total METs-minute/week). Data from participants who had reported any signs of abnormality involving the cardiorespiratory, muscular or neurologic systems during measurement, or who were unable to comply with the study protocol were excluded from the analysis.
3.6. Statistical Analysis
The results were represented as mean ± standard deviation (SD). The population distribution was tested by D’Agostino and Pearson omnibus normality test to determine the statistical parametric. All samples were assumed for unequal variances. Brown-Forsythe and Welch ANOVA tests were used to analyze the variables among the three groups. Multiple comparisons were statistically calculated using Holm-Sidak’s multiple comparisons test. Independent t-test with Welch’s correction for normal distribution and Mann-Whitney U test for non-normal distribution data were used to analyze the variables between the two groups. Notably, it has been proposed that overall cardiovascular health has its peak around the age of 25 years before it gradually declines with advancing age (16). For the statistical analysis, the statistical software SPSS version 18.0 for Windows was used. The P values of 0.05 or less were considered as statistically significant.
4. Results
All participants were able to follow the study protocol completely. However, the data of one participant from the low physical activity group was excluded from analysis due to the uninterpretable results. General characteristics of all studied groups are reported in Table 1. All athletes showed a significantly higher degree of estimated VO2max than low physical activity persons. Maximum aerobic capacity was not different between athletes aging below 25 and above 25 years old. Body weight and BMI in both the age groups of athletes were higher than the low activity group. In regards to body composition, muscle mass was significantly higher in athletes when compared with the low activity population. No significant difference in percent body fat among experimental subjects was observed. Additionally, the IPAQ score demonstrated similar daily training activity between the two athlete groups.
General Characteristics and Physical Activity Level of All Studied Groups
Sedentary, Mean ± SD | Athlete Under 25, Mean ± SD | Athlete Over 25, Mean ± SD | P Value | DF | |
---|---|---|---|---|---|
N | 12 | 18 | 10 | ||
Age (year) | 22.4 ± 1.9 | 21.4 ± 1.8 | 28.4 ± 1.6a, b | < 0.0001 | 32.8 |
IPAQ score | 371 ± 220 | 5200 ± 2823a | 5704 ± 1489a | < 0.0001 | 25.7 |
Weight (kg) | 60.2 ± 7.4 | 70.4 ± 9.9a | 68.8 ± 8.9 | 0.0102 | 32.9 |
Height (cm) | 171 ± 5 | 173 ± 7 | 171 ± 4 | 0.4649 | 36.4 |
BMI | 20.5 ± 1.9 | 23.4 ± 2.5a | 23.4 ± 2.5a | 0.0055 | 30.4 |
Waist circumference (inches) | 30.8 ± 2.0 | 31.2 ± 2.4 | 31.4 ± 3.0 | 0.8657 | 25.1 |
Abdominal fat thickness (mm) | |||||
Right | 17.4 ± 6.0 | 12.8 ± 5.5 | 12.7 ± 11.1 | 0.2869 | 17.6 |
Left | 17.0 ± 6.4 | 13.0 ± 5.3 | 12.9 ± 11.3 | 0.3941 | 17.7 |
Fat mass | |||||
% | 17.4 ± 5.0 | 14.5 ± 6.9 | 15.9 ± 4.7 | 0.3627 | 36.9 |
kg | 10.72 ± 4.22 | 9.54 ± 4.74 | 11.18 ± 4.37 | 0.6032 | 33.4 |
Abdominal fat (%) | 18.5 ± 6.5 | 13.4 ± 6.0 | 16.5 ± 5.7 | 0.0856 | 32.3 |
Muscle mass | |||||
% | 78.3 ± 4.8 | 83.6 ± 5.4a | 84.0 ± 4.7a | 0.0095 | 34.4 |
kg | 46.9 ± 4.3 | 58.6 ± 6.3a | 57.6 ± 6.4a | < 0.0001 | 28.9 |
Abdominal muscle (kg) | 24.2 ± 2.2 | 27.8 ± 3.8a | 24.9 ± 2.7 | 0.0037 | 35.2 |
Total body water (%) | 54.1 ± 5.2 | 58.5 ± 8.7 | 58.9 ± 7.1 | 0.1982 | 33.0 |
Estimated VO2max (mL/kg/min) | 44.0 ± 5.6 | 57.8 ± 9.9a | 48.4 ± 18.4a | 0.0002 | 31.3 |
The effect of physical activity on cardiac autonomic activity was evaluated by measuring heart rate and heart rate variability indices of time- and frequency-domain analysis (Table 2). As compared by age matching (Figure 1), athletes aged below 25 years old showed significantly higher average RR interval, RMSSD, and SDNN than low physical activity persons (sedentary), without any significant difference in the frequency domain (LF & HF). Interestingly, SDNN and RMSSD of athletes aged above 25 years were significantly lower than that of athletes aged below 25 and became similar to low activity persons. Additionally, the resting LF/HF ratio in athletes aged over 25 years was increased when compared to both sedentary and athletes aged below 25 years.
Resting Heart Rate, Blood Pressure and Heart Rate Variability Indices of Time- and Frequency-Domain Analysis of all Studied Groups
Sedentary, Mean ± SD | Athlete Under 25, Mean ± SD | Athlete Over 25, Mean ± SD | P Value | DF | |
---|---|---|---|---|---|
Resting SBP (mmHg) | 123 ± 6 | 122 ± 7 | 124 ± 5 | 0.7293 | 36.4 |
Resting DBP (mmHg) | 71.6 ± 5.6 | 66.2 ± 6.4 | 68.6 ± 4.3 | 0.0329 | 35.9 |
Average RR (ms) | 881 ± 103 | 1017 ± 118a | 951 ± 157 | 0.0314 | 24.2 |
SDNN (ms) | 51.2 ± 13.5 | 90.5 ± 24.6a | 58.7 ± 21.5b | < 0.0001 | 30.0 |
Average rate (bpm) | 69.2 ± 7.5 | 60.2 ± 6.4a | 65.1 ± 11.8 | 0.0478 | 20.0 |
RMSSD (ms) | 40.0 ± 12.9 | 80.2 ± 26.8a | 38.13 ± 19.1b | < 0.0001 | 32.9 |
Total frequency (ms2) | 2524 ± 1402 | 8171 ± 4131a | 3787 ± 3319b | 0.0001 | 27.5 |
LF (ms2) | 742 ± 500 | 1855 ± 1136a | 1130 ± 1112 | 0.0137 | 25.6 |
HF (ms2) | 745 ± 513 | 3050 ± 2317a | 727 ± 760b | < 0.0001 | 23.9 |
LF (%) | 29.8 ± 11.4 | 23.0 ± 11.2 | 25.6 ± 11.7 | 0.3023 | 31.2 |
HF (%) | 34.5 ± 18.9 | 31.6 ± 11.5 | 17.2 ± 8.1ab | 0.0155 | 22.2 |
LF/HF (%) | 0.86 ± 0.78 | 0.82 ± 0.54 | 1.41 ± 0.63b | 0.1041 | 23.0 |
LFnu | 48.7 ± 22.9 | 39.9 ± 15.5 | 57.1 ± 13.7b | 0.0632 | 26.5 |
HFnu | 51.1 ± 21.5 | 57.2 ± 14.0 | 40.8 ± 11.6b | 0.0551 | 25.1 |
Time- and frequency-domain heart rate variability of sedentary (n = 12), athlete under 25 (n = 18) and over 25 years old (n = 10). (A) The standard deviation of NN intervals (SDNN), (B) root mean square of the successive differences (RMSSD) and (C) low frequency/high frequency (LF/HF ratio). *Significantly different (P < 0.05) between two groups using Brown-Forsythe and Welch ANOVA test.
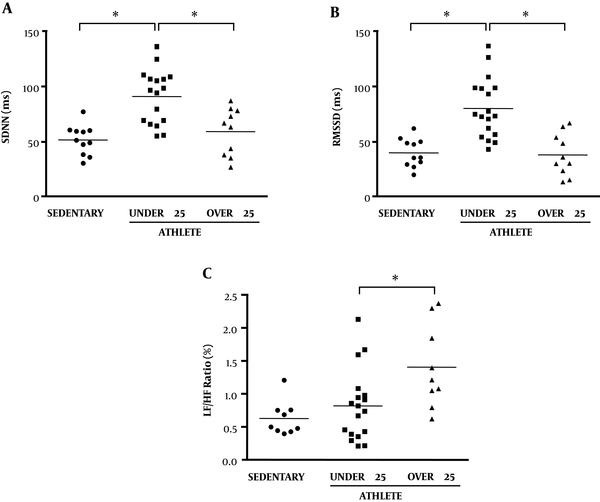
By comparing two groups of athletes, results demonstrated that handball players had a significantly higher RMSSD than futsal players (Figure 2B). While SDNN showed no difference between two athlete groups (Figure 2A), LF/HF was significantly increased in futsal players (Figure 2C). Data suggested that specific sports or stress due to competitive levels potentially affect time- and frequency-domain of HRV. In order to clarify the major factors that influence HRV quality in athletes, the HRV parameters of futsal players aged under 25 years (n = 5) were compared with that of futsal players aged above 25 years (n = 10). Results showed that RMSSD of futsal players aged above 25 years became lower than the RMSSD of futsal players aged under 25 years (Figure 3B). However, there was no significant difference in SDNN and LF/HF between these two age groups of futsal teams (Figure 3A and C).
Time- and frequency-domain heart rate variability of handball players (n = 13) and futsal players (n = 15). (A) The standard deviation of NN intervals (SDNN), (B) root mean square of the successive differences (RMSSD) and (C) low frequency/high frequency (LF/HF ratio). *Significantly different (P < 0.05) between two groups using the Student unpaired t-test.
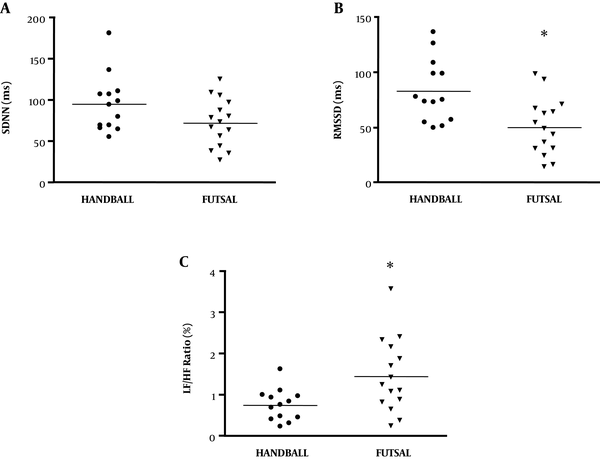
Time- and frequency-domain heart rate variability of futsal players of age between under 25 (n = 5) and over 25 years old (n = 10). (A) The standard deviation of NN intervals (SDNN), (B) root mean square of the successive differences (RMSSD) and (C) low frequency/high frequency (LF/HF ratio). *Significantly different (P < 0.05) between two groups using the Student unpaired t-test.
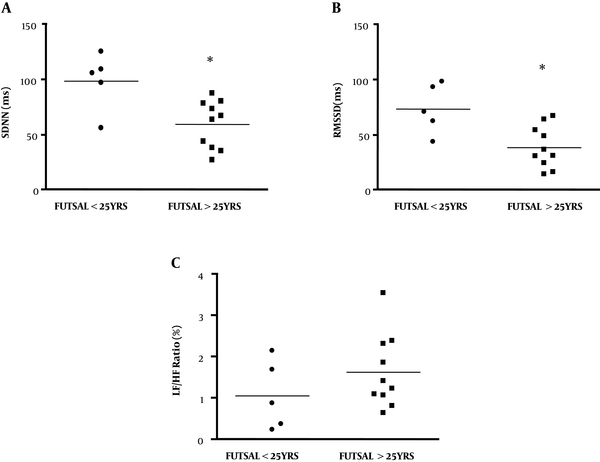
Furthermore, the relationship between cardiorespiratory fitness and heart rate variability at rest was determined from all participants together (Figure 4). The relationships between estimated oxygen consumption level and time-domain HRV, either SDNN or RMSSD, showed a moderate positive correlation (r = 0.53 and 0.43, respectively). On the other hand, the relationship between the fitness level and LF/HF was very low (r = 0.046). When the data of age over 25 years old were excluded, the correlations between the aerobic fitness and either SDNN or RMSSD were proportionally decreased (r = 0.41 and 0.26, respectively). These results implied that age has no significant impact on cardiac autonomic activation even in well-trained athletes.
Correlation analysis between the estimated maximal oxygen consumption and SDNN (A, D), RMSSD (B, E) and LF/HF ratio (C, F). A, B, and C are summarized from all subjects of all three groups, while D, E, and F are included all subjects aged under 25 years old. The central line represents medians values and the graphs show the best fitted lines with 95% confidence interval. Statistical analyses were performed by Pearson’s test. Each point represents one subject. r2: coefficient of determination.
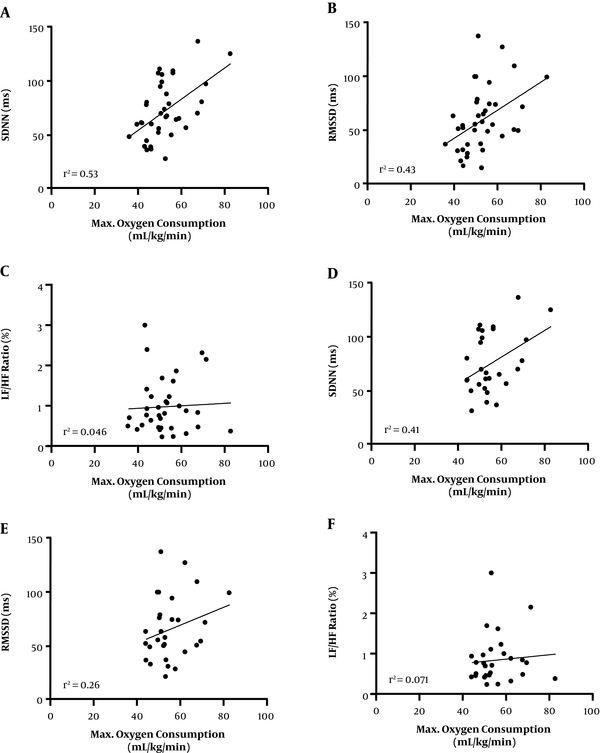
5. Discussion
The present study aimed to verify the factors that could affect cardiac autonomic activation in team sport athletes. The level of physical activity, age difference, and competitive level of athletes affecting heart rate variability were demonstrated. The results show that athletes under 25 years had significantly higher time-domain HRV parameters than those in age-matched low physical activity persons. Interestingly, athletes aged above 25 years had significantly less HRV profiles than that of athletes under 25 years. A significantly higher time-domain HRV in elite handball players compared to world-class futsal players were observed despite relatively similar daily physical activity training. This finding suggested that the competitive level partly influences cardiac autonomic activation. Modest positive correlation between physical fitness and time-domain HRV supports an indication that exercise training helps increase parasympathetic over sympathetic activation at rest. Indeed, this beneficial effect of exercise training is modified by age and stress conditions.
HRV is a non-invasive measurement tool that has been widely used in clinical settings and sport-related fields. Each HRV variable elicits a distinct function, for example, high SDNN and RMSSD, and time-domain measurement, indicate higher parasympathetic regulation (17, 18) representing less cardiac work. In regards to the frequency-domain, high frequency reflects parasympathetic activity, whereas low frequency is shown to be associated with sympathetic activity (17). It has been mostly believed that regular exercise training helps increasing parasympathetic modulation (or lower sympathetic activity) at rest (19).
Although regular exercise has been recommended for improving cardiovascular performance, many individual factors need to be considered. In the present study, we primarily hypothesized that intensive exercise and sports training may not deliver a healthy benefit to the heart. Results support this idea by which age and/or competitive stress become important factors. Similar to our findings, Melo et al. (20) studied the HRV in the active, non-athlete population. They demonstrated a significantly higher time-domain HRV e.g. RMSSD in active young men compared to those in age-matched sedentary men. Likewise, they also reported that active elderly people had a higher LF/HF ratio (low HRV) than active young men. Correspondingly, our results added more knowledge that it is not only the elderly effect. Age-dependent alteration in HRV is also observed in elite athletes from young adults to the middle ages. Besides age dependence, mode of exercise training might also influence cardiac autonomic adaptation. It has been demonstrated that cardiac autonomic nervous response was dependant on the amount of volume-load in marathon runners (21). On the other hand, the response to resistance training was substantially contradicted to the response to aerobic training (22). Therefore, excessive volume-load of aerobic training induced a higher sympathetic activation, while high training load in resistance training activated a progressive parasympathetic predominance. Unfortunately, both studies were performed within the same age range. It is therefore interesting to see whether age difference could affect cardiac autonomic adaptation in different modes of exercise. Nevertheless, since handball and futsal players are trained for aerobic and resistance together in a program, the response should not be similar to a marathon runner or weightlifter.
Most athletes were typically exposed to physiological and psychological stress especially during pre-competitive and competitive phases (23). The great amount of stress could remarkably affect athletes’ performance as well as the cardiac health status. Studies have reported a high LF/HF ratio when faced with stressful situations such as the pre-training period describing the greater sympathetic modulation (10). From our results, handball players had a lower HRV than futsal players, whereas a higher LF/HF ratio was found in futsal players. At the time of study, the Thailand national futsal team was in the top-twenty world rankings. An elevated psychological stress was then expected. Interestingly, when deeply focusing on futsal players, the athletes with an age under 25 years demonstrated higher SDNN and RMSSD than that of athletes above 25 years. However, it is well-accepted that younger athletes mostly have higher levels of competitive anxiety than older athletes (24). Thus, it could not conclude that competitive stress is a main factor in decreasing HRV in futsal athletes. Besides psychological stress, physiological stress of overtraining and injury might affect HRV adaptation. Mourot et. al. have demonstrated the decreased HRV in athletes suffering from overtraining syndrome when compared with normally trained participants (25). Injury and overreaching in older athletes might then be another mechanism of high sympathetic activity. This finding raises the concern towards sufficient training programs and well-managed emotional status among athletes in order to avoid overtraining syndrome. Unfortunately, we did not evaluate the psychological stress and overreaching state of participants in the present study. We assumed that the stress level of all futsal players in the team should not be much different.
Besides the cardiac autonomic activation, heart rate variability might be a predictor of aerobic capability. Many previous studies have proposed the correlation between HRV and cardiorespiratory fitness (26, 27). Phoemsapthawee et. al. showed the positive correlation of HRV and peak oxygen consumption in sedentary and exercise-trained young obese participants (26). Similarly, Leite et. al. also concluded that HRV is a predictive measurement tool in estimating peak oxygen uptake in COPD patients (27). Our present finding in athletes also supports the positive correlation between time-domain HRV and maximal oxygen consumption. From this evidence, it is undoubted that HRV could be a reliable predictive tool for monitoring the aerobic fitness profile in athletes. Numerous studies have also reported the effectiveness of HRV in monitoring training adaptation, maladaptation as well as the biofeedback during training sessions (7, 28). The primary aim is to determine optimal training load, which subsequently leads to performance improvement. Moreover, reduction in HRV during the sport training program could be the indicator of overreaching and overtraining development.
Notably, the study had a limitation in which no interventions and invasive procedures were allowed in the athletes. Therefore, stress conditions were not possible to evaluate in this study. In addition, the present study was performed only in the male population at the age of peak performance; whether results in female and/or aged athletes demonstrate similar responses are also of interest. Accordingly, future studies regarding the gender difference and aging effects on HRV response should be further investigated.
5.1. Conclusion
From the results, it can be concluded that physical activity and age following training are important factors influencing heart rate variability and cardiac autonomic regulation. The involvement of competitive stress in cardiac autonomic regulation was also proposed. Although an exercise training program is beneficial, a sufficient exercise program should be cautiously executed in order to avoid maladaptive cardiac functions which could consequently result in athletes’ career termination or cardiac problems. In addition, as the correlation between HRV and maximal oxygen consumption is demonstrated, HRV may be a potential marker in detecting abnormal changes of cardiac health in the general population and professional athletes.
Acknowledgements
References
-
1.
Wasfy MM, Hutter AM, Weiner RB. Sudden Cardiac Death in Athletes. Methodist Debakey Cardiovasc J. 2016;12(2):76-80. [PubMed ID: 27486488]. [PubMed Central ID: PMC4969030]. https://doi.org/10.14797/mdcj-12-2-76.
-
2.
Eckart RE, Shry EA, Burke AP, McNear JA, Appel DA, Castillo-Rojas LM, et al. Sudden death in young adults: an autopsy-based series of a population undergoing active surveillance. J Am Coll Cardiol. 2011;58(12):1254-61. [PubMed ID: 21903060]. https://doi.org/10.1016/j.jacc.2011.01.049.
-
3.
Sessa F, Anna V, Messina G, Cibelli G, Monda V, Marsala G, et al. Heart rate variability as predictive factor for sudden cardiac death. Aging. 2018;10(2):166-77. [PubMed ID: 29476045]. https://doi.org/10.18632/aging.101386.
-
4.
Landry CH, Allan KS, Connelly KA, Cunningham K, Morrison LJ, Dorian P, et al. Sudden Cardiac Arrest during Participation in Competitive Sports. N Engl J Med. 2017;377(20):1943-53. [PubMed ID: 29141175]. [PubMed Central ID: PMC5726886]. https://doi.org/10.1056/NEJMoa1615710.
-
5.
Corrado D, Migliore F, Basso C, Thiene G. Exercise and the risk of sudden cardiac death. Herz. 2006;31(6):553-8. [PubMed ID: 17036186]. https://doi.org/10.1007/s00059-006-2885-8.
-
6.
Gimeno-Blanes FJ, Blanco-Velasco M, Barquero-Perez O, Garcia-Alberola A, Rojo-Alvarez JL. Sudden Cardiac Risk Stratification with Electrocardiographic Indices - A Review on Computational Processing, Technology Transfer, and Scientific Evidence. Front Physiol. 2016;7:82. [PubMed ID: 27014083]. [PubMed Central ID: PMC4780431]. https://doi.org/10.3389/fphys.2016.00082.
-
7.
Dong JG. The role of heart rate variability in sports physiology. Exp Ther Med. 2016;11(5):1531-6. [PubMed ID: 27168768]. [PubMed Central ID: PMC4840584]. https://doi.org/10.3892/etm.2016.3104.
-
8.
Deus LA, Sousa CV, Rosa TS, Filho JMS, Santos PA, Barbosa LD, et al. Heart rate variability in middle-aged sprint and endurance athletes. Physiol Behav. 2019;205:39-43. [PubMed ID: 30389479]. https://doi.org/10.1016/j.physbeh.2018.10.018.
-
9.
Kiss O, Sydo N, Vargha P, Vago H, Czimbalmos C, Edes E, et al. Detailed heart rate variability analysis in athletes. Clin Auton Res. 2016;26(4):245-52. [PubMed ID: 27271053]. https://doi.org/10.1007/s10286-016-0360-z.
-
10.
Souza RA, Beltran OAB, Zapata DM, Silva E, Freitas WZ, Junior RV, et al. Heart rate variability, salivary cortisol and competitive state anxiety responses during pre-competition and pre-training moments. Biol Sport. 2019;36(1):39-46. [PubMed ID: 30899138]. [PubMed Central ID: PMC6413577]. https://doi.org/10.5114/biolsport.2018.78905.
-
11.
Jandackova VK, Scholes S, Britton A, Steptoe A. Are Changes in Heart Rate Variability in Middle-Aged and Older People Normative or Caused by Pathological Conditions? Findings From a Large Population-Based Longitudinal Cohort Study. J Am Heart Assoc. 2016;5(2). [PubMed ID: 26873682]. [PubMed Central ID: PMC4802439]. https://doi.org/10.1161/JAHA.115.002365.
-
12.
Rattanawiwatpong P, Khunphasee A, Pongurgsorn C, Intarakamhang P. Validity and reliability of the Thai version of Short Format International Physical Activity Questionnaire (IPAQ). J Thai Rehabil. 2006;16(3):147-60.
-
13.
European Society of Cardiology and The North American Society of Pacing and Electrophysiology. Heart rate variability--Standards of measurement, physiological interpretation, and clinical use. European Heart Journal. 1996;17:354-81.
-
14.
Ferguson B. ACSM’s Guidelines for Exercise Testing and Prescription 9th Ed. 2014. The Journal of the Canadian Chiropractic Association. 2014;58(3):328. [PubMed ID: PMC4139760].
-
15.
Astrand PO, Ryhming I. A nomogram for calculation of aerobic capacity (physical fitness) from pulse rate during sub-maximal work. J Appl Physiol. 1954;7(2):218-21. [PubMed ID: 13211501]. https://doi.org/10.1152/jappl.1954.7.2.218.
-
16.
Heyward V, Gibson A. Advanced fitness assessment and exercise prescription. 7th ed. Champaign, IL: Human Kinetics; 2014. p. 99-108.
-
17.
Malik M. Heart rate variability: Standards of measurement, physiological interpretation, and clinical use. 93. Circulation; 1996.
-
18.
Shaffer F, Ginsberg JP. An Overview of Heart Rate Variability Metrics and Norms. Front Public Health. 2017;5:258. [PubMed ID: 29034226]. [PubMed Central ID: PMC5624990]. https://doi.org/10.3389/fpubh.2017.00258.
-
19.
Berkoff DJ, Cairns CB, Sanchez LD, Moorman C3. Heart rate variability in elite American track-and-field athletes. J Strength Cond Res. 2007;21(1):227-31. [PubMed ID: 17313294]. https://doi.org/10.1519/00124278-200702000-00041.
-
20.
Melo RC, Santos MD, Silva E, Quiterio RJ, Moreno MA, Reis MS, et al. Effects of age and physical activity on the autonomic control of heart rate in healthy men. Braz J Med Biol Res. 2005;38(9):1331-8. [PubMed ID: 16138216].
-
21.
Manzi V, Castagna C, Padua E, Lombardo M, D'Ottavio S, Massaro M, et al. Dose-response relationship of autonomic nervous system responses to individualized training impulse in marathon runners. Am J Physiol Heart Circ Physiol. 2009;296(6):H1733-40. [PubMed ID: 19329770]. https://doi.org/10.1152/ajpheart.00054.2009.
-
22.
Iellamo F, Lucini D, Volterrani M, Casasco M, Salvati A, Gianfelici A, et al. Autonomic nervous system responses to strength training in top-level weight lifters. Physiol Rep. 2019;7(20). e14233. [PubMed ID: 31642195]. [PubMed Central ID: PMC6805848]. https://doi.org/10.14814/phy2.14233.
-
23.
van Paridon KN, Timmis MA, Nevison CM, Bristow M. The anticipatory stress response to sport competition; a systematic review with meta-analysis of cortisol reactivity. BMJ Open Sport Exerc Med. 2017;3(1). e000261. [PubMed ID: 29177073]. [PubMed Central ID: PMC5604718]. https://doi.org/10.1136/bmjsem-2017-000261.
-
24.
Rice SM, Gwyther K, Santesteban-Echarri O, Baron D, Gorczynski P, Gouttebarge V, et al. Determinants of anxiety in elite athletes: a systematic review and meta-analysis. Br J Sports Med. 2019;53(11):722-30. [PubMed ID: 31097452]. [PubMed Central ID: PMC6579501]. https://doi.org/10.1136/bjsports-2019-100620.
-
25.
Mourot L, Bouhaddi M, Perrey S, Cappelle S, Henriet M, Wolf J, et al. Decrease in heart rate variability with overtraining: assessment by the Poincaré plot analysis. Clinical Physiology and Functional Imaging. 2004;24(1):10-8. https://doi.org/10.1046/j.1475-0961.2003.00523.x.
-
26.
Phoemsapthawee J, Prasertsri P, Leelayuwat N. Heart rate variability responses to a combined exercise training program: correlation with adiposity and cardiorespiratory fitness changes in obese young men. J Exerc Rehabil. 2019;15(1):114-22. [PubMed ID: 30899746]. [PubMed Central ID: PMC6416511]. https://doi.org/10.12965/jer.1836486.243.
-
27.
Leite MR, Ramos EM, Kalva-Filho CA, Rodrigues FM, Freire AP, Tacao GY, et al. Correlation between heart rate variability indexes and aerobic physiological variables in patients with COPD. Respirology. 2015;20(2):273-8. [PubMed ID: 25381699]. https://doi.org/10.1111/resp.12424.
-
28.
Jimenez Morgan S, Molina Mora JA. Effect of Heart Rate Variability Biofeedback on Sport Performance, a Systematic Review. Appl Psychophysiol Biofeedback. 2017;42(3):235-45. [PubMed ID: 28573597]. https://doi.org/10.1007/s10484-017-9364-2.