Abstract
Background:
Various training protocols have been used in combat sports, including Taekwondo (TKD), to rapidly improve performance and to avoid injury by balancing the exercise intensity and the athletes’ metabolic tolerance and hormonal response. However, little is known about such metabolic responses in previously-trained individuals.Objectives:
To investigate the effects of a concurrent TKD plus resistance (RT) training program on post-exercise blood biomarkers of physiological stress in previously-trained individuals.Methods:
Twenty-eight (16 males: 12 females) previously-trained individuals aged 20 - 26 years were matched by gender and randomly divided into either moderate- to vigorous-intensity exercising group (MODG) or a high-intensity exercising group (HIG). The MODG performed TKD training at a target training intensity of 55 - 70% (weeks 1 - 2) and 70 - 85% (weeks 3 - 4) maximum heart rate (HRmax) and RT training for four sets of 10 - 15 repetitions for each of the 13 whole-body RT exercises. The HIG performed TKD training at a target training intensity of 85 - 100% (weeks 1 - 2) and 95 - 100% (weeks 3 - 4) HRmax and RT training for five sets of 10 - 15 repetitions for each of the 13 whole-body RT exercises. A structured taekwondo tasks (sTT) test was conducted before and after the training period.Results:
Significant post-exercise improvements were found for total testosterone (P = 0.02), free testosterone (P = 0.01), C/T ratio (P = 0.03) and lactate (P = 0.03) in the mixed gender HIG. With regards to the specific gender effects, post-exercise improvements were found in S-CPK in the male MODG and HIG, with improvements in uric acid only in the male HIG. In turn, post-exercise improvements were found for total testosterone, testosterone binding globulin, free testosterone in the female MODG and HIG, with post-exercise improvements in S-CPK and uric acid in the female HIG only.Conclusions:
This study demonstrates that even a short period of concurrent taekwondo and resistance training improves adaptation and post-exercise responses to physiological stress, and such adaptation may even be more marked in previously-trained female athletes. Thus, this mode of training may provide a novel exercise training modality to rapidly improve an individuals’ capacity to exercise, especially in situations of plateau, while avoiding overtraining and an increased concomitant chance of illness and musculoskeletal injury.Keywords
Adaptation to Exercise Combat Sport Cortisol Muscle Damage Testosterone
1. Background
Participation in sporting activities induces both acute and chronic metabolic changes through physical training and competition (1). While overload training aims to cause an imbalance between training load and recovery, this overload is only for a short time and for a load that is manageable, resulting in a return to homeostasis. Should the load be too stressful or too easy, a state of overtraining can be observed with various typical alterations (e.g., molecular, biochemical and regulatory), which may lead to disturbances of underperformance, well-being, and possible illness and injury (2). Thankfully, biochemical and hematological data have allowed the identification of the balance between training and recovery (2, 3).
An incorrect stimulation and overproduction of the catabolic hormones and/or decrease in anabolic hormones (i.e., testosterone) may result in an increased risk of musculoskeletal injuries (1-4). Specifically, cortisol is a powerful hormone controlled by the adrenal cortex and has a catabolic effect. Thus, it is essential for an athlete to reduce levels of cortisol to achieve tissue growth, bone health, ligament health, and positive adaptations to exercise training, while avoiding excessive tissue inflammation and injury (2, 3). Problematically, excess cortisol also suppresses the immune system, producing a greater risk of infections and injury and concomitant decreased levels of testosterone (3, 5). In turn, exercise that increases anabolic hormone production may result in an increased tensile strength of contractile and non-contractile units, effectively reducing the risk of musculoskeletal injury (1-5). Testosterone also plays a key role in health and well-being and is responsible for increased muscle and bone mass, with insufficient levels leading to abnormalities, including frailty and bone loss that could result in musculoskeletal injuries (6).
More recently, elevated uric acid and serum creatine phosphokinase (S-CPK) levels have been demonstrated to be a greater risk factor for musculoskeletal damage (7). Also, athletes undergoing changes in response to endurance training may produce more free radicals, resulting in strong physical exertion-induced oxidative stress (8, 9). This may, in turn, affect signaling pathways in the muscles, increasing the possibility of musculoskeletal injury (8). While research on endurance training is forthcoming, such research on the association of various sports and even resistance training and biomarkers of oxidative stress are scarce (8, 9). Further, exercise of too high an intensity may affect muscle signaling and metabolism (8, 9) and the role of some endogenous antioxidants, such as uric acid and lactate, in scavenging free radicals (8, 9). Specifically, uric acid oxidation scavenges oxo-heme oxidants, lipid hydroperoxide radicals, hydroxyl radicals (10), and singlet molecular oxygen (11). In addition to inhibiting lipid peroxidation, urate-ferric ion (Fe3+) complexes also intensely inhibit Fe3+-catalyzed ascorbate oxidation (12). Physiological urate further increases the stability of ascorbate in human serum approximately and may protect against H+-induced ascorbate oxidation (13). Similarly, the lactate ion might be considered as a potential antioxidant agent, with scavenging activities of lactate toward both the superoxide anion (O2-) and hydroxyl radical (OH), and an ability to inhibit lipid peroxidation (14).
Specifically, resistance training, dependent on the type of resistance training, intensity, number of sets, and type of muscle contraction, have been demonstrated to stimulate greater increase in testosterone levels when compared to aerobic training (7, 9, 15). It is important to note that many sports contain both aerobic and resistance training elements and could potentially stimulate both anabolic and catabolic hormonal responses or adaptations (16). TKD is one such intermittent sport in which the athletes have to train and compete at a variety of intensities, utilizing all of the energy systems (17). This is because the actions involved in TKD are characterized by periods of high levels of technical, tactical, psychological, physical fitness, and physiological characteristics (18, 19).
It is therefore critical to determine the effect of various exercise modalities, either alone or in combination, on blood biomarkers of muscle damage (1-5). However, few studies are forthcoming on individual martial arts disciplines, especially taekwondo (TKD), and how they affect acute blood biomarkers of muscle damage (17, 20). This would provide support for guidance to those athletes, coaches, as well as conditioning and health specialists to help them understand the preferred strategies to prevent musculoskeletal injury risk specific to their sport.
2. Objectives
To investigate the effects of a concurrent TKD plus resistance (RT) training program on post-exercise blood biomarkers of physiological stress in previously-trained individuals.
3. Methods
3.1. Participants
A pretest-posttest randomized-groups experimental study took place over four weeks (Figure 1). Purposive sampling was utilized to recruit participants who volunteered to participate in this study. No estimation of a proportion was made since this study is a small scale proof-of-concept investigation. Participants were TKD athletes with the South African Taekwondo Federation and had more than two years of training experience (training age mean: 7.4 ± 1.6 years), were recruited in the Zululand area, Kwazulu-Natal, South-Africa. Following sampling, in order to eliminate the physiological and hormonal influences accompanying gender which may have compromised the findings of the study, 28 previously-trained individuals (16 males and 12 females; mean age: 23.5 ± 2.6 years; mean height: 1.73 ± 0.40 m) participants were grouped by gender and randomly assigned using a random numbers table to either moderate- to vigorous-intensity exercising group (MODG, n = 8 males, n = 6 females; mean age 23.1 ± 2.5 years; mean height 1.72 ± 0.51 m) or a high-intensity exercising group (HIG, n = 8 male, n = 6 female; mean age 22.9 ± 2.73 years; mean height 1.73 ± 0.34 m).
CONSORT flow diagram of study
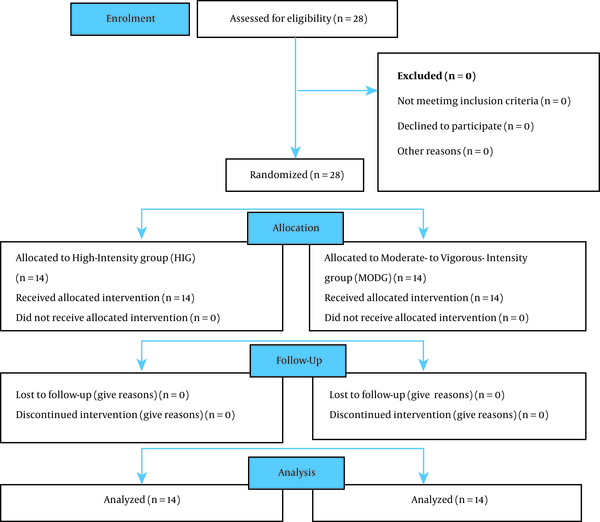
Written informed consent was obtained from the participants following an explanation of the purpose of the study, measurement procedures, and the possible adverse events that could be encountered during the study. Following written informed consent, all participants participated in a TKD test before and after the four-week concurrent TKD and RT program. Participants' blood samples were obtained pre- and post-experimentally in the morning between 07:30 and 09:00 by registered nurses from the antecubital vein following a 12-hour fast, and their testosterone, cortisol, S-CPK, uric acid, and lactate were analyzed at an International Organization for Standardization (ISO)-accredited laboratory. The study was conducted according to the Human Rights Declaration of Helsinki and approved by the South African Taekwondo Federation (SATF). Ethical clearance to conduct this study was obtained from the Institutional Review Boards of the University of Zululand (UZREC 171110030 PGD 2015/88).
3.2. Biochemical Analyses
Blood samples were obtained pre- and post-experimentally in the morning between 07:30 and 09:00 by registered nurses from the antecubital vein following a 12-hour fast and analyzed at an International Organization for Standardization (ISO)-accredited laboratory (Lancet Laboratories®, Bay Hospital, Richards Bay, South Africa). Testosterone, cortisol, creatine phosphokinase (S-CPK), uric acid, and lactate were analyzed using commercially available kits (Phoenix Peptides, Karlsruhe, Germany) according to the South African Standards of Good Laboratory Practice. Testosterone, cortisol, S-CPK, uric acid, and lactate concentrations were measured using Architect© Immunoassay kits (Abbott Ireland Diagnostics Division, Longford, Ireland). The functional sensitivity of the testosterone and cortisol assays was calculated to be 0.14 ng/mL and ≤ 1 μg/dL, respectively. The limits of detection for S-CPK is 5 U.L-1, 0.06 mg.dL-1 for uric acid and 0.05 mg.dL-1 (0.006 mmol.L-1) for lactic acid. The intra-assay coefficient of variation for testosterone, cortisol, S-CPK, uric acid, and lactate was 4.5, 5.5, ≤ 3.6, ≤ 6.0, and ≤ 6.3%, respectively. To control for variations in plasmatic volume, minimum sample volume is calculated automatically by the system.
3.3. Intervention
All participants participated in a TKD test before and after a four-week concurrent TKD and RT program that consisted of a five-minute warm-up that entailed jogging, shuttle runs, cone drills, and five minutes of dynamic whole-body stretching (21). This was then followed by a one-hour TKD workout, and each workout concluded with a five-minute cool-down entailing jogging and soft kicks and five-minutes of whole-body static stretching exercises (12). The structured TKD test is a technical test of 20 minutes (22). The first 5 minutes consisted of stepping back and forth in different positions on ready stance (defense, attack, and counter-attack) at approximately 75 - 85% HRmax. Since HRmax can be determined directly, through ergometers with incremental effort protocols, or indirectly, through predictive equations, the present study utilized a predictive equation. This is so since, in addition to attempting to make exercise testing affordable, maximum HR testing is more likely to cause adverse events and requires medical supervision and ECG monitoring. As such, HRmax was calculated using the estimation of HRmax formula: 220-age (23). The next 10 minutes consisted of two sets of 16 repetitions for 5 minutes each of kicking techniques at 65 - 95% HRmax executing different techniques, such as; dollyo chagi montong, naeryo chagi, parambal dollyo chagi, chirrugi, mirro chagi, nare chagi, dwit chagi. The final 5 minutes consisted of one set of technical exercises: continuous push- ups, sit- ups, burpees for 3 minutes, and one set of sparring drills using mini matches/sparring with an opponent from the same category all performed at an intensity of 100%HRmax (22).
While both groups participated in the five-day weekly four-week training program (22), the MODG participated in a moderate- to vigorous-intensity, one-hour TKD workout that involved both upper- and lower-body TKD techniques (blocks, punches, and kicks) at a target training intensity of 55 - 70% maximum heart rate (HRmax) for weeks 1 and 2 and 70 - 85% HRmax for weeks 3 and 4 (13). Target HR ranges were monitored continuously using a HR monitor (Polar Electro, Kempele, Finland) during each session. The RT portion of the MODG took place five times weekly for 30 - 45 min in the afternoon to limit the interference effect (23) and consisted of power cleans, push presses, dumbbell raises, power snatches, squats, deadlifts, leg curls, bench press, latissimus dorsi pulldowns, seated row, abdominal crosses, supine “bicycles” and spiderman plank crunches (24, 25). MODG RT exercises were conducted for four sets and 10 - 15 repetitions each (24), focusing on hypertrophy and muscular endurance (9). This standardized load, instead of load based on an individualized one-repetition-maximum (1-RM) testing, was selected since the inverse relationship that exists between repetitions and intensity allows for the use of a repetition maximum continuum whereby 10 repetitions would correspond to approximately 75% 1-RM and 15 repetitions to approximately 65% 1-RM in all participants (9). As the focus was on hypertrophy- and muscular endurance-type RT, inter- and intra-rest periods lasted 30 seconds (9).
In turn, the high-intensity TKD portion of the HIG four-week program also consisted of a one-hour TKD workout performed in the morning, but in contrast to the MODG program, intensity was set at 85 - 100% HRmax for weeks 3 - 4 (21). In contrast to the MODG, HIG RT exercises were conducted for five sets and 10 - 15 repetitions each (24).
3.4. Statistics Analysis
All statistical analysis was performed using the SPSS for Windows software (version 20.0, SPSS Inc., Chicago, Illinois, USA). T-tests were utilized to examine the differences in hormonal and biochemical responses to taekwondo training. The changes that arose after the tests before and after training were compared using a two-way (group × time) repeated-measures analysis of variance (ANOVA). This was performed as a whole for the HIG and MODG and also per gender to determine if any gender effects were present. Data are presented as means ± standard deviation (SD). For all measured variables in this study, the small sample size necessitated that the normality assumption could not be met and used of a more conservative P-value of 0.01 in addition to 0.05 (26). Further, absolute (i.e. value of the indicator in period 2 minus that in period 1) and relative changes (i.e. change in the indicator in percentage terms) were calculated and presented as mean differences and percentage (%) change, respectively.
4. Results
Prior to the four weeks of concurrent training, the measured blood biomarkers of muscle damage were found to be normal and within the acceptable clinical ranges for the conditions under which they were collected (27). The ANOVA demonstrated that a statistically-significant interaction was found for total testosterone (P = 0.02); free testosterone (P = 0.01), C/T ratio (P = 0.03) and lactate (P = 0.03). With regards to total testosterone, the HIG decreased their mean values by 4.2 nmol.L-1 (21.32%) prior to the training but then increased their testosterone value by a mean of 0.1 nmol.L-1 (0.52%). In turn, the MODG increased their mean values by 2.0 nmol.L-1 (7.72%) before training but then decreased their mean values by 1.7 nmol.L-1 (5.64%) after the training period. Free testosterone was decreased by a mean of 104.3 pmol.L-1 (27.51%) in the HIG before the four-week training period but then increased by 13.9 pmol.L-1 (4.13%) following the high-intensity training period. In turn, the MODG increased their mean values by 107.0 pmol.L-1 (23.53%) before training, but then (similarly to the HIG) increased their values by 37.3 pmol.L-1 (23.53%) after training. For the C/T ratio, the HIG were found to have increased their ratio before training by a mean of 1.9 (12.67%), but then decreased this ratio by a mean of 1.2 (6.97%) following training. The MODG, in turn, decreased their C/T ratio by a mean of 2.6 (23.63%) before their training regime, followed by a mean decrease of 1.0 (9.17%) following training. Lactate was found to be decreased by a mean of 0.2 mmol.L-1 (11.76%) before training but increased by a mean of 1.2 mmol.L-1 (25.0%) following their training program. Similarly, the MODG was found to have a decreased lactate (mean: 0.4 mmol.L-1; 33.33%) before training, with an increased lactate following their respective training (mean: 2.0 mmol.L-1; 40.81%).
With regards to total testosterone, the HIG decreased their mean values by 4.2 nmol.L-1 (21.32%) prior to the training but then increased their testosterone value by a mean of 0.1 nmol.L-1 (0.52%). In turn, the MODG increased their mean values by 2.0 nmol.L-1 (7.72%) before training but then decreased their mean values by 1.7 nmol.L-1 (5.64%) after the training period. Free testosterone was decreased by a mean of 104.3 pmol.L-1 (27.51%) in the HIG before the four-week training period, but then increased by 13.9 pmol.L-1 (4.13%) following the high-intensity training period. In turn, the MODG increased their mean values by 107.0 pmol.L-1 (23.53%) before training, but then (similarly to the HIG) increased their values by 37.3 pmol.L-1 (23.53%) after training. For the C/T ratio, the HIG were found to have increased their ratio before training by a mean of 1.9 (12.67%), but then decreased this ratio by a mean of 1.2 (6.97%) following training. The MODG, in turn, decreased their C/T ratio by a mean of 2.6 (23.63%) before their training regime, followed by a mean decrease of 1.0 (9.17%) following training. Lactate was found to be decreased by a mean of 0.2 mmol.L-1 (11.76%) before training, but increased by a mean of 1.2 mmol.L-1 (25.0%) following their training program. Similarly, the MODG were found to have a decreased lactate (mean: 0.4 mmol.L-1; 33.33%) before training, with an increased lactate following their respective training (mean: 2.0 mmol.L-1; 40.81%).
When specifically examining the effect of concurrent training per gender group to determine if any gender effect existed, the two-way ANOVA with repeated measures with Bonferroni post hoc test demonstrated that the male TKD athletes had a statistically-significant interaction for S-CPK (P = 0.001) and uric acid (P = 0.03). Specifically, the HIG were found to have decreased their S-CPK before training by a mean of 73.0 IU.L-1 (7.72%) and by 98.2 IU.L-1 (11.82%) following their four weeks of high-intensity training. In turn, the MODG decreased their S-CPK by a mean of 65.8.8 IU.L-1 (9.96%) before training and by a mean of 36.7 IU.L-1 (5.58%) following training. In the HIG, no absolute or relative changes were found in the HIG's uric acid levels either before or after training. In turn, the MODG decreased their uric acid by a mean of 0.2 nmol.L-1 (50.00%) before training but found no change in levels after training.
With regard to the female TKD athletes, the two-way ANOVA demonstrated a significant interaction for testosterone binding globulin (P = 0.001), total testosterone (P = 0.04), free testosterone (P = 0.04), S-CPK (P = 0.001), and uric acid (P = 0.04).
With regards to total testosterone, the HIG were found to have increased levels before training (mean: 0.2 nmol.L-1; 25.00%) and after training (mean: 0.4 nmol.L-1; 33.33%). In turn, the MODG had no change in their levels before training but did find an increase after training (mean: 0.2 nmol.L-1; 28.57%). Testosterone binding globulin levels were found to be decreased in the HIG before training (mean: 3.4 nmol.L-1; 5.67%) and after training (mean: 2.3 nmol.L-1; 4.18%). This was similar to the MODG, which found decreased levels before training (mean: 1.3 nmol.L-1; 2.64%) and after training (mean: 3.1 nmol.L-1; 6.84%). Following their high-intensity training period, the female HIG athletes increased their free testosterone by a mean of 0.5 pmol.L-1 (6.66%) before training and by a mean of 2.9 pmol.L-1 (47.54%) after training. However, the MODG were found to have decreased their levels by a mean of 0.8 pmol.L-1 (6.89%) before training, followed by a mean increase of 1.9 pmol.L-1 (22.89%) after their training regime. S-CPK levels were found to be increased in the female HIG before training (mean: 5.8 IU.L-1; 1.69%), but increased following their respective training program (mean: 1.7 IU.L-1; 1.30%). The female MODG, however, found increases in their mean S-CPK levels before (mean: 29.3 IU.L-1; 8.55%) and after (mean: 7.5 IU.L-1; 22.67%) training. Mean uric acid levels were found to be increased by 0.1 nmol.L-1 (50.00%) before their training regime and by 0.1 nmol.L-1 (33.33%) after training. In turn, the MODG found no alterations in their uric acid levels before and after their training program.
5. Discussion
Significant post-exercise improvements were found for total testosterone, free testosterone, C/T ratio, and lactate in the mixed gender HIG. With regards to the specific gender effects, post-exercise improvements were found in S-CPK in the male MODG and HIG, with improvements in uric acid only in the male HIG. In turn, post-exercise improvements were found for total testosterone, testosterone binding globulin, free testosterone in the female MODG and HIG, with post-exercise improvements in S-CPK and uric acid in the female HIG only.
The decline in total testosterone before the high-intensity training regime indicates that the HIG experienced the TKD test as a stress. However, following their four weeks of high-intensity concurrent training, the HIG demonstrated an increase, albeit only 0.52%, in their total testosterone after the TKD test. In turn, the MODG were found to have a decreased total testosterone following their four-week training program, indicating a catabolic response to the TKD test. This was the case with regards to free testosterone for the HIG as demonstrated by a 27.51% decrease before training but a 4.13% increase following training. With regards to the C/T ratio, the HIG were found to have a 12.67% decreased ratio before training, indicting a catabolic response to the TKD test, but the four weeks of high-intensity concurrent training resulted in a 6.97% decline in the ratio, indicating a more anabolic response to the TKD test. While the MODG were found to have a decreased C/T ratio and thus catabolic response to the TKD test, both before and after training. This finding may suggest that females may adapt better this mode of concurrent high-intensity training than their male counterparts.
This response of elevated total testosterone and free testosterone levels, as demonstrated in the present study, supports the results from the study of Benedini and colleagues (28), in which ten karateka demonstrated increased testosterone levels following a period of training. In addition, this study is in agreement with the findings of Alghadir and co-workers (29) in which athletes were also subjected to four weeks of aerobic training. The finding of improved testosterone indices in the mixed gender and female HIG is supported by evidence that the higher the intensity and the longer the duration of exercise, the greater the hormonal response (30). Moreover, the adrenal response is also stronger for intermittent anaerobic and aerobic exercise (31), as is utilized in this study. The C/T ratio is commonly utilized as a biomarker of physiological stress and an exercise adaptation index (29, 32). In this study, both the mixed gender and female HIG groups, as well as the female MODG (albeit with a smaller magnitude), were found to have improved their post-exercise C/T ratios. The range of C/T ratio values observed in this study are similar to previous studies (29).
Lactate is an important biomarker of physiological stress in that it indicates the activation of glycolysis in skeletal muscle during exercise and physiological adaptation to exercise (33). As expected, lactate accumulation was substantially greater in the HIG when compared to the MODG following their respective training regimes. This indicates that the mixed gender HIG and MODG (albeit to a smaller magnitude) could better improve contribution of glycolysis to energy demand and also tolerate lactate more effectively (34). This may indeed be the case in the present study that utilized previously-trained TKD athletes and actions involved in TKD, which is a sport characterized by periods of high levels of technical, tactical, psychological, and physical fitness (22). Nevertheless, these results are consistent with the findings of previous studies (35), but contrary to others (36). The contrasting observation may be due to differences in various study design parameters such as sample size, type of test, and duration of the tests used.
While the mixed gender grouping found no alterations in their post-exercise S-CPK and uric acid levels before and after their respective training programs, both the male and female groupings found significant alterations in their S-CPK and uric acid levels after their respective training programs. Since S-CPK can be utilized as a gauge of the level of stress to skeletal muscle (28), it is noteworthy that specifically, in the male grouping, post-exercise S-CPK declines were of a greater magnitude following the high-intensity concurrent training when compared to the moderate- to vigorous-intensity training. In the female TKD athletes, only the HIG demonstrated an improved post-exercise S-CPK response to the TKD test following their four-week high-intensity training. Since uric acid has been identified as a potential endogenous antioxidant, its elevation could be interpreted as an indicator of the mobilization and maintenance of plasma antioxidant status needed during the recovery period after exercise (37). These findings are in line with previous studies (38).
Uric acid is an essential blood biomarker of physiological stress since elevated levels result in a greater risk factor for musculoskeletal damage (7, 39). However, marked increases in plasma uric acid has been reported following high-intensity exercise (39, 40). With regards to gender-specific alterations in post-exercise uric acid levels, while the male HIG found no alterations in their uric acid levels, it appears that the moderate- to vigorous-intensity training provided some adaptation in that a decreased magnitude of increase in uric acid levels was observed following the TKD test. Interestingly, this finding is reversed in the female grouping with the HIG, but not MODG, appearing to gain some adaptation to physiological stress following a reduction in magnitude of post-exercise uric acid level increase following their four weeks of training.
5.1. Limitations
This study is limited by its ecological validity, and as such generalization of the results of this study should be cautioned against. In this regard, due to the novelty of this study, it was necessary to test our new research hypothesis in a small number of participants first. Since an association was indeed found in this study, it is important that larger confirmatory studies be undertaken in future. In addition, the present study trained individuals with different fitness levels at the same percentage of the theoretical HRmax that were not matched at pre-test. However, percentages of maximal heart rate are widely used, especially in low-resource settings, to determine training intensities (41). Further, the present study utilized a repetition range for RT intensity instead of maximal 1-RM testing. This study utilized repetition ranges since they correlate well with estimated load (i.e. % of 1-RM.) (29). In addition, the use of 1-RM testing is contraindicated in novice weight lifters who do not have adequate experience handling free weights, and cannot be later utilized in settings without appropriate facilities, equipment, and qualified personnel and spotters (29). This study also utilized previously trained athletes, and it would be useful to determine the effects of this concurrent training on previously sedentary athletes.
5.2. Conclusions
This study demonstrates that even a short period (i.e., four weeks) of concurrent taekwondo and resistance training improves adaptation and post-exercise responses to physiological stress, and such adaptation may even be more marked in previously-trained female athletes. Thus, this mode of training may provide a novel exercise training modality to rapidly improve an individuals' capacity to exercise, especially in situations of plateau, while avoiding overtraining and an increased concomitant chance of illness and musculoskeletal injury. This may be so since intermittent physical events, such as TKD, may require greater strength and muscle power demands on both the upper- and lower-body with high anaerobic energy demands (37). The data from this study further supports the importance of monitoring post-exercise blood biomarkers of physiological stress in evaluating the effectiveness of different protocols and training responses (37), as well as the need to ensure that training programs are of appropriate design (i.e. concurrent) and sufficient intensity to promote optimal adaptation and reduce musculoskeletal overuse injuries.
References
-
1.
Banfi G, Colombini A, Lombardi G, Lubkowska A. Metabolic markers in sports medicine. Adv Clin Chem. 2012;56:1-54. [PubMed ID: 22397027]. https://doi.org/10.1016/b978-0-12-394317-0.00015-7.
-
2.
Lee EC, Fragala MS, Kavouras SA, Queen RM, Pryor JL, Casa DJ. Biomarkers in sports and exercise: Tracking health, performance, and recovery in athletes. J Strength Cond Res. 2017;31(10):2920-37. [PubMed ID: 28737585]. [PubMed Central ID: PMC5640004]. https://doi.org/10.1519/JSC.0000000000002122.
-
3.
Hug M, Mullis PE, Vogt M, Ventura N, Hoppeler H. Training modalities: over-reaching and over-training in athletes, including a study of the role of hormones. Best Pract Res Clin Endocrinol Metab. 2003;17(2):191-209. [PubMed ID: 12787547]. https://doi.org/10.1016/s1521-690x(02)00104-5.
-
4.
Shariat A, Shaw I, Shaw BS, Sadeghi H. Analysis of the effects of resistance training on circadan rhythm of endocrine hormones. Russian Open Medical Journal. 2015;4(3). https://doi.org/10.15275/rusomj.2015.03.
-
5.
Steinacker JM, Lormes W, Reissnecker S, Liu Y. New aspects of the hormone and cytokine response to training. Eur J Appl Physiol. 2004;91(4):382-91. [PubMed ID: 14608461]. https://doi.org/10.1007/s00421-003-0960-x.
-
6.
Krasilshchikov O, Sungkit NB, Shihabudin TM, Shaw I, Shaw BS. Effects of an eight-week training programme on pain relief and physical condition of overweight and obese women with early stage primary knee osteoarthritis. Afr J Phys Health Educ Recreat Dance. 2011;17(2). https://doi.org/10.4314/ajpherd.v17i2.67669.
-
7.
Smilios I, Pilianidis T, Karamouzis M, Tokmakidis SP. Hormonal responses after various resistance exercise protocols. Med Sci Sports Exerc. 2003;35(4):644-54. [PubMed ID: 12673149]. https://doi.org/10.1249/01.MSS.0000058366.04460.5F.
-
8.
Djarova T, Watson G, Basson A, Grace J, Cloete J, Ramakoaba A. ACTN3 and TNF gene polymorphism association with C-reactive protein, uric acid, lactate and physical characteristics in young African cricket players. Afr J Biochem Res. 2011;5(1):22-7.
-
9.
Shaw BS, Shaw I, Brown GA. Resistance exercise is medicine: Strength training in health promotion and rehabilitation. Int J Ther Rehabil. 2015;22(8):385-9. https://doi.org/10.12968/ijtr.2015.22.8.385.
-
10.
Simon MI, Vanvunakis H. The dye-sensitized photooxidation of purine and pyrimidine derivatives. Arch Biochem Biophys. 1964;105:197-206. [PubMed ID: 14165495]. https://doi.org/10.1016/0003-9861(64)90253-x.
-
11.
Howell RR, Wyngaarden JB. On the mechanism of peroxidation of uric acids by hemoproteins. J Biol Chem. 1960;235:3544-50. [PubMed ID: 13716276].
-
12.
Davies KJ, Sevanian A, Muakkassah-Kelly SF, Hochstein P. Uric acid-iron ion complexes. A new aspect of the antioxidant functions of uric acid. Biochem J. 1986;235(3):747-54. [PubMed ID: 3753442]. [PubMed Central ID: PMC1146751]. https://doi.org/10.1042/bj2350747.
-
13.
Sevanian A, Davies KJ, Hochstein P. Conservation of vitamin C by uric acid in blood. J Free Radic Biol Med. 1985;1(2):117-24. [PubMed ID: 3836238]. https://doi.org/10.1016/0748-5514(85)90015-7.
-
14.
Groussard C, Morel I, Chevanne M, Monnier M, Cillard J, Delamarche A. Free radical scavenging and antioxidant effects of lactate ion: An in vitro study. J Appl Physiol (1985). 2000;89(1):169-75. [PubMed ID: 10904049]. https://doi.org/10.1152/jappl.2000.89.1.169.
-
15.
McCaulley GO, McBride JM, Cormie P, Hudson MB, Nuzzo JL, Quindry JC, et al. Acute hormonal and neuromuscular responses to hypertrophy, strength and power type resistance exercise. Eur J Appl Physiol. 2008;105(5):695-704. [PubMed ID: 19066934]. https://doi.org/10.1007/s00421-008-0951-z.
-
16.
Vatansever-Ozen S, Tiryaki-Sonmez G, Bugdayci G, Ozen G. The effects of exercise on food intake and hunger: Relationship with acylated ghrelin and leptin. J Sports Sci Med. 2011;10(2):283-91. [PubMed ID: 24149873]. [PubMed Central ID: PMC3761859].
-
17.
Haddad M, Chaouachi A, Wong del P, Castagna C, Hue O, Impellizzeri FM, et al. Influence of exercise intensity and duration on perceived exertion in adolescent Taekwondo athletes. Eur J Sport Sci. 2014;14(Suppl 1):S275-81. [PubMed ID: 24444218]. https://doi.org/10.1080/17461391.2012.691115.
-
18.
Shariat A, Shaw BS, Kargarfard M, Shaw I, Lam ETC. Kinanthropometric attributes of elite male Judo, Karate and Taekwondo athletes. Rev Bras Med Esporte. 2017;23(4):260-3. https://doi.org/10.1590/1517-869220172304175654.
-
19.
Bridge CA, Ferreira da Silva Santos J, Chaabene H, Pieter W, Franchini E. Physical and physiological profiles of taekwondo athletes. Sports Med. 2014;44(6):713-33. [PubMed ID: 24549477]. https://doi.org/10.1007/s40279-014-0159-9.
-
20.
Pilz-Burstein R, Ashkenazi Y, Yaakobovitz Y, Cohen Y, Zigel L, Nemet D, et al. Hormonal response to Taekwondo fighting simulation in elite adolescent athletes. Eur J Appl Physiol. 2010;110(6):1283-90. [PubMed ID: 20803154]. https://doi.org/10.1007/s00421-010-1612-6.
-
21.
Murphy JC, Nagle E, Robertson RJ, Mccrory JL. Effect of single set dynamic and static stretching exercise on jump height in college age recreational athletes. Int J Exerc Sci. 2010;3(4):8.
-
22.
Shaw BS, Mathunjwa ML, Djarova-Daniels TG, Shariat A, Shaw I. Hematologic responses following concurrent Taekwondo and resistance training. South Afri J Res Sport Phys Educ Recr. 2020;42(1):47-56.
-
23.
American College of Sports Medicine (Acsm). ACSM's health-related physical fitness assessment manual. 4th ed. Philadelphia, USA: Lippincott Williams & Wilkins; 2013.
-
24.
Shaw BS, Shaw I, Brown GA. Comparison of resistance and concurrent resistance and endurance training regimes in the development of strength. J Strength Cond Res. 2009;23(9):2507-14. [PubMed ID: 19910823]. https://doi.org/10.1519/JSC.0b013e3181bc191e.
-
25.
Hackett DA, Amirthalingam T, Mitchell L, Mavros Y, Wilson GC, Halaki M. Effects of a 12-week modified German volume training program on muscle strength and hypertrophy-a pilot study. Sports (Basel). 2018;6(1). [PubMed ID: 29910312]. [PubMed Central ID: PMC5969184]. https://doi.org/10.3390/sports6010007.
-
26.
Wasserman L. Hypothesis testing and P-values. All of statistics: A concise course in statistical inference. London, UK: Springer; 2004. p. 149-73.
-
27.
Kidney Disease Improving Global Outcomes Blood Pressure Work Group. KDIGO 2021 clinical practice guideline for the management of blood pressure in chronic kidney disease. Kidney Int. 2021;99(3S):S1-S87. [PubMed ID: 33637192]. https://doi.org/10.1016/j.kint.2020.11.003.
-
28.
Benedini S, Longo S, Caumo A, Luzi L, Invernizzi PL. Metabolic and hormonal responses to a single session of kumite (free non-contact fight) and kata (highly ritualized fight) in karate athletes. Sport Sci Health. 2012;8(2-3):81-5. [PubMed ID: 23440907]. [PubMed Central ID: PMC3573715]. https://doi.org/10.1007/s11332-012-0132-7.
-
29.
Alghadir AH, Gabr SA, Aly FA. The effects of four weeks aerobic training on saliva cortisol and testosterone in young healthy persons. J Phys Ther Sci. 2015;27(7):2029-33. [PubMed ID: 26311920]. [PubMed Central ID: PMC4540811]. https://doi.org/10.1589/jpts.27.2029.
-
30.
de Souza Duarte N, de Almeida Corrêa LM, Assunção LR, de Menezes AA, de Castro OB, Teixeira LF. Relation between depression and hormonal dysregulation. Open Journal of Depression. 2017;6(3):69-78. https://doi.org/10.4236/ojd.2017.63005.
-
31.
Czuba M, Wilk R, Karpinski J, Chalimoniuk M, Zajac A, Langfort J. Intermittent hypoxic training improves anaerobic performance in competitive swimmers when implemented into a direct competition mesocycle. PLoS One. 2017;12(8). e0180380. [PubMed ID: 28763443]. [PubMed Central ID: PMC5538675]. https://doi.org/10.1371/journal.pone.0180380.
-
32.
Pourvaghar MJ, Ghaeini AA, Ravasi AA, Kordi MR. Effects of training time on serum immunoglobulin alterations and cortisol testosterone responses in male athlete students. Biology of Sport. 2010;27(1):25-8. https://doi.org/10.5604/20831862.907783.
-
33.
Jabbour G, Iancu HD, Paulin A. Effects of high-intensity training on anaerobic and aerobic contributions to total energy release during repeated supramaximal exercise in obese adults. Sports Med Open. 2015;1(1):36. [PubMed ID: 26512339]. [PubMed Central ID: PMC4612325]. https://doi.org/10.1186/s40798-015-0035-7.
-
34.
Hur S, Han GS, Cho BJ. Changes in glucose, TNF-alpha and IL-6 blood levels in middle-aged women associated with aerobic exercise and meditation training. J Phys Ther Sci. 2014;26(12):1933-6. [PubMed ID: 25540501]. [PubMed Central ID: PMC4273061]. https://doi.org/10.1589/jpts.26.1933.
-
35.
Conway KJ, Orr R, Stannard SR. Effect of a divided caffeine dose on endurance cycling performance, postexercise urinary caffeine concentration, and plasma paraxanthine. J Appl Physiol (1985). 2003;94(4):1557-62. [PubMed ID: 12482764]. https://doi.org/10.1152/japplphysiol.00911.2002.
-
36.
Davis JM, Murphy EA, Carmichael MD, Zielinski MR, Groschwitz CM, Brown AS, et al. Curcumin effects on inflammation and performance recovery following eccentric exercise-induced muscle damage. Am J Physiol Regul Integr Comp Physiol. 2007;292(6):R2168-73. [PubMed ID: 17332159]. https://doi.org/10.1152/ajpregu.00858.2006.
-
37.
Margonis K, Fatouros IG, Jamurtas AZ, Nikolaidis MG, Douroudos I, Chatzinikolaou A, et al. Oxidative stress biomarkers responses to physical overtraining: Implications for diagnosis. Free Radic Biol Med. 2007;43(6):901-10. [PubMed ID: 17697935]. https://doi.org/10.1016/j.freeradbiomed.2007.05.022.
-
38.
Passelergue PA, Lac G. Salivary hormonal responses and performance changes during 15 weeks of mixed aerobic and weight training in elite junior wrestlers. J Strength Cond Res. 2012;26(11):3049-58. [PubMed ID: 22207258]. https://doi.org/10.1519/JSC.0b013e3182473e3d.
-
39.
Svensson MB, Ekblom B, Cotgreave IA, Norman B, Sjoberg B, Ekblom O, et al. Adaptive stress response of glutathione and uric acid metabolism in man following controlled exercise and diet. Acta Physiol Scand. 2002;176(1):43-56. [PubMed ID: 12193218]. https://doi.org/10.1046/j.1365-201X.2002.01008.x.
-
40.
Hellsten-Westing Y, Balsom PD, Norman B, Sjodin B. The effect of high-intensity training on purine metabolism in man. Acta Physiol Scand. 1993;149(4):405-12. [PubMed ID: 8128888]. https://doi.org/10.1111/j.1748-1716.1993.tb09636.x.
-
41.
Shaw BS, Shaw I. Resistance training as a countermeasure for key non-communicable diseases in low-resource settings: A review. Asian J Sports Med. 2020;12(1). https://doi.org/10.5812/asjsm.106588.