Abstract
Objectives:
The aim of this study was to evaluate the validity and reliability of introducing a new activity-based balance index using a triaxial accelerometer during activity.Methods:
Twenty seven soccer players (age: 14.5 ± 0.4 years old, body mass: 58.3 ± 9.3 kg, height: 172 ± 8 cm) who participated in the national premier league were recruited. The participants were tested for their balance, activity, and skill in four tests: (I) one leg stance; (II) dynamic Y balance; (III) running; and (IV) dribbling slalom. The acceleration of the body was recorded using an accelerometer during those tests. By processing acceleration data, a new activity-based balance index (ABI) was calculated based on the velocity, acceleration, and position index of soccer players. Using intra-class correlation coefficients (ICC), reliability was calculated.Results:
Reliability was high (ICC = 0.87 - 0.89; 95% CI = 0.77 - 0.93) in calculating ABI for the three activities performed in the tests. A significant positive correlation between ABI and both static and dynamic balance scores (r = 0.62, P = 0.002) was observed. Furthermore, negative correlation was found significantly between ABI and dribbling scores (r = -0.61, P = 0.026) and Y Balance test (r = 0.6, P = 0.002).Conclusions:
In conclusion, the introduced ABI demonstrates great potential to determine balance and skill scores based on accelerometer-based measures.Keywords
Biomechanical Phenomena Postural Balance Acceleration Football Performance
1. Background
In classical mechanics, balance is defined as the sum of all forces and torques put on the object, such that it equates to zero (1). Regarding the location of the forces of weight and ground reaction in a standing position, balance can be defined as lesser displacement of the center of pressure (COP) around the balance point (1-3). In human actions, such as walking, and running the oscillations of the COP around the foot axis can be referenced as an indicator of balance (2). In biomechanics stability may be defined as resistance to balance changes (4); whilst the balance is defined as the ability to maintain a center of gravity in the COP zone between two legs (2, 3). The internal factors that affect balance are sensory information received from the sensory system, the visual and vestibular system, and motor responses that affect coordination, range of motion, and strength, respectively (5). Evidently, balance is a complex phenomenon, especially during the execution of complex motor skills (6). Thus, the objective assessment of balance during complex activities is crucial. Among other assessment approaches (e.g., static and dynamic balance measurement), the oscillation of COP around a desired point or path is a potentially useful indicator of balance, that can be conducted in an easy and accurate manner (7, 8). Therefore, as a functional assessment of balance it seems that estimating COP oscillation during an activity by accelerometer could be used. The oscillation of COP over the foot axis during walking may be considered as a balance index. Because this oscillation could be intuitively and objectively understood, we defined a new activity-based balance index. Oscillations of movement-related parameters over their optimal values (Figure 1, solid line) were quantitatively calculated. The movement-related parameters consisted of the object position and its first and second derivations, known as velocity and acceleration. Each of these parameters is asserted to have a specific application based on the type of the activity. To the best of our knowledge, there is no data about this hypothesis.
Schematic representation of activity-based balance index (Equation 1)
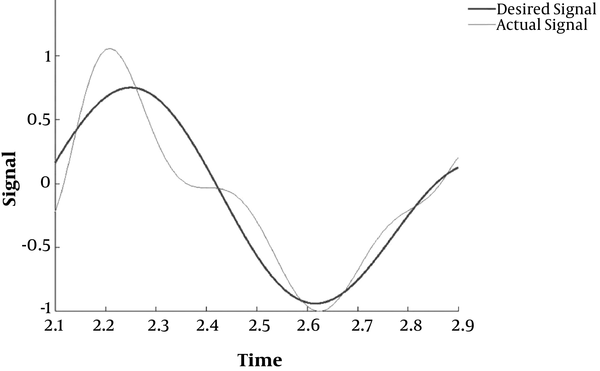
Furthermore, numerous tests such as Y balance, functional reach, and Berg balance tests are often used to measure balance and stability in laboratory and field conditions (9). Generally, force plates are regarded as the gold standard to measure balance and stability in human activities (10). However, the complicated mounting requirements in specific spaces and high cost of force plates limit their applicability in many situations. Luckily, there are alternative instruments and methods that can be used to get valid and reliable information about balance and stability (11, 12); such as a tri-axial accelerometer (13-15). Following the extraction of raw accelerometer data, it is possible to assess balance in many sport skills (16). Moreover, given their ubiquity, accelerometers are reasonably cost-efficient, are non-invasive, and, generally, easy to use. Seimetz et al. (4) compared the accelerometer data and force plate and reported that there was no statistically significant difference between them in postural stability measurement. The authors concluded that using the accelerometer as a simple and practical method for measuring balance can be recommended. However, it should be noted that, in the study of Seimetz et al. (4), only static balance was measured, and no measurement during an activity or skill was performed.
On the other hand, soccer represents one of the most popular sports in the world. Players perform a variety of activities such as omnidirectional running with different speeds, jumping, turning around, tackling, and throwing (17). Players’ capabilities are also affected by psychological and emotional stress during competition or gameplay (18). Thus, collectively, there are a variety of factors that can contribute to injuries among players (19-21). Some studies suggest that lack of postural control among soccer players is one of the most important factors for injuries (19); indeed, lack of postural control during soccer contributes to falling, which can result in injury. Therefore, the ability to accurately and while performing a skill, easily assess the balance of a player is of great importance and utility to coaches and players, particularly in the prediction and prevention of sports injuries.
2. Objectives
Given the distinct lack of appropriate studies in this field, the present study aimed to firstly introduce a novel index for evaluating the balance during activity using an accelerometer, and subsequently, to investigate the validity and reliability of the novel index in assessing the balance in static, dynamic, and soccer performance balance.
3. Methods
To calculate ABI, our four tests included static balance running on Footscan, Y dynamic balance, and dribbling slalom test were used. For test-retest validity, all tests were performed twice, separated by a period of one week, and performed at the same time of the day for each test. Also, using a tri-axial-accelerometer (1600Hz, ± 16 g, 13 bit, Didepardaz, Iran), the acceleration of players was recorded. It was attached to the skin on the lumbar region of the back.
3.1. Participants
Twenty-seven junior male soccer players of the U-14 and U-15 age group took part in the present study. The players’ training routine included three training sessions (90 minutes per session) with one match per week. The inclusion criteria were: (1) more than 3 years training experience; (2) no recent muscular or skeletomuscular injuries; (3) no use of sports supplements, anti-inflammatory drugs, and medication at least 6 months prior to the study. Exclusion criteria included balance disorders, and other risk factors based on a doctor’s examination. All participants were familiar with the test. All participants signed the informed consent form and were informed about the experimental protocol.
Participants’ anthropometric and professional experience data are shown in Table 1.
Values | |
---|---|
Numbers | 27 |
Age, y | 14.5 ± 0.45 |
Mass, kg | 58.0 ± 9.3 |
Height, cm | 172.2 ± 8.2 |
Professional Experience in, y | 4.5 |
3.2. Calculating the Balance Index
The oscillation of COP over the foot axis during walking may be considered as a balance index. To quantify the activity-based balance index, we developed the following equation:
Where S(t) and A(t) denote the optimal signal and the actual measured signal, respectively (discussed later). The difference between S(t) and A(t) was considered as a sub-index. The number of sub-indices was determined by two frequencies defined as the frequency of index (fs) and the frequency of activity (fa). Subsequently, the activity-based balance index was defined as the average value of these sub-indices during the complete time of the activity (3). The so-called activity-based balance index was defined as the percentage of optimal signal.
For computing the activity-based balance index, we initially measured movement-related signals. The S(t) and A(t) values were calculated via the values of movement-related signals.
According to the definition of balance, the optimal value of the signal (S) is the situation of movement variable over longer time intervals, i.e. the overall trend or behavior of a movement variable, which is obtained by a lower degree curve fitting of the S signal.
Although the main signal is recorded at a high frequency, this necessitates the filtering of the main signal using an appropriate low pass filter, where the frequency value is dependent on the activity or skill type. The next step is to define the frequency of activity (A) signal, which represents the movement variable at a high frequency. Whatever higher frequency is selected, the quantity of oscillations around the S signal is higher, and consequently, a higher activity balance index is calculated. Similarly, defining the appropriate frequency for the A signal was performed according to movement or skill type and the characteristics of participants. Therefore, there were two frequencies (low and high) called activity and index frequencies, reported as fa and fs. Thus, whenever the ratio of fa/fs is greater, the numerical value of the activity index is increased (3).
3.3. Static and Dynamic Tests
3.3.1. Static Balance Test
The static balance test was performed by means of a foot scan (plate 0.5 m, 300 Hz, RSscan, Belgium) that measured the displacements of COP. The tests were conducted in a gym free from external distractions (22). One training trial was allowed before data collection (22).
3.3.2. Running Test
Participants ran at 4 m/s ± 4% over a Footscan (plate 0.5 m, 300Hz, RSscan, Belgium) that recorded the displacements of the center of foot pressure (COP) (23). The experimental gym was 40 m long. Running velocity was quantified using timing gates (Arena Gear, RM-501-TrackNField-2G, USA). During practice trials, the starting location of the run was recorded, allowing the position to be identified that ensured that the participants struck the force or pressure transducer using their dominant foot with their fourth foot strike. Participants completed three successful trials (23).
3.3.3. The Y Balance Test
The Y Balance test is a measure of dynamic balance in a unilateral stance that has been deemed to be reliable and valid (24). The participant reaches with one foot in the anterior, posteromedial, and posterolateral directions while standing on the other foot on a centralized stance platform (24). The test is performed barefoot with both left and right limbs. Each participant performed six practice trials before the three data-collection trials. Subjects were instructed to reach as far as they could while maintaining their balance, with the stance-foot toes immediately behind the start line (24). All raters were trained in performing the Y Balance test protocol (24). The reach distance in each direction was normalized to the height length. The sum of 3 normalized reach distances was then averaged and multiplied by 100 to generate a composite score (24).
3.3.4. Dribbling Slalom Test
Participants did dribbling by zigzagging between 6 cones, with intervals of 2 m (25) along a straight line of 10 m. The time of dribbling was measured using a stopwatch (25).
3.4. Data Analysis
According to the ABI definition, the assessment signal included acceleration and position. The acceleration data were recorded by a tri-axial-accelerometer (1600 Hz, ± 16 g, 13 bit, Didepardaz, Iran) and integrated mathematically to calculate velocity and position. According to Equation 1 to calculate the corresponding activity-based index, using MATLAB software, the raw data of these signals were processed.
3.5. Statistical Analyses
To evaluate the validity of ABI. a test-retest condition was performed. The intra-test reliability was measured by using the intra-class correlation test. The interclass correlation coefficient (ICC) was used to examine the reliability of the ABI over three trials. ICC results were interpreted as the classification scale: very high (0.90 - 1.00), high (0.70 - 0.89), moderate (0.50 - 0.69), and low (0.26 - 0.49) (26). The relationship between the calculated indices and COP, score, and time, respectively, was calculated using correlation coefficients. The validity of ABI was determined using Pearson’s correlation. Data are presented as means with standard deviation.
4. Results
Table 2 shows the average COP movement in AP and ML directions, dribbling time, running, and Y balance tests.
Results of Four Tests
Parameters | Mean (SD) | Measurement Unit |
---|---|---|
Dribbling time | 12.7 (1.0) | Second |
Static balance | ||
COP movement In AP direction | 117.0 (66.2) | Millimeter |
COP movement in ML Direction | 78.2 (75.4) | Millimeter |
Total COP movement | 1041.8 (501.2) | Millimeter |
Y balance | ||
Anterior direction score | 51.9 (7.4) | % of height |
Posterior lateral direction score | 63.2 (5.5) | % of height |
Posterior medial direction score | 61.3 (6.2) | % of height |
overall score | 58.8 (5.5) | % of height |
Running | ||
ML COP movement for right support foot | 31.2 (9.4) | Millimeter |
AP COP movement for right support foot | 224.3 (52.6) | Millimeter |
ML COP movement for left support foot | 36.7 (11.3) | Millimeter |
AP COP movement for left support foot | 233.2 (56.8) | Millimeter |
To demonstrate the effect of selecting different values as the index and activity frequencies, which are selected according to the type of activity, Table 3 shows the result of calculating indices for different frequencies.
Mean of Various Indices During Static Balance Test According to Different Frequencies
Activity Frequency, Hz | 5 | 5 | 2 |
---|---|---|---|
Index Frequency, Hz | 500 | 1000 | 500 |
Acceleration index | |||
AP direction | 158.2 | 158.6 | 214.4 |
ML direction | 172.3 | 172.7 | 219.1 |
Vertical | 221.8 | 222.3 | 331.6 |
Resultant | 232.1 | 232.7 | 358.2 |
Velocity index | |||
AP direction | 8.9 | 8.9 | 16.4 |
ML direction | 8.3 | 8.3 | 14.9 |
Vertical | 15.2 | 15.2 | 26.4 |
Resultant | 15.8 | 15.8 | 27.8 |
Position index | |||
AP direction | 0.41 | 0.41 | 1.4 |
ML direction | 0.39 | 0.39 | 1.3 |
Vertical | 0.81 | 0.81 | 2.1 |
Resultant | 0.82 | 0.82 | 2.2 |
The correlation coefficient of COP movements with indices calculated from acceleration and position are detailed in Table 4. Whilst in Table 5 movement-related indices and corresponding results are shown. A significant positive correlation between ABI and both static and dynamic balance scores (r = 0.62, P = 0.002) was observed. Furthermore, a negative correlation was found significantly between ABI and dribbling scores (r = -0.61, P = 0.026) and Y Balance test (r = 0.6, P = 0.002), respectively. This indicates that ABI is a valid index.
The Correlation Coefficients Between Indices and COP Movements
The Correlation Coefficients Between Activity Tests and ABI Indices
Dynamic Tests | CC (Correlation Coefficient) |
---|---|
Dribbling | |
Balance index and dribbling time | -0.61a |
Balance index and dribbling | -0.61a |
Y Balance | |
Balance index and Y balance score in AP direction | 0.60b |
Balance index and total score of Y balance | 0.57a |
Running | |
Balance index and ML COP movement | 0.61b |
Balance index and ML COP movement | 0.65a |
Table 6 shows high (0.873 - 0.924) interclass correlation coefficients and 95% confidence interval between 3 trial measurements in ABI in dribbling slalom, running, Y Balance and static balance tests. This indicates that ABI is reliable index.
Interclass Correlation Coefficients and 95% Confidence Interval Between Selected Indices
ICC | 95% Confidence Interval | F Test with True Value 0 | |||
---|---|---|---|---|---|
Lower Bound | Upper Bound | Values | Sig | ||
ABI static balance | 0.894 | 0.888 | .897 | 485.971 | 0.000 |
ABI Y balance | 0.924 | 0.862 | 0.962 | 37.259 | 0.000 |
ABI running | 0.879 | 0.860 | 0.889 | 137.948 | 0.000 |
ABI dribbling slalom | 0.873 | 0.776 | 0.935 | 21.593 | 0.000 |
5. Discussion
The aim of this study was to evaluate the validity and reliability of introducing a new activity-based balance index using a triaxial accelerometer during activity. The results showed high reliability (ICC = 0.87 - 0.89; 95% CI = 0.77 - 0.93) in calculating ABI for three trial performed all tests. A significant positive correlation between ABI and both static and dynamic balance scores (r = 0.62, P = 0.002) was observed. Further, negative correlation was found significantly between ABI and dribbling scores (r = -0.61, P = 0.026) and Y Balance test (r = 0.6, P = 0.002), respectively. The introduced ABI demonstrates great potential to determine balance and skill scores based on accelerometer-based measures. This indicates that ABI is a valid and reliable index for assessing balance during activity. In line with our data, Clark et al. (16) showed that the Slam Tracker was demonstrated to be an accurate and reliable device for measuring the raw accelerations of movement. Also, Noamani et al. (11) investigated the validity of using wearable inertial sensors for assessing the dynamics of standing balance and showed that accelerometers are accurate and recommended for standing balance evaluation. Interestingly there was a negative correlation between ABI and the dribbling time. This indicates that as the dribbling time shortens, the score increases, which means that performance is better. The results of this study also suggest that, as soccer players have more fluctuations in the range of their base of support, performance, assessed by soccer-specific assessments, is improved. In order to determine the most appropriate activity and index frequencies, further studies should be conducted to identify and refine indicators of better performance in each activity. Generally, if the goal is to determine the index needed to demonstrate the differences between player skills, increasing the frequency of the actual measured signal, i.e. frequency of activity, can continue to the extent that the index shows a meaningful difference between the heterogeneous groups.
Previous studies show that many components of physical fitness directly increase the performance-related ability of sports players. Elite athletes usually have greater speed, acceleration, agility, strength, anaerobic power, aerobic fitness, and better technical skills than semi-skilled athletes (27, 28). Furthermore, the components of physical fitness, agility and speed, are regarded as the main reason for the difference between elite and semi-skilled male soccer players (27). Indeed, in soccer, agility, concomitant with the ball carrying skills, is the most important predictor of skilled and elite execution (29). Moreover, players need to maintain good balance when controlling a ball with one leg, when landing, and when defending against an attacker; importantly, this ability requires excellent coordination. Although balance is defined by the ability to maintain the center of gravity within the bounds of reliance, during sport activities, the center of gravity is continuously fluctuating in the limit of reliance. Therefore, the brain receives feedback from different systems, including proprioceptive, vestibular, and vision; then processes these data to control the balance (27). From these, the body-feedback system provides feedback from neural systems in ligaments, joint capsules, skin, and muscle tissue. The proprioceptive system is part of this system, which relies on a special feedback from its position and motion. This feedback system continuously receives intra-data and allows soft movements during a challenge to complete a specific nervous system (27).
On the other hand, athletes should not only be able to maintain his balance, but should also be effective in skills which require dynamic balance. Indeed, field-based athletes are rarely in the state of good balance during the competition; in fact, there are many exercises such as capturing, knocking, and throwing the ball, which are performed with reduced contact with the ground. Elite athletes seem to be able to produce stronger movements while maintaining their central body stability. Although in soccer, the lower limbs are used, the central body should also have enough power to produce the desired force, and the highest amount of productive force is generated when the body center is in optimal conditions biomechanically (30).
Agility is the reaction that is defined by the ability to move the entire body along with the change of speed or direction in response to a stimulus (31). Although there are a few studies on reactivity agility (31), it seems that soccer players require good reactions and agility. When the player accelerates, it is necessary to respond effectively to different environmental stimuli. Therefore, when acceleration, speed, or position fluctuations are in the range of individual reliance, the agility will likely improve.
Previous studies have shown that static and dynamic balances are important factors in the implementation of sports skills and techniques. Previous reports have highlighted that static and dynamic balance are directly related to sport performance. Indeed, some researchers have shown that soccer players have a better and more stable static and dynamic balance than non-athletes or beginners (27, 32, 33). According to recent investigations, balance represents a unique concern according to each specific performance or activity. Indeed, due to the diversity of sports and the open and closed chain skills, traditional balance tests may not be appropriate for all athletic performance. The advancement of technology provides some portable and inexpensive laboratory equipment that could be used to assess balance ability thoroughly. The accelerometer is one of the most pervasive pieces of equipment used by researchers; particularly because they are small, portable devices which are easily attached to athletes’ body or clothing (34).
Indeed, it is possible to calculate the velocity and position of an object from acceleration data during an activity or skill by using mathematical equations. Since acceleration, velocity, and position time series data vary during an activity or skill, it is possible to apply the well-known, or introduce novel, mathematical methods that provide a better and more robust interpretation of these data. Therefore, the activity-based balance index was defined as a new mathematical formula and evaluated in a real-life situation in this study.
We acknowledge the limitation of this study. Because this study was conducted in a real context, the sample size for such correlation studies is limited and relatively few variables were studied. Conversely, the strength of this study was the use of raw accelerometer data, to introduce new and appropriate quantitative indices for balance assessment. Finally, it seems that by using modern, low-cost technologies and further quantitative indices, the traditional view of balance could be changed. This could be useful to many sports and, indeed, in the assessment of motor skill. Despite the novel addition to the literature this study has provided, more studies are needed to evaluate ABI for different activities according to the skill level of the individuals. In this case, it is possible to compare players at different levels of skill and provide appropriate normative data.
5.1. Practical Implications
The present study introduces a new balance index, manifest from accelerometer data, and highlights its utility in assessing the skill level of soccer players. These findings may help coaches and athletes to easily and objectively collect useful data for training, competition, and injury monitoring and prevention. However, given this is the first time this index has been reported, further work is required to refine and develop its utility.
5.2. Conclusions
In conclusion, the introduced ABI is a valid and reliable index and demonstrates great potential to determine balance and skill scores, based on accelerometer-based measures. However, given this is the first time this index has been reported, further work is required to refine and develop its utility.
Acknowledgements
References
-
1.
Meriam JL, Kraige LG. Engineering mechanics: dynamics. John Wiley & Sons; 2012.
-
2.
LeVeau BF. Biomechanics of human motion: basics and beyond for the health professions. Slack Incorporated; 2010.
-
3.
Lenjannejadian S, Reisi J, Salimi M, editors. Introducing a New Activity-Based Balance Index Using Accelerometer Data and Evaluating It as a Predictor of Skill Level among Elite Junior Soccer Players. The 13th Conference of the International Sports Engineering Association. 2020.
-
4.
Seimetz C, Tan D, Katayama R, Lockhart T. A comparison between methods of measuring postrual stability: force plates versus accelerometers. Biomed Sci Instrum. 2012;48:386-92. [PubMed ID: 22846310]. [PubMed Central ID: PMC3716367].
-
5.
Bressel E, Yonker JC, Kras J, Heath EM. Comparison of static and dynamic balance in female collegiate soccer, basketball, and gymnastics athletes. J Athl Train. 2007;42(1):42-6. [PubMed ID: 17597942]. [PubMed Central ID: PMC1896078].
-
6.
Hilbun AL. Strategies of Balancing: Regulation of Posture as a Complex Phenomenon. Electronic Theses and Dissertations. 2016.
-
7.
Matsuda S, Demura S, Demura T. Examining differences between center of pressure sway in one-legged and two-legged stances for soccer players and typical adults. Percept Mot Skills. 2010;110(3 Pt 1):751-60. [PubMed ID: 20681329]. https://doi.org/10.2466/PMS.110.3.751-760.
-
8.
Thompson LA, Badache M, Cale S, Behera L, Zhang N. Balance Performance as Observed by Center-of-Pressure Parameter Characteristics in Male Soccer Athletes and Non-Athletes. Sports (Basel). 2017;5(4). [PubMed ID: 29910446]. [PubMed Central ID: PMC5969037]. https://doi.org/10.3390/sports5040086.
-
9.
Winter DA. Human balance and posture control during standing and walking. Gait Posture. 1995;3(4):193-214. https://doi.org/10.1016/0966-6362(96)82849-9.
-
10.
Huurnink A, Fransz DP, Kingma I, van Dieen JH. Comparison of a laboratory grade force platform with a Nintendo Wii Balance Board on measurement of postural control in single-leg stance balance tasks. J Biomech. 2013;46(7):1392-5. [PubMed ID: 23528845]. https://doi.org/10.1016/j.jbiomech.2013.02.018.
-
11.
Noamani A, Nazarahari M, Lewicke J, Vette AH, Rouhani H. Validity of using wearable inertial sensors for assessing the dynamics of standing balance. Med Eng Phys. 2020;77:53-9. [PubMed ID: 31926830]. https://doi.org/10.1016/j.medengphy.2019.10.018.
-
12.
Dewan BM, Roger James C, Kumar NA, Sawyer SF. Kinematic Validation of Postural Sway Measured by Biodex Biosway (Force Plate) and SWAY Balance (Accelerometer) Technology. Biomed Res Int. 2019;2019:8185710. [PubMed ID: 31930140]. [PubMed Central ID: PMC6942738 publication of this paper]. https://doi.org/10.1155/2019/8185710.
-
13.
Mayagoitia RE, Nene AV, Veltink PH. Accelerometer and rate gyroscope measurement of kinematics: an inexpensive alternative to optical motion analysis systems. J Biomech. 2002;35(4):537-42. [PubMed ID: 11934425]. https://doi.org/10.1016/s0021-9290(01)00231-7.
-
14.
Howell D, Osternig L, Chou LS. Monitoring recovery of gait balance control following concussion using an accelerometer. J Biomech. 2015;48(12):3364-8. [PubMed ID: 26152463]. https://doi.org/10.1016/j.jbiomech.2015.06.014.
-
15.
Bourke AK, van de Ven P, Gamble M, O'Connor R, Murphy K, Bogan E, et al. Evaluation of waist-mounted tri-axial accelerometer based fall-detection algorithms during scripted and continuous unscripted activities. J Biomech. 2010;43(15):3051-7. [PubMed ID: 20926081]. https://doi.org/10.1016/j.jbiomech.2010.07.005.
-
16.
Clark CC, Barnes CM, Holton M, Summers HD, Stratton G. SlamTracker Accuracy under Static and Controlled Movement Conditions. Sport Sci Rev. 2016;25(5-6):374-83. https://doi.org/10.1515/ssr-2016-0020.
-
17.
Castagna C, D'Ottavio S, Abt G. Activity profile of young soccer players during actual match play. J Strength Cond Res. 2003;17(4):775-80. [PubMed ID: 14636107]. https://doi.org/10.1519/1533-4287(2003)017<0775:apoysp>2.0.co;2.
-
18.
Kinugasa T, Kilding AE. A comparison of post-match recovery strategies in youth soccer players. J Strength Cond Res. 2009;23(5):1402-7. [PubMed ID: 19620926]. https://doi.org/10.1519/JSC.0b013e3181a0226a.
-
19.
Soderman K, Alfredson H, Pietila T, Werner S. Risk factors for leg injuries in female soccer players: a prospective investigation during one out-door season. Knee Surg Sports Traumatol Arthrosc. 2001;9(5):313-21. [PubMed ID: 11685365]. https://doi.org/10.1007/s001670100228.
-
20.
Alentorn-Geli E, Myer GD, Silvers HJ, Samitier G, Romero D, Lazaro-Haro C, et al. Prevention of non-contact anterior cruciate ligament injuries in soccer players. Part 2: a review of prevention programs aimed to modify risk factors and to reduce injury rates. Knee Surg Sports Traumatol Arthrosc. 2009;17(8):859-79. [PubMed ID: 19506834]. https://doi.org/10.1007/s00167-009-0823-z.
-
21.
Murphy DF, Connolly DA, Beynnon BD. Risk factors for lower extremity injury: a review of the literature. Br J Sports Med. 2003;37(1):13-29. [PubMed ID: 12547739]. [PubMed Central ID: PMC1724594]. https://doi.org/10.1136/bjsm.37.1.13.
-
22.
Ricotti L, Ravaschio A. Break dance significantly increases static balance in 9 years-old soccer players. Gait Posture. 2011;33(3):462-5. [PubMed ID: 21251832]. https://doi.org/10.1016/j.gaitpost.2010.12.026.
-
23.
Sinclair J, Hobbs SJ, Taylor PJ, Currigan G, Greenhalgh A. The influence of different force and pressure measuring transducers on lower extremity kinematics measured during running. J Appl Biomech. 2014;30(1):166-72. [PubMed ID: 24676524]. https://doi.org/10.1123/jab.2012-0238.
-
24.
Butler RJ, Southers C, Gorman PP, Kiesel KB, Plisky PJ. Differences in soccer players' dynamic balance across levels of competition. J Athl Train. 2012;47(6):616-20. [PubMed ID: 23182008]. [PubMed Central ID: PMC3499884]. https://doi.org/10.4085/1062-6050-47.5.14.
-
25.
Gelen E. Acute effects of different warm-up methods on sprint, slalom dribbling, and penalty kick performance in soccer players. J Strength Cond Res. 2010;24(4):950-6. [PubMed ID: 20300033]. https://doi.org/10.1519/JSC.0b013e3181cb703f.
-
26.
Rabbani A, Kargarfard M, Twist C. Reliability and Validity of a Submaximal Warm-up Test for Monitoring Training Status in Professional Soccer Players. J Strength Cond Res. 2018;32(2):326-33. [PubMed ID: 29210958]. https://doi.org/10.1519/JSC.0000000000002335.
-
27.
Dolan K. Reactive agility, core strength, balance, and soccer performance. Ithaca College Theses; 2013.
-
28.
Reilly T, Williams AM, Nevill A, Franks A. A multidisciplinary approach to talent identification in soccer. J Sports Sci. 2000;18(9):695-702. [PubMed ID: 11043895]. https://doi.org/10.1080/02640410050120078.
-
29.
Re AH, Correa UC, Bohme MT. Anthropometric characteristics and motor skills in talent selection and development in indoor soccer. Percept Mot Skills. 2010;110(3 Pt 1):916-30. [PubMed ID: 20681343]. https://doi.org/10.2466/PMS.110.3.916-930.
-
30.
Oliver GD, Di Brezzo R. Functional balance training in collegiate women athletes. J Strength Cond Res. 2009;23(7):2124-9. [PubMed ID: 19855341]. https://doi.org/10.1519/JSC.0b013e3181b3dd9e.
-
31.
Gabbett T, Benton D. Reactive agility of rugby league players. J Sci Med Sport. 2009;12(1):212-4. [PubMed ID: 18069064]. https://doi.org/10.1016/j.jsams.2007.08.011.
-
32.
Paillard T, Noe F, Riviere T, Marion V, Montoya R, Dupui P. Postural performance and strategy in the unipedal stance of soccer players at different levels of competition. J Athl Train. 2006;41(2):172-6. [PubMed ID: 16791302]. [PubMed Central ID: PMC1472651].
-
33.
Matsuda S, Demura S, Nagasawa Y. Static one-legged balance in soccer players during use of a lifted leg. Percept Mot Skills. 2010;111(1):167-77. [PubMed ID: 21058597]. https://doi.org/10.2466/05.23.26.27.PMS.111.4.167-177.
-
34.
Clark CC, Barnes CM, Stratton G, McNarry MA, Mackintosh KA, Summers HD. A Review of Emerging Analytical Techniques for Objective Physical Activity Measurement in Humans. Sports Med. 2017;47(3):439-47. [PubMed ID: 27402456]. https://doi.org/10.1007/s40279-016-0585-y.