Abstract
Background:
The fear of hypoglycemia is the strongest barrier when patients with type 1 diabetes mellitus consider physical activity. There is still a lack of information regarding pre- and post-exercise therapy adaptation strategies with respect to different exercise intensities corresponding to the three phases of lactate metabolism.Objectives:
The aim of this experimental, cross-sectional study was to investigate exercise intensity-dependent, short-acting insulin reductions to avoid hypoglycemia, applying standardized exercises using insulin degludec (®Tresiba/Novo Nordisk, Denmark).Methods:
Seven male participants with type 1 diabetes mellitus were switched to insulin degludec. Intensities for the 30 minutes continuous cycle ergometer exercise tests were set at 5% below (A) and above (B) the lactate turn point 1, and below (C) and above (D) the lactate turn point 2. Reductions in short-acting insulin were applied as following: by 25% for intensity A, by 50% for intensity B and by 75% for intensities C and D four hours before the start of exercise and immediately after exercise. Blood glucose, interstitial glucose, lactate, catecholamines, cortisol, IGF-1 and glucagon were measured.Results:
No hypoglycemic events occurred, but linear blood glucose decreases were observed: 2.01 ± 1.04 mmol.L-1, P = 0.35 (A), 3.00 ± 1.54 mmol.L-1, P = 0.48 (B), 3.42 ± 2.34 mmol.L-1, P = 0.40 (C), and 3.70 ± 3.36 mmol.L-1, P = 0.12 (D). Adrenaline, noradrenaline and IGF-1 (B, C, D, not A), dopamine (C, D, not A and B) and cortisol (A, C, D, not B) increased significantly from baseline, but not glucagon (P > 0.05). Interstitial glucose showed no post-exercise hypoglycemia.Conclusions:
The applied therapy adaptation before and after exercises was adequate to avoid hypoglycemia while using an ultra-long-acting insulin.Keywords
1. Background
In addition to standard insulin therapy, regular exercise has been suggested to lead to positive effects in patients with diabetes by lowering the risk of cardiovascular diseases and early all-cause mortality (1). Furthermore, it was recently shown that adopting a healthy lifestyle in patients with type 1 diabetes mellitus led to an improvement in body fat, body mass index (BMI) and lipid profiles as well as in blood pressure (2). Despite this evidence, the fear of hypoglycemia is the strongest barrier when these patients consider physical activity (3). Exercise-induced hypoglycemia occurs if insulin levels are not adequately lowered before starting to exercise, resulting in an elevated risk of a high glucose uptake rate into the tissue (4). Three possible strategies have been identified to prevent exercise-induced hypoglycemia in patients with type 1 diabetes: first, insulin reduction (short-acting insulin (5-7) or insulin basal rate in pump therapy (8)), second, supplementation with carbohydrates (9) and third, the combination of insulin reduction and carbohydrate ingestion (10). As people with type 1 diabetes mellitus often suffer from weight gain (11), short-acting insulin reduction strategies arise as beneficial for weight management (12). In the last few years, a novel ultra-long-acting insulin has been developed (insulin degludec (IDeg) (®Tresiba/ Novo Nordisk, Bagsvaerd, Denmark)). A recent study has shown that intensive insulin therapy with IDeg is related to less frequent nocturnal hypoglycemic events (13), lower levels and smaller day-to-day variability of fasting plasma glucose (14) while implementing a lower daily insulin dose (15). It also showed a comparable symptomatic and cognitive response to artificially induced hypoglycemia (16) in comparison to insulin glargine (IGlar) (Lantus/ Sanofi-Aventis, France). Only a few studies have investigated physical exercise in patients with type 1 diabetes when treated with IDeg (17, 18). In a recent study (18), no hypoglycemic events were reported during 30 minutes cycle ergometer exercise at 65% peak oxygen uptake with either IDeg or IGlar, but this study only investigated one single exercise intensity. The incidence of late-onset hypoglycemia was similar for both basal insulins indicating a similar risk of hypoglycemia during and after exercise relative to other long-acting insulins. There are currently no recommendations to counteract exercise-induced hypoglycemia when using Ideg. Importantly, most studies investigating the effects of exercise in patients with type 1 diabetes mellitus prescribed exercise intensities as percentages of maximal oxygen uptake (VO2max), maximal heart rate (HRmax) or maximal power output (Pmax) (6, 19, 20), which results in inhomogeneous metabolic and cardiorespiratory responses as shown earlier (21, 22). IDeg has not yet proven to be effective in combination with different short-acting insulin reductions at exercise intensities near to or above the anaerobic threshold. There is a lack of information regarding pre- and post-exercise adaptation strategies for short-acting insulin with respect to different exercise intensities corresponding to the three phases of lactate metabolism. As a hypothesis we expected that standardized therapy adaptations are successful in avoiding hypoglycemia during and after exercise in patients with type 1 diabetes mellitus.
2. Objectives
The main aim of the present study was, therefore, to prove that standardized short-acting insulin dose reductions, dependent on specified exercise intensities, are successful in avoiding hypoglycemia during and after exercise. As a second aim, we assessed the acute metabolic, cardiopulmonary, and hormonal responses at these exercise intensities. A third aim was to extrapolate blood glucose decreases during continuous exercise at different exercise intensities to predict the maximal duration of exercise before hypoglycemia occurs.
3. Methods
3.1. Participants
To be eligible for the study, participants had to be male with type 1 diabetes mellitus for a duration of at least 12 months, aged 18 to 35 years, HbA1c below 8 % (64 mmol.mol-1), fasting c-peptide below 0.3 nmol.L-1, treated with intensive insulin therapy, no disease-related long-term complications and no other physical and/or mental diseases. Just male participants were recruited since the female menstrual cycle influences glucose metabolism, and could interfere with the long investigation period. Participants were excluded if they had a history of any disease that could confound the results of the trial, use of drugs (which may interfere with the interpretation of the trial’s results or is known to be clinically relevant in interfering with insulin action), recovery from hypoglycemia, current alcohol addiction, controlled substance abuse (determined by investigator questioning, documented in the case report form (CRF)), known or suspected allergy to trial products or related products, language barriers precluding adequate understanding, unwillingness, mental incapacity, and any condition that the study physician feels would interfere with the trial participation. Testing day inclusion criteria were absence of hypoglycemia 48 hours prior to testing, documented by the participant by self-measured blood sugar and additionally controlled by the observation of the continuous glucose monitoring system (CGM) (®Guardian, REAL-Time System, Medtronic, Fridley, Minnesota, USA) by the study physician, as well as no alcohol consumption 24 hours before testing determined by questioning (documented in the CRF). Testing day exclusion criteria were defined as: illness, glucose levels before testing below 4.4 mmol.L-1, CGM problems, incorrect time or amount of last short-acting insulin injection before testing. All inclusion and exclusion criteria were reported in a CRF. Hypoglycemia was set at ≤ 3.9. mmol.L-1.
3.2. Measures
3.2.1. Preliminary Adjustment of Long-Acting Insulin Therapy
The usual long-acting insulin dose per day was determined by interviewing at the first visit four weeks prior to the study. IDeg (®Tresiba/Novo Nordisk, Bagsvaerd, Denmark) was subcutaneously injected once daily in the evening, starting with a dose of 70% of total daily long-acting insulin (16). In cases of hypo- or hyperglycemia, the insulin dose was adjusted. A run-in period of one month ensured an optimal long-acting insulin therapy.
3.3. Incremental Exercise Test
All participants performed an incremental cycle ergometer exercise test until exhaustion to determine exercise intensities for the subsequent continuous cycle ergometer exercise tests (21). At the beginning of the incremental exercise test, participants passively sat on the ergometer (3 min - 0 W). Afterwards participants warmed-up cycling for 3 minutes (40 W). Subsequently, the intensity was increased every minute (20 W) until maximal exhaustion, followed by 3 minutes active recovery (40 W) and 3 minutes passive recovery (0 W). The first (LTP1) and the second lactate turn point (LTP2) were identified by a linear regression break point analysis (relationship of lactate concentration to power output). According to the three-phases of energy supply, LTP1 and LTP2 were determined as the intersection point between two regression lines. The individual test results were used to define the intensities for the continuous exercise tests for each patient. These workload intensities were set at 5% of Pmax below and above LTP1 and LTP2 do define four metabolically different individual exercise intensities. Exercise intensity below LTP1 (A) conformed to regular physical activity as well as occupational work for several hours, and exercise above LTP1 (B) conformed to moderate walking or low-intensity running. The intensity just below LTP2 (C) matched to high-intensity continuous running or cycling in a metabolic steady state but for a limited duration, and the intensity above LTP2 (D) corresponded to clearly limited exercise duration without a lactate steady state and with early termination due to exhaustion.
3.4. Continuous Cycle Ergometer Exercise Tests
Each patient performed four 30 minutes continuous cycle ergometer exercise tests (A, B, C, D). The four tests were separated by one week for recovery. Exercise intensities were not randomized in order to minimize potential risks, as the defined exercise intensities and short-acting insulin reductions were used for the first time in that experimental setting. Since only one exercise test of 30 minutes was performed within one week, no “training effects” were suggested, as no intra-individual differences were observed during passive and active warm-up periods in metabolic and cardiorespiratory responses. Tests begun identically to the incremental exercise tests (passive and active warm-up) followed by a stepwise increase of exercise intensity (20 W.min-1) until the final target workload for each subject was reached and maintained for 30 minutes. Finally, the cool-down period was similar to the incremental exercise test (3 minutes active recovery at 40 W and 3 minutes passive recovery at 0 W). The warm-up, the incremental approach to the target workload and the recovery phase were performed in order to control for day-to-day variability in physiological responses.
3.5. Analyses
Blood lactate and blood glucose concentrations were determined by taking capillary blood samples (ear lobe) during all tests at rest, warm-up, during exercise and recovery; blood samples were analyzed by means of a enzymatic-amperometric method (®Biosen S-line, EKF diagnostics, Germany). Breath-by-breath data were collected continuously during all tests, and 5 s average data were used for analyses (ZAN 600, ZAN, Germany). Heart rate was measured beat-to-beat during all tests, and 5 s average data were used for calculations (PE 4000, Polar Electro, Finland). A 12-lead electrocardiogram and blood pressure measurements (every 2 minutes) were used for cardiac monitoring. Venous samples of catecholamines, cortisol, insulin-like growth factor-1 (IGF-1), and glucagon were determined from a cubital vein before the start of the exercise, at 15 minutes during exercise, and at the end of each continuous 30 minutes exercise test (A - D). Adrenaline (limit of detection 10 pg.mL-1), noradrenaline (limit of detection 50 pg.mlL-1), and dopamine (limit of detection 20 pg.mL-1) were quantified by ®RIA (DRG Diagnostics, USA). Glucagon (limit of detection 20 pg.ml-1) was determined by ®RIA (ICN, USA), and cortisol (detection of 0.20 - 75 µg.dL-1) and IGF-1 (limit of detection 10.1 pg.mL-1) by ®CLIA (CENTAUR, siemens healthcare diagnostics, USA). C-peptide was measured by ®Advia Centaur (CENTAUR, siemens healthcare diagnostics, USA) (detection of 0.05 - 30 ng.mL-1).
3.6. Continuous Glucose Monitoring
Patients were fitted with a CGM and provided with all necessary instructions. The CGM sensor was inserted into the posterolateral abdominal region a minimum of 24 hours pre-exercise to measure blood glucose alongside self-measurement to avoid hypoglycemic events. The CGM was left inserted for another 24 hours post-exercise to observe glucose activity and the effect of exercise intensity (A, B, C, D). CGM alerts were set at below 4.4 mmol.L-1 and above 16.0 mmol.L-1 during the two 24 hours periods. The study physician asked about previous hypoglycemic events before the exercise tests, and any relevant information was documented in a CRF.
3.6.1. Short-Acting Insulin Reduction
After an overnight fast, each participant received a standardized meal (last meal before exercise testing) on test days (Fortimel Extra, Nutricia GmbH, Germany). The carbohydrate amount of 59 ± 8 g before to the tests was calculated on the mean amount at breakfast over the last four weeks prior the study. Standardized nutrition was composed of 39% carbohydrates, 36% proteins and 25% fat (Fortimel Extra, Nutricia GmbH, Germany). This standardized meal and the before mentioned short-acting insulin injections were administered four hours before the start of the exercise tests. This long time span was mainly applied to see the exercise-induced blood glucose decrease by minimizing the influence of short-acting insulin action during cycling. The short-acting insulin dose was reduced by 40% for the incremental exercise test, by 25% for exercise A, by 50% for exercise B and by 75% for both exercise intensities C and D, modified from Rabasa-Lhoret et al. (6). Post-exercise, patients injected the same reduced short-acting insulin dose and ingested the same amount of carbohydrate as before testing to simulate post-exercise eating habits. For the rest of the day, patients were requested to continue with their regular insulin dose according to the formula (carbohydrate factor = (5.7 * bodyweight (kg)) / total daily insulin dose-1).
3.7. Procedures
All participants gave a signed informed consent prior the trial started. The trial was approval by the local ethics committee, according to good clinical practice (GCP) and declaration of Helsinki (DoH) as well as approved by Clincicaltrials.gov. The study consisted of 20 visits at the outpatient clinic of Endocrinology and Metabolism, and at the exercise physiology laboratory. The outpatient clinic was visited for the adjustment to IDeg (visits 1 - 10, with three days in between) and to equip participants with CGM (visits 12, 14, 16, and 18, with one week in between). The exercise physiology laboratory was visited for the incremental exercise test (visit 11) and for the continuous cycle ergometer exercise tests (visits 13, 15, 17, and 19, with one week in between).
3.8. Statistical Analysis
A repeated-measures ANOVA design was calculated with an effect size of 0.5 and an α-error of 0.05 based on a pilot study with a main outcome defined as the expected blood glucose decrease. With a sample size of six patients with type 1 diabetes mellitus the power (β-1) is greater than 0.95. To control for dropout, we conducted our study with seven participants. Data were normally distributed except CGM levels, analyzed via Shapiro-Wilk normality test. Participants’ anthropometric data, performance characteristics and diabetes specific data are given as mean and range. Means of blood glucose decrease and hormone responses during exercise were calculated by repeated measures ANOVA and Tukey’s multiple comparison post-hoc test. Blood glucose decrease was extrapolated from the end of the test until reaching a hypoglycemic threshold of 3.9 mmol.L-1. Relationships between blood glucose decreases and carbohydrate oxidation were performed by Pearson’s correlation coefficient analysis. CGM data are given as median and range (upper limit - lower limit), and coefficient of variation (CV) analysis from median was applied. For analysis of time spent in hyper- or hypoglycemia with the CGM we used the median and range for the values above 10.0 mmol.L-1 or below 3.9 mmol.L-1. All statistics were calculated with prism Software version 4.0 (GraphPad, USA).
4. Results
Seven men with type 1 diabetes mellitus were enrolled in this study (Table 1). All participants were switched to ultra-long-acting IDeg with an average dose of 68 ± 13% of their usual pre-study long-acting insulin dose, for daily conditions. All data were normally distributed.
Variable | Characteristic | Characteristic | Characteristic |
---|---|---|---|
Participants’ characteristics | |||
Age, y | Height, m | Body weight, kg | BMI, kg/m2 |
24.7 (20 - 34) | 1.76 (171 - 181) | 74 (66 - 81) | 23.9 (20 - 27) |
Performance characteristics | |||
VO2max, ml.kg-1.min-1 | PLTP1, W | PLTP2, W | Pmax, W |
52 (41 - 67) | 82.1 (51 - 111) | 192.1 (151 - 253) | 284.3 (230 - 360) |
Diabetes specific data | |||
Hba1c, No.%, mmol.mol-1 | c-peptide, nmol.L-1 | Diabetes, y | CHO factor, g |
7.4 (57) ((6.5 - 8) (47 - 64)) | 0.13 (0 - 0.3) | 16.9 (4 - 26) | 12 (9 - 16) |
Average oxygen consumption for each exercise intensity was 36 ± 10% VO2max (A), 40 ± 13% VO2max (B), 78 ± 11% VO2max (C), and 84 ± 15% VO2max (D).
Blood lactate concentration profiles corresponded well to the expected patterns with resting lactate levels in A, slightly but significantly elevated steady state lactate levels in B, significantly elevated but still steady state lactate levels in C, but no steady state and a continuous increase of lactate with an early termination of exercise in D (Figure 1A).
A: Lactate Concentrations During the 4 Continuous Exercise Tests (A, B, C, and D) Given as Mean ± SD. Solid Line for Exercise Intensity A, Broken Line for B, Dotted Line for C, and Dash-Dot Line For D. Differences in Lactate at Min Zero (Target Workload) Occur Due to Different Time Spans in the Warm-Up Period. B: Average Carbohydrate Oxidation Over Time (CHO) (G.Min-1) for the 4 Exercise Intensities (A, B, C, And D) Given as Mean ± SD. Significant Differences Were Found for All Tests (P < 0.01) Except a Vs. B (P = 0.36)
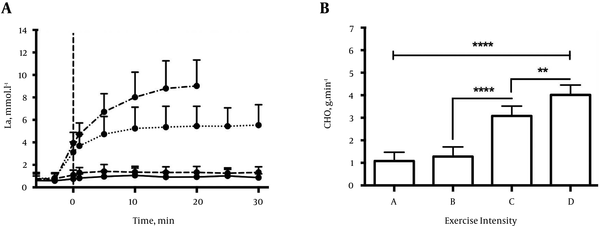
The average short-acting insulin dose four hours before the exercise on each test day was 0.041 ± 0.014 U.kg-1 for the incremental exercise test, 0.054 ± 0.014 U.kg-1 for continuous cycle ergometer exercise A, 0.027 ± 0.014 U.kg-1 for B, and 0.014 ± 0.007 U.kg-1 for C and D, respectively. Those amounts were combined with a carbohydrate meal of 0.797 ± 0.069 g.kg-1 (Fortimel Extra, Nutricia GmbH, Germany), which was the average amount ingested at breakfasts 4 weeks prior to the study start.
4.1. Blood Glucose Levels and Carbohydrate Oxidation
No participant experienced hypoglycemia (blood glucose ≤ 3.9 mmol.l-1), neither during exercise nor within 24 hours after any of the 35 exercise sessions. All participants maintained a glucose steady state during the incremental exercise test when short-acting insulin was reduced by 40%, with no difference between the start and the end of the test (10.58 ± 3.42 mmol.L-1 vs. (10.24 ± 3.48 mmol.L-1, P = 0.85). Starting blood glucose for the continuous cycle ergometer exercise tests were found at 10.42 ± 2.01 mmol.L-1 (range: 6.19 - 12.31 mmol.L-1) for exercise intensity A, 12.78 ± 3.76 mmol.L-1 (range: 7.44 - 16.01 mmol.L-1) (B), 12.84 ± 3.21 mmol.L-1 (range: 6.15 - 16.40 mmol.L-1) (C), and 14.98 ± 2.43 mmol.L-1 (range: 9.72 - 16.16 mmol.L-1) (D) (Figure 2). Blood glucose linearly decreased in all continuous exercise sessions with delta values of 2.01 ± 1.04 mmol.L-1, P = 0.35 (A), 3.00 ± 1.54 mmol.l-1, P = 0.48 (B) 3.42 ± 2.34 mmol.l-1, P = 0.40 (C), and 3.70 ± 3.36 mmol.L-1, P = 0.12 (D), respectively. Delta blood glucose was not significantly different (P = 0.64) and not significantly correlated with carbohydrate oxidation (r = 0.31; P = 0.34). Carbohydrate oxidation, which was calculated from respiratory data, was 1.08 ± 0.39 g.min-1 for A, 1.28 ± 0.42 g.min-1 for B, 3.07 ± 0.44 g.min-1 for C, and 4.02 ± 0.44 g.min-1 for D (Figure 1B). A linear extrapolation of blood glucose decrease to the hypoglycemic threshold of 3.9 mmol.L-1 was significantly different only between exercise intensity A and C (P = 0.03). The durations to the hypoglycemic threshold were found at 120 ± 56 minutes (A), 92 ± 47 minutes (B), 80 ± 62 minutes (C) and 59 ± 52 minutes (D) (Figure 2).
Exercise-Intensity Dependent Linear Blood Glucose Decrease During Continuous Exercise Tests (A Represents Exercise Intensity A, B Represents Exercise Intensity B, C Represents Exercise Intensity C and D Represents Exercise Intensity D) Given as Mean ± SD (Dotted Line: Critical Time). For Test D, the Exercise Intensity-Induced Strain Resulted in an Early Stop At 21 ± 6 minutes. The Time Point Where the Extrapolation of Blood Glucose Crosses the X-Axis Indicates the Hypoglycemic Threshold of 3.9 mmol.L-1 and the Time Point Where the Extrapolation Of Blood Glucose Crosses the Horizontal Dotted Line Indicates 50% of Baseline Value
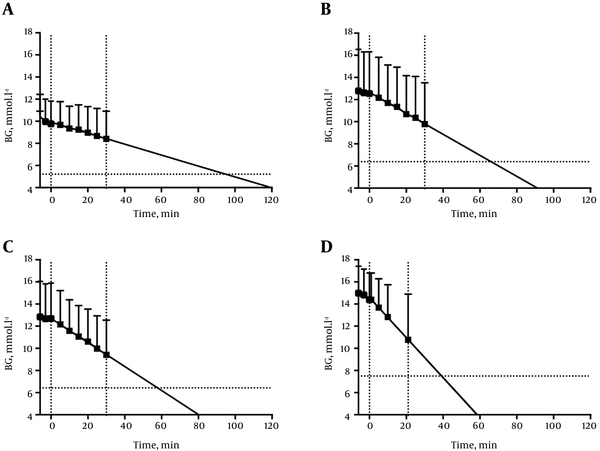
4.2. Late Glycemic Onset Measured by CGM
Median (range) glucose levels during 24 hours post exercise were 8.52 (4.99 - 12.30) mmol.L-1 with a coefficient of variation (CV) of 16.49% (A), 9.86 (5.96 - 15.67) mmol.L-1, CV 18.04% (B), 8.98 (5.91 - 13.57) mmol.L-1, CV 24.90% (C), and 8.48 (5.57 - 13.36) mmol.L-1, CV 28.15% (D). No hypoglycemia (≤ 3.9 mmol.L-1) was found for all exercise intensities during the 24 hours post-exercise period. Hyperglycemic conditions (> 10.0 mmol.L-1) were observed in A for 280 minutes with an average of 10.6 (10 - 12.1) mmol.L-1, in B for 660 minutes with 10.9 (10 - 14.5) mmol.L-1, in C for 415 minutes with 11.5 (10 - 14.7) mmol.L-1, and in D for 340 minutes with 11.8 (10 - 15.9) mmol.L-1 (Figure 3).
Median Line of Interstitial Glucose After Exercise Intensity A, B, C, And D. Solid Line for Exercise Intensity A, Broken Line for B, Dotted Line for C, and Dash-Dot Line for D. Horizontal Dotted Lines Set For Hypo- (Lower Line) and Hyperglycaemia (Upper Line)
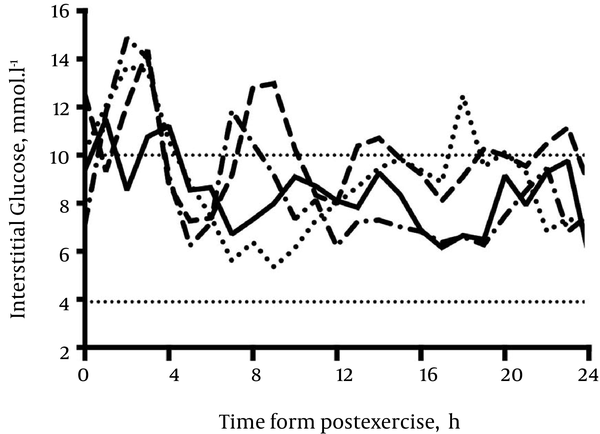
4.3. Hormone Levels
Adrenaline (C and D, P < 0.01), noradrenaline (B, C and D, P < 0.01), dopamine (C and D, P < 0.01), cortisol (A, P = 0.03; C, P = 0.01 and D, P = 0.03), and IGF-1 (B, P = 0.04; C and D, P < 0.01) but not glucagon (P > 0.05), significantly increased during constant load exercise (Figure 4). The time course of adrenaline, noradrenaline, and cortisol was similar to the pattern of blood lactate concentrations. This clearly displayed the expected three phase time course for these variables with no increase for intensities A and B, but a significant increase in C, and a marked elevation and no steady state in D.
Comparison of Adrenaline (A), Noradrenaline (B), Dopamine (C), Cortisol (D), Glucagon, (E) and IGF-1 (F) With Respect to the Four Different Exercise Intensities, Given as Mean ± SD. Solid Line For Exercise Intensity A, Broken Line For B, Dotted Line for C, and Dash-Dot Line For D
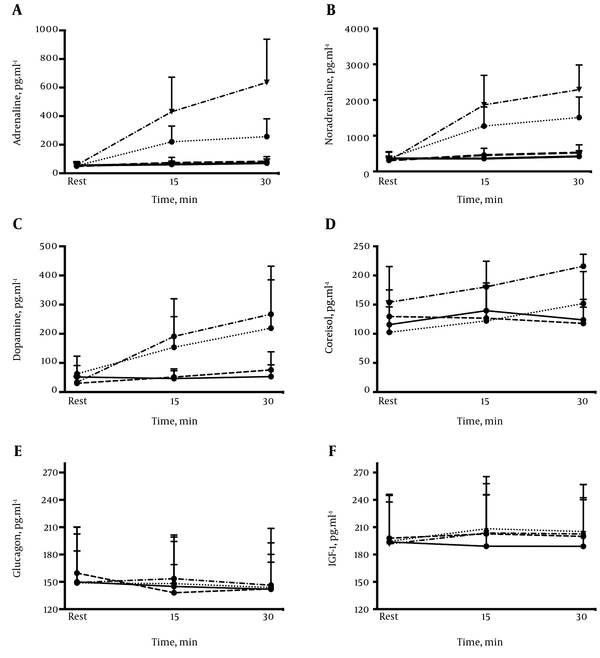
5. Discussion
Our study included low and high exercise intensities at 5% of Pmax below and above both lactate turn points. Even for high intensity exercise (C and D), the applied short-acting insulin reductions in combination with IDeg were found to be safe. This is highly relevant since many patients with type 1 diabetes mellitus are performing high-intensity competitive sports (23). In comparison to the findings of Heise and colleagues (18), where exercise intensity was set at 65 % of VO2max with the same duration as in our study (30 minutes), the observed blood glucose decrease was higher in our study. Due to different exercise prescription methods and differences in exercise intensities, a comparison of these results is difficult. Our study showed a dose-response relationship of exercise intensity and the decrease of blood glucose concentration during continuous cycle ergometer exercises independent of insulin reduction. The blood glucose decrease was linear with time and was dependent on exercise intensity, which allows to estimate critical time limits (time to reach the hypoglycemic threshold of 3.9 mmol.L-1) as well as to prospectively calculate the risk of hypoglycemia. The linear extrapolation of the blood glucose decrease during exercises A, B, C, and D showed an intensity-related range of duration between 120 ± 56 minutes (A) and 59 ± 52 minutes (D) to reach the hypoglycemic threshold. A prospective calculation of the expected blood glucose decrease might be a simple method which could be used easily by the patients themselves, and which could help patients learn when and how to supplement carbohydrates with respect to exercise intensity and duration. For conclusive recommendations, however, additional large-scale studies are required specifically on the influence of the pre-exercise glucose concentration. Since we started at high blood glucose levels in our tests, it has to be critically mentioned that these conditions (although usual in daily practice) (23) might influence the glucose consumption rate and the glucose decrease with time as shown by Jenny and colleagues (24). These authors (24) demonstrated that in hyperglycemic conditions, metabolism was dominated by carbohydrate oxidation. Furthermore, hyperinsulinemic conditions were shown to directly increase exogenous glucose utilization (25). Based on these studies, we assume that the hyperglycemic pre-exercise blood glucose levels in our study subsequently influenced the blood glucose decreases during exercise. On the other hand, it was shown by Stettler and colleagues (26) that hyperglycemia did not affect exercise capacity, and no significant differences were found in cardiorespiratory and metabolic responses when hyper- and euglycemic conditions were compared. Previous self-reported glucose levels before an exercise in athletes with type 1 diabetes mellitus (23), were similar to our pre-exercise values. These results indicate that high blood glucose levels illustrate real-life situations in patients with type 1 diabetes mellitus performing regular exercise. On the other hand, the starting blood glucose level at exercise intensity D was non-physiologically high, but was suggested necessary to avoid hypoglycemia during and after high-intensity anaerobic exercise without any supplementation of carbohydrates during the test. As the accelerated lactate accumulation and acidosis of this exercise test clearly limits the duration, there is no need to adapt to artificially high starting glucose concentrations even if a high-intensity interval method is applied (17). While most of our post-exercise values for time spent in hyperglycemia (> 10.0 mmol.L-1) were comparable to a recent study with similar conditions (7), we identified a long-time period of 660 minutes of hyperglycemia at exercise intensity B. This might be explained by an inadequate and exaggerated short-acting insulin reduction after exercise. The 50 % reduction of insulin seems to be too high for people with type 1 diabetes mellitus for this type of exercise. Unfortunately, due to the low number of available studies, recommendations for individual insulin reductions for exercise are usually too generalized for patients with type 1 diabetes mellitus. One main outcome of our study was to show that the individual turn points of lactate (LTP1, LTP2) from an incremental exercise test, allow to clearly describe three different exercise domains indicated by a distinct difference of blood lactate and catecholamine responses. This is in contrast to most studies, which used exercise intensity prescriptions by means of percentages of maximal values (e.g. VO2max or maximal heart rate). It was shown by Scharhag-Rosenberger and colleagues (22) that this kind of exercise intensity prescription for endurance training and study purposes gave a non-acceptable range of metabolic responses that may be individually inadequate and inhomogeneous within a group of subjects. Therefore, these authors (22) as well as others (21, 27) recommended using exercise intensity prescriptions based on thresholds (turn points) but not on % of VO2max or HRmax. Applying the individual lactate turn point method to determine individual exercise intensities resulted in the expected exercise intensity-dependent response pattern for lactate, adrenaline, noradrenaline, cortisol, IGF-1, and dopamine. A lack of a glucagon response in patients with a long duration of type 1 diabetes, has been shown to be tightly linked to endogenous insulin deficiency (28), specific beta-cell and neural factors. One shortcoming of the study was that we did not measure insulin levels and just controlled the injected insulin doses and c-peptide levels. Therefore, we could not directly analyze the effect of insulin on the decrease of blood glucose. The fairly high standard deviation of the blood glucose decrease and the difference in starting blood glucose levels, despite the same short-acting insulin reduction (for exercise intensities C and D), could be considered normal when analyzing the patients’ intra-variability in insulin action (29, 30). Our study was also limited by the low number of subjects and the short duration of exercise bouts (30 minutes). A longer duration may be necessary for lower exercise intensities (A, B) to better validate the extrapolation model. As we just used the recommended intensity-dependent insulin reduction strategy with standardized exercise conditions, further studies are necessary to investigate different reduction strategies for short-acting insulin related to our theory-based exercise prescription model, including a greater number of subjects. Despite these limitations, our study was able to show that individual recommendations for the reduction of short-term insulin are possible and safe if applied to individually defined exercise intensities. The method of our study allows to identify the individual exercise intensity-dependent, maximal duration, before reaching the hypoglycaemia threshold during a constant load exercise with a given insulin reduction strategy, instead of an overall recommendation.
References
-
1.
Kodama S, Tanaka S, Heianza Y, Fujihara K, Horikawa C, Shimano H, et al. Association between physical activity and risk of all-cause mortality and cardiovascular disease in patients with diabetes: a meta-analysis. Diabetes Care. 2013;36(2):471-9. [PubMed ID: 23349151]. https://doi.org/10.2337/dc12-0783.
-
2.
Leroux C, Gingras V, Desjardins K, Brazeau AS, Ott-Braschi S, Strychar I, et al. In adult patients with type 1 diabetes healthy lifestyle associates with a better cardiometabolic profile. Nutr Metab Cardiovasc Dis. 2015;25(5):444-51. [PubMed ID: 25770760]. https://doi.org/10.1016/j.numecd.2015.01.004.
-
3.
Brazeau AS, Rabasa-Lhoret R, Strychar I, Mircescu H. Barriers to physical activity among patients with type 1 diabetes. Diabetes Care. 2008;31(11):2108-9. [PubMed ID: 18689694]. https://doi.org/10.2337/dc08-0720.
-
4.
Galassetti P, Riddell MC. Exercise and Type 1 Diabetes (T1DM). Compr Physiol. 2013:1309-36. https://doi.org/10.1002/cphy.c110040.
-
5.
West DJ, Morton RD, Bain SC, Stephens JW, Bracken RM. Blood glucose responses to reductions in pre-exercise rapid-acting insulin for 24 h after running in individuals with type 1 diabetes. J Sports Sci. 2010;28(7):781-8. [PubMed ID: 20496226]. https://doi.org/10.1080/02640411003734093.
-
6.
Rabasa-Lhoret R, Bourque J, Ducros F, Chiasson JL. Guidelines for premeal insulin dose reduction for postprandial exercise of different intensities and durations in type 1 diabetic subjects treated intensively with a basal-bolus insulin regimen (ultralente-lispro). Diabetes Care. 2001;24(4):625-30. [PubMed ID: 11315820].
-
7.
Campbell MD, Walker M, Bracken RM, Turner D, Stevenson EJ, Gonzalez JT, et al. Insulin therapy and dietary adjustments to normalize glycemia and prevent nocturnal hypoglycemia after evening exercise in type 1 diabetes: a randomized controlled trial. BMJ Open Diabetes Res Care. 2015;3(1):85. [PubMed ID: 26019878]. https://doi.org/10.1136/bmjdrc-2015-000085.
-
8.
Franc S, Daoudi A, Pochat A, Petit MH, Randazzo C, Petit C, et al. Insulin-based strategies to prevent hypoglycaemia during and after exercise in adult patients with type 1 diabetes on pump therapy: the DIABRASPORT randomized study. Diabetes Obes Metab. 2015;17(12):1150-7. [PubMed ID: 26264812]. https://doi.org/10.1111/dom.12552.
-
9.
Francescato MP, Geat M, Accardo A, Blokar M, Cattin L, Noacco C. Exercise and glycemic imbalances: a situation-specific estimate of glucose supplement. Med Sci Sports Exerc. 2011;43(1):2-11. [PubMed ID: 20473219]. https://doi.org/10.1249/MSS.0b013e3181e6d6a1.
-
10.
West DJ, Stephens JW, Bain SC, Kilduff LP, Luzio S, Still R, et al. A combined insulin reduction and carbohydrate feeding strategy 30 min before running best preserves blood glucose concentration after exercise through improved fuel oxidation in type 1 diabetes mellitus. J Sports Sci. 2011;29(3):279-89. [PubMed ID: 21154013]. https://doi.org/10.1080/02640414.2010.531753.
-
11.
Conway B, Miller RG, Costacou T, Fried L, Kelsey S, Evans RW, et al. Temporal patterns in overweight and obesity in Type 1 diabetes. Diabet Med. 2010;27(4):398-404. [PubMed ID: 20536510]. https://doi.org/10.1111/j.1464-5491.2010.02956.x.
-
12.
Gallen IW, Hume C, Lumb A. Fuelling the athlete with type 1 diabetes. Diabetes Obes Metab. 2011;13(2):130-6. [PubMed ID: 21199264]. https://doi.org/10.1111/j.1463-1326.2010.01319.x.
-
13.
Dzygalo K, Golicki D, Kowalska A, Szypowska A. The beneficial effect of insulin degludec on nocturnal hypoglycaemia and insulin dose in type 1 diabetic patients: a systematic review and meta-analysis of randomised trials. Acta Diabetol. 2015;52(2):231-8. [PubMed ID: 25430705]. https://doi.org/10.1007/s00592-014-0604-0.
-
14.
Yamamoto C, Miyoshi H, Fujiwara Y, Kameda R, Ichiyama M, Nomoto H, et al. Degludec is superior to glargine in terms of daily glycemic variability in people with type 1 diabetes mellitus. Endocr J. 2016;63(1):53-60. [PubMed ID: 26522272]. https://doi.org/10.1507/endocrj.EJ15-0438.
-
15.
Nakamura T, Sakaguchi K, So A, Nakajima S, Takabe M, Komada H, et al. Effects of insulin degludec and insulin glargine on day-to-day fasting plasma glucose variability in individuals with type 1 diabetes: a multicentre, randomised, crossover study. Diabetologia. 2015;58(9):2013-9. [PubMed ID: 26044206]. https://doi.org/10.1007/s00125-015-3648-y.
-
16.
Koehler G, Heller S, Korsatko S, Roepstorff C, Rasmussen S, Haahr H, et al. Insulin degludec is not associated with a delayed or diminished response to hypoglycaemia compared with insulin glargine in type 1 diabetes: a double-blind randomised crossover study. Diabetologia. 2014;57(1):40-9. [PubMed ID: 24057153]. https://doi.org/10.1007/s00125-013-3056-0.
-
17.
Moser O, Tschakert G, Mueller A, Groeschl W, Pieber TR, Obermayer-Pietsch B, et al. Effects of High-Intensity Interval Exercise versus Moderate Continuous Exercise on Glucose Homeostasis and Hormone Response in Patients with Type 1 Diabetes Mellitus Using Novel Ultra-Long-Acting Insulin. PLoS One. 2015;10(8):e0136489. [PubMed ID: 26317981]. https://doi.org/10.1371/journal.pone.0136489.
-
18.
Heise T, Bain SC, Bracken RM, Zijlstra E, Nosek L, Stender-Petersen K, et al. Similar risk of exercise-related hypoglycaemia for insulin degludec to that for insulin glargine in patients with type 1 diabetes: a randomized cross-over trial. Diabetes Obes Metab. 2016;18(2):196-9. [PubMed ID: 26450456]. https://doi.org/10.1111/dom.12588.
-
19.
Bracken RM, West DJ, Stephens JW, Kilduff LP, Luzio S, Bain SC. Impact of pre-exercise rapid-acting insulin reductions on ketogenesis following running in Type 1 diabetes. Diabet Med. 2011;28(2):218-22. [PubMed ID: 21219433]. https://doi.org/10.1111/j.1464-5491.2010.03162.x.
-
20.
Campbell MD, Walker M, Trenell MI, Jakovljevic DG, Stevenson EJ, Bracken RM, et al. Large pre- and postexercise rapid-acting insulin reductions preserve glycemia and prevent early- but not late-onset hypoglycemia in patients with type 1 diabetes. Diabetes Care. 2013;36(8):2217-24. [PubMed ID: 23514728]. https://doi.org/10.2337/dc12-2467.
-
21.
Hofmann P, Tschakert G. Special needs to prescribe exercise intensity for scientific studies. Cardiol Res Pract. 2010;2011:209302. [PubMed ID: 21197479]. https://doi.org/10.4061/2011/209302.
-
22.
Scharhag-Rosenberger F, Meyer T, Gassler N, Faude O, Kindermann W. Exercise at given percentages of VO2max: heterogeneous metabolic responses between individuals. J Sci Med Sport. 2010;13(1):74-9. [PubMed ID: 19230766]. https://doi.org/10.1016/j.jsams.2008.12.626.
-
23.
Ratjen I, Weber KS, Roden M, Herrmann ME, Mussig K. Type 1 diabetes mellitus and exercise in competitive athletes. Exp Clin Endocrinol Diabetes. 2015;123(7):419-22. [PubMed ID: 25853705]. https://doi.org/10.1055/s-0035-1545344.
-
24.
Jenni S, Oetliker C, Allemann S, Ith M, Tappy L, Wuerth S, et al. Fuel metabolism during exercise in euglycaemia and hyperglycaemia in patients with type 1 diabetes mellitus--a prospective single-blinded randomised crossover trial. Diabetologia. 2008;51(8):1457-65. [PubMed ID: 18512043]. https://doi.org/10.1007/s00125-008-1045-5.
-
25.
Chokkalingam K, Tsintzas K, Norton L, Jewell K, Macdonald IA, Mansell PI. Exercise under hyperinsulinaemic conditions increases whole-body glucose disposal without affecting muscle glycogen utilisation in type 1 diabetes. Diabetologia. 2007;50(2):414-21. [PubMed ID: 17119916]. https://doi.org/10.1007/s00125-006-0520-0.
-
26.
Stettler C, Jenni S, Allemann S, Steiner R, Hoppeler H, Trepp R, et al. Exercise capacity in subjects with type 1 diabetes mellitus in eu- and hyperglycaemia. Diabetes Metab Res Rev. 2006;22(4):300-6. [PubMed ID: 16302286]. https://doi.org/10.1002/dmrr.608.
-
27.
Tschakert G, Kroepfl J, Mueller A, Moser O, Groeschl W, Hofmann P. How to regulate the acute physiological response to "aerobic" high-intensity interval exercise. J Sports Sci Med. 2015;14(1):29-36. [PubMed ID: 25729286].
-
28.
Cryer PE. Hypoglycaemia: the limiting factor in the glycaemic management of Type I and Type II diabetes. Diabetologia. 2002;45(7):937-48. [PubMed ID: 12136392]. https://doi.org/10.1007/s00125-002-0822-9.
-
29.
Heise T, Hermanski L, Nosek L, Feldman A, Rasmussen S, Haahr H. Insulin degludec: four times lower pharmacodynamic variability than insulin glargine under steady-state conditions in type 1 diabetes. Diabetes Obes Metab. 2012;14(9):859-64. [PubMed ID: 22594461]. https://doi.org/10.1111/j.1463-1326.2012.01627.x.
-
30.
Hermansen K, Fontaine P, Kukolja KK, Peterkova V, Leth G, Gall MA. Insulin analogues (insulin detemir and insulin aspart) versus traditional human insulins (NPH insulin and regular human insulin) in basal-bolus therapy for patients with type 1 diabetes. Diabetologia. 2004;47(4):622-9. [PubMed ID: 15298338]. https://doi.org/10.1007/s00125-004-1365-z.