Abstract
Background:
Omega-3 fatty acid supplementation has been shown to help maintain muscle function and reduce muscle soreness after exercise-induced muscle damage. However, the relationship between the dietary and biological status of omega-3 fatty acids in rugby players remains unclear.Objectives:
This study aimed to investigate the connection between the dietary and biological status of omega-3 fatty acids in university rugby players.Methods:
We conducted a cross-sectional study involving university rugby players and age-matched sedentary controls. We assessed diets, including omega-3 and omega-6 polyunsaturated fatty acids, using self-administered dietary questionnaires. Whole blood lipidomics was performed before and after a single training session.Results:
The rugby group (n = 29) had significantly higher intakes of omega-3 and omega-6 fatty acids compared to the control group (n = 31). While the blood omega-6 relative concentration did not differ between the groups, the rugby group had lower omega-3 fatty acid levels than the control group (4.4 ± 1.1 vs. 6.2 ± 1.8%). Approximately 48% of rugby players had an omega-3 index (O3i) considered as high risk for cardiovascular disease, and 52% were classified as intermediate risk. None of the players had a low-risk O3i. A single training session had an impact on the omega-3 and omega-6 metabolic pathways.Conclusions:
Despite having a higher omega-3 fatty acid intake than controls, rugby football players may still be deficient in omega-3 fatty acids. Further nutritional interventions are needed to address these issues.Keywords
1. Background
The nutritional status, including omega-3 polyunsaturated fatty acids (n-3 PUFAs) status, significantly impacts skeletal muscle health and metabolism (1). n-3 PUFAs play a crucial role in various cellular functions, such as structural maintenance, cell membrane fluidity, and signaling. Additionally, n-3 PUFAs regulate inflammatory processes, blood clotting, blood pressure, glucose tolerance, and the nervous system (2).
Furthermore, scientific research suggests that supplementing with n-3 PUFAs can help maintain muscle function and reduce muscle soreness following exercise-induced muscle damage (3). The omega-3 index (O3i), which comprises docosahexaenoic acid (DHA, 22:6 n-3) and eicosapentaenoic acid (EPA, 20:5 n-3) levels, serves as an indicator of coronary heart disease mortality (4). Among American football athletes, the O3i was categorized as follows: high risk (34%), intermediate (66%), and low risk (0%) (5). However, the relationship between the dietary and biological status of omega-3 fatty acids in Japanese rugby players remains unknown. Furthermore, no studies on changes in lipidomics after training have been published to date.
Nutritional interventions incorporating omega-3 fatty acids offer potential benefits in terms of performance enhancement, facilitating recovery, and reducing the risk of sports-related injuries (6). A meta-analysis conducted by Yeomans et al. revealed that the injury rate among amateur rugby union players was lower than that among professional players but higher than the rates reported in adolescent and youth rugby players (7). Therefore, it becomes crucial to assess omega-3 intake and evaluate the omega-3 status in the bodies of university-level rugby players.
2. Objectives
The study aimed to investigate the correlation between the dietary and biological status of omega-3 fatty acids in university rugby players.
3. Methods
3.1. Research Design
This case-control study involving university rugby players and controls took place between March and May 2019 in Japan.
3.2. Population
We selected rugby players from a single university team. The inclusion criteria for the case group were as follows: participants had to be university rugby football players competing at the highest level of the local league (A-League) in Japan. Additionally, we recruited 31 control subjects from the same university through poster advertisements. The inclusion criteria for the control group were as follows: they were not engaged in regular exercise (8). Exclusion criteria for both groups were as follows: they did not consent to the study protocol. To ensure the absence of selection bias, we intentionally recruited participants from the same university.
3.3. Measures
Body mass index (BMI) was calculated as the ratio of one's weight in kilograms (kg) to the square of their height in meters (m2). Body composition, including body fat and lean body mass, was evaluated using bioimpedance analysis with the InBody 270 device, manufactured by InBody Japan Inc, located in Tokyo, Japan. Dietary intake of omega-3 and omega-6 fatty acids was assessed using a validated self-administered dietary questionnaire (9). The Brief-type Diet History Questionnaire (BDHQ), specifically designed for evaluating nutrient and food intake, was tailored to Japanese dietary habits. It consisted of 80 questions aimed at estimating the consumption of 58 different foods. Previous studies demonstrated a strong association between the BDHQ and the biomarker for EPA, with a Spearman's correlation coefficient of 0.58 in men and 0.44 in women. Similarly, a significant correlation was observed for DHA, with values of 0.55 in men and 0.29 in women (10).
3.4. Biochemical Analysis and Training Session
Peripheral venous blood samples were collected from participants who had fasted overnight between 8:00 and 9:00 a.m. Additional peripheral venous blood samples were obtained following a single training session, which included a 2-hour shuttle run. During the two-hour rugby training session, players participated in various drills, and they performed high-intensity 40-meter shuttle runs in 10 sets, covering a total distance of 800 meters. A comprehensive analysis of whole blood lipidomics, with a specific focus on fatty acids, was conducted using the dried blood spot technique (11, 12). This examination provided a comprehensive evaluation of the intracellular distribution of a total of 22 fatty acids. The Omega-3 index (O3i), represented as the ratio of EPA and DHA to the total fatty acid content, was calculated (13). Rugby players were categorized based on the proposed omega-3 index (EPA+DHA) risk zones for cardiovascular disease as follows: low risk, >8%; intermediate risk, 4 – 8%; high risk, < 4% (14). This study protocol (approval number: 2013–005) was approved by the Ethics Committee.
3.5. Statistical Analysis
A total sample size of 52 subjects was required, considering statistical power (80%), alpha error (20%), and effect size (0.8). We employed the t-test, chi-square test, and Pearson's correlation coefficient for statistical analysis. Cases with missing data were excluded from the relevant analysis, and sensitivity analysis was conducted. All analyses were performed using the R software.
4. Results
A total of 60 participants were recruited. The rugby group (RG, n = 29) had higher BMI, body fat, and lean body mass compared to the control group (CG, n = 31). Among the forwards and the backs within the rugby group, the forwards exhibited greater body weight, BMI, and lean body mass, but there were no distinctions in age, height, or body fat between the two subgroups.
The RG had a significantly higher intake of omega-6 and omega-3 fatty acids than the CG. Blood omega-6 fatty acid levels did not differ between the groups; however, the RG had lower omega-3 fatty acid levels than the CG (4.4 ± 1.1 vs. 6.2 ± 1.8%), with no difference in omega-6 fatty acid levels between the groups (Figure 1). Approximately 48% of rugby players had an O3i that was considered high risk (<4%), and 52% had an O3i considered intermediate risk (4 – 8%). None of the participants had a low-risk O3i (Table 1). There was no correlation between omega-3 and omega-6 fatty acids in rugby players (r = 0.15 and -0.08, respectively). A single training session increased changes upstream of the omega-3 fatty acid pathways and reduced changes downstream of the omega-6 and omega-3 pathways (Figure 2). No correlation was found between dietary intake and blood levels of n-3 and n-6 polyunsaturated fatty acids (Table 2).
Dietary and biological status of omega-3 fatty acids in the rugby- and control groups
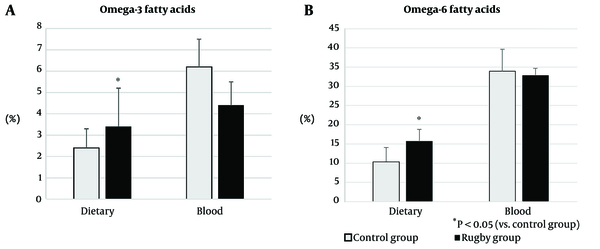
Clinical Characteristics of the Participants
Variables | Control Group (N = 31) | Rugby Group (N = 29) | Rugby Group | |
---|---|---|---|---|
Forward (n = 14) | Bucks (n = 15) | |||
Age, y | 20.8 (1.9) | 20.7 (0.7) | 20.9 (0.9) | 20.5 (0.5) |
Height, cm | 172.2 (4.9) | 174.6 (7.6) | 176.4 (8.5) | 172.8 (6.9) |
Body weight, kg | 64.9 (10.8) | 88.1 (16.0) a | 99.3 (14.5) a, b | 77.7 (9.7) a |
BMI, kg/m2 | 20.7 (4.8) | 28.8 (4.2) a | 31.9 (4.0) a, b | 25.9 (1.8) a |
Body fat, % | 17.6 (5.2) | 20.2 (6.1) a | 23.6 (5.7) a | 17.1 (4.9) |
Lean body mass, kg | 53.0 (6.2) | 69.7 (10.4) a | 75.5 (10.7) a, b | 64.2 (7.3) a |
Dietary intake, g | ||||
Omega-3 fatty acids | 2.4 (0.9) | 3.4 (1.3) a | 3.6 (1.5) a | 3.2 (0.9) a |
Omega-3 fatty acids/BW | 0.037(0.015) | 0.040 (0.016) | 0.037 (0.017) | 0.043 (0.014) |
Omega-6 fatty acids | 10.4 (3.7) | 15.7 (5.7) a | 16.1 (7.4) a | 15.2 (3.8) a |
Omega-6 fatty acids/BW | 0.16 (0.06) | 0.18 (0.07) | 0.17 (0.07) | 0.20 (0.07)a |
Whole blood | ||||
Omega-3 fatty acids | 6.2 (1.8) | 4.4 (1.1) | 4.6 (1.3) a | 4.2 (0.9) a |
Omega-6 fatty acids | 33.9 (3.1) | 32.8 (1.9) | 32.9 (1.8) | 32.7 (2.1) |
Omega-3 index, % | ||||
High risk (< 4%) | 12.9 | 48.3 a | 35.7 | 60.0 a |
Intermediate (4 - 8%) | 74.2 | 51.7 | 64.3 | 40.0 a |
Low risk (> 8%) | 12.9 | 0 a | 0 | 0 |
Effects of a single training session on omega-3 and 6 fatty acid concentrations in rugby players
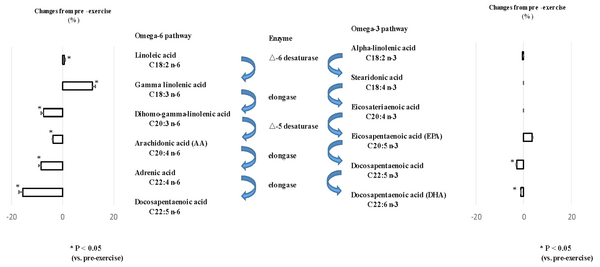
Correlation Between Dietary Intake and Blood Levels of n-3 and n-6 Polyunsaturated Fatty Acids
Variable | Control Group | Rugby Group | Rugby Group | |
---|---|---|---|---|
Forward | Bucks | |||
n-3 PUFAs | 0.05 | 0.15 | 0.21 | -0.05 |
n-6 PUFAs | 0.09 | -0.08 | -0.14 | -0.01 |
5. Discussion
This study marks the first indication that Japanese rugby players may be deficient in omega-3 fatty acids despite their higher intake of these fatty acids. Additionally, a single training session had an impact on the omega-3 and omega-6 pathways. The precise reason for the variations in the omega-3 index among the groups remains uncertain. Importantly, none of the participants in this study had taken omega-3 supplements. It is possible that differences in exercise and training routines could account for these variations, possibly influencing muscle metabolism.
The Omega-3 index (O3i), a measure of the combined DHA + EPA content, provides valuable information regarding cardiovascular disease risk: <4% is considered high risk, while >8% is considered low risk (14). Our findings are consistent with a previous study on American football athletes. The mean O3i values for Summer Olympians (14), Winter Olympians (15), and National Collegiate Athletic Association Division 1 collegiate footballers were 5.1%, 4.9%, and 4.4%, respectively. The recommended O3i target range for athletes is 8 – 11% (16). Football players face an increased risk of head injuries and cardiovascular disease (17). The O3i serves as a valuable tool for evaluating an individual's omega-3 status.
Practical recommendations for n-3 PUFAs intake for rugby players are lacking. Huang et al.'s meta-analysis revealed a positive impact on muscle mass, especially for individuals taking supplements exceeding 2 grams per day (18). Black et al. reported that 1.5 g of n-3 PUFAs supplementation for 20 professional Rugby Union players during 5 weeks of pre-season training showed a moderate beneficial effect on muscle soreness, translating into better maintenance of explosive power (3). A non-randomized study of 31 football players by Heileson et al. demonstrated cardio- and neuroprotective effects of combined 560 mg EPA+320mg DPA+2000mg DHA supplementation (19). However, among 19 male and 15 female rugby players, even with supplementation, the O3i remained in the high-risk category for some athletes who followed the recommended dietary intake of n-3 PUFAs (20). This suggests that relying solely on dietary intake may not be sufficient, and athletes may require additional dietary adjustments and n-3 PUFAs (21). Moreover, the ingestion of 6 grams of DHA can quickly lead to the attainment of a targeted O3i (>8%) in athletes within just 8 weeks. Based on the findings of this study, dietary recommendations should be tailored to each individual's O3i.
Rugby and football players are at an increased risk of head trauma (22), and recurrent head injuries can lead to lasting neurological consequences (23). Animal models have shown a decrease in neuronal DHA levels following brain injury (24, 25). Notably, two studies involving patients with severe head trauma suggested potential benefits of n-3 PUFAs acid supplementation (26, 27). A human clinical trial involving football athletes found evidence suggesting a neuroprotective effect of DHA supplementation (28). The correlation between EPA and DHA levels in skeletal muscle and red blood cells is noteworthy. College students with an O3i exceeding 4% reported reduced muscle soreness post-eccentric exercise compared to their counterparts (29). Additionally, supplementation with DHA-rich fish oil has been linked to improved cycling economy and enhanced cognitive flexibility in the executive function of endurance athletes (30, 31). Overall, human studies suggest that n-3 PUFAs supplementation may have a neuroprotective effect.
Walnuts and flaxseeds stand out as primary plant-based sources of n-3 PUFAs (32). Additionally, both walnuts and flaxseeds provide essential nutrients such as fiber, potassium, magnesium, and various non-essential compounds like polyphenols and sterols, which, when combined, are recognized for their positive impact on cardiovascular health. This study raises questions about the sources of omega-3 and omega-6 fatty acids, specifically whether they are derived from fish or plants. Further investigation, particularly into plant-based sources, is necessary to address these uncertainties and provide a comprehensive understanding of the subject.
This study possesses notable strengths, including the utilization of validated methods such as lipidomics and a reliable dietary assessment tool (BDHQ). Nevertheless, it is essential to acknowledge several limitations, including a relatively small sample size, the absence of a crossover design, the lack of assessment concerning performance and sports-related injuries, and the exclusive focus on male Japanese university rugby players. Consequently, it is important to recognize that the findings may not be universally applicable. Philpott and colleagues found that a dosage of 1.1 mg of n-3PUFA has a beneficial effect in reducing muscle soreness and elevated levels of creatine kinase in competitive soccer players after eccentric exercise (33). Escribano-Ott et al. reported that EPA (2 g) had a potential role in recovery and wellness in basketball players (34). This study suggests that the results have the potential to offer advantages to other athletic groups. We did not assess additional ratios, such as the omega-6/omega-3 fatty acid ratio. A more favorable outcome is associated with a lower ratio of omega-6 to omega-3 fatty acids, which is beneficial in reducing the risk of numerous prevalent chronic diseases in Western societies (35). It's important to note that we did not compute the omega-6/omega-3 fatty acid ratio in this study. In an observational study involving 275 non-elite runners, who were not using omega-3 supplements, the research highlighted the O3i as potential indicators linked to the risk of running-related injuries (36). It's important to note that the study did not investigate the participants' history of sports-related injuries. To substantiate these findings, additional research encompassing this specific ratio and involving larger sample sizes is necessary. In the case of collegiate women soccer players, a brief omega-3 FFQ (Food Frequency Questionnaire) demonstrated a correlation with erythrocyte omega-3 fatty acid levels, providing a practical tool for health professionals to assess omega-3 intake among this specific collegiate sports population (37). However, this current study focused on male rugby players. To gain a more comprehensive understanding of these issues, further research is necessary, including the use of a brief omega-3 FFQ and conducting prospective studies.
In conclusion, rugby players may be at a higher risk of cardiovascular disease than controls. Rugby players are exposed to repeated head impacts, which might cause neurological deficits. The O3i and lipidomics can be used to profile and monitor rugby players.
Acknowledgements
References
-
1.
Jeromson S, Gallagher IJ, Galloway SD, Hamilton DL. Omega-3 Fatty Acids and Skeletal Muscle Health. Mar Drugs. 2015;13(11):6977-7004. [PubMed ID: 26610527]. [PubMed Central ID: PMC4663562]. https://doi.org/10.3390/md13116977.
-
2.
Gammone MA, Riccioni G, Parrinello G, D'Orazio N. Omega-3 Polyunsaturated Fatty Acids: Benefits and Endpoints in Sport. Nutrients. 2018;11(1). [PubMed ID: 30591639]. [PubMed Central ID: PMC6357022]. https://doi.org/10.3390/nu11010046.
-
3.
Black KE, Witard OC, Baker D, Healey P, Lewis V, Tavares F, et al. Adding omega-3 fatty acids to a protein-based supplement during pre-season training results in reduced muscle soreness and the better maintenance of explosive power in professional Rugby Union players. Eur J Sport Sci. 2018;18(10):1357-67. [PubMed ID: 29985775]. https://doi.org/10.1080/17461391.2018.1491626.
-
4.
Fielding BA. Omega-3 index as a prognosis tool in cardiovascular disease. Curr Opin Clin Nutr Metab Care. 2017;20(5):360-5. [PubMed ID: 28768293]. https://doi.org/10.1097/MCO.0000000000000404.
-
5.
Anzalone A, Carbuhn A, Jones L, Gallop A, Smith A, Johnson P, et al. The Omega-3 Index in National Collegiate Athletic Association Division I Collegiate Football Athletes. J Athl Train. 2019;54(1):7-11. [PubMed ID: 30645147]. [PubMed Central ID: PMC6410989]. https://doi.org/10.4085/1062-6050-387-18.
-
6.
Ochi E, Tsuchiya Y. Eicosapentaenoic Acid (EPA) and Docosahexaenoic Acid (DHA) in Muscle Damage and Function. Nutrients. 2018;10(5). [PubMed ID: 29710835]. [PubMed Central ID: PMC5986432]. https://doi.org/10.3390/nu10050552.
-
7.
Yeomans C, Kenny IC, Cahalan R, Warrington GD, Harrison AJ, Hayes K, et al. The Incidence of Injury in Amateur Male Rugby Union: A Systematic Review and Meta-Analysis. Sports Med. 2018;48(4):837-48. [PubMed ID: 29299876]. [PubMed Central ID: PMC5856893]. https://doi.org/10.1007/s40279-017-0838-4.
-
8.
Nirengi S, Fujibayashi M, Furuno S, Uchibe A, Kawase Y, Sukino S, et al. Nonalcoholic Fatty Liver Disease in University Rugby Football Players. Front Endocrinol (Lausanne). 2018;9:341. [PubMed ID: 29973915]. [PubMed Central ID: PMC6019459]. https://doi.org/10.3389/fendo.2018.00341.
-
9.
Kobayashi S, Murakami K, Sasaki S, Okubo H, Hirota N, Notsu A, et al. Comparison of relative validity of food group intakes estimated by comprehensive and brief-type self-administered diet history questionnaires against 16 d dietary records in Japanese adults. Public Health Nutr. 2011;14(7):1200-11. [PubMed ID: 21477414]. https://doi.org/10.1017/S1368980011000504.
-
10.
Takayama M, Arai Y, Sasaki S, Hashimoto M, Shimizu K, Abe Y, et al. Association of marine-origin n-3 polyunsaturated fatty acids consumption and functional mobility in the community-dwelling oldest old. J Nutr Health Aging. 2013;17(1):82-9. [PubMed ID: 23299385]. https://doi.org/10.1007/s12603-012-0389-1.
-
11.
Marangoni F, Colombo C, Galli C. A method for the direct evaluation of the fatty acid status in a drop of blood from a fingertip in humans: applicability to nutritional and epidemiological studies. Anal Biochem. 2004;326(2):267-72. [PubMed ID: 15003567]. https://doi.org/10.1016/j.ab.2003.12.016.
-
12.
Meyer BJ, Sparkes C, Sinclair AJ, Gibson RA, Else PL. Fingertip Whole Blood as an Indicator of Omega-3 Long-Chain Polyunsaturated Fatty Acid Changes during Dose-Response Supplementation in Women: Comparison with Plasma and Erythrocyte Fatty Acids. Nutrients. 2021;13(5). [PubMed ID: 33922507]. [PubMed Central ID: PMC8147031]. https://doi.org/10.3390/nu13051419.
-
13.
Walker RE, Jackson KH, Tintle NL, Shearer GC, Bernasconi A, Masson S, et al. Predicting the effects of supplemental EPA and DHA on the omega-3 index. Am J Clin Nutr. 2019;110(4):1034-40. [PubMed ID: 31396625]. https://doi.org/10.1093/ajcn/nqz161.
-
14.
Harris WS, Del Gobbo L, Tintle NL. The Omega-3 Index and relative risk for coronary heart disease mortality: Estimation from 10 cohort studies. Atherosclerosis. 2017;262:51-4. [PubMed ID: 28511049]. https://doi.org/10.1016/j.atherosclerosis.2017.05.007.
-
15.
Drobnic F, Rueda F, Pons V, Banquells M, Cordobilla B, Domingo JC. Erythrocyte Omega-3 Fatty Acid Content in Elite Athletes in Response to Omega-3 Supplementation: A Dose-Response Pilot Study. J Lipids. 2017;2017:1472719. [PubMed ID: 28656110]. [PubMed Central ID: PMC5471584]. https://doi.org/10.1155/2017/1472719.
-
16.
von Schacky C, Kemper M, Haslbauer R, Halle M. Low Omega-3 Index in 106 German elite winter endurance athletes: a pilot study. Int J Sport Nutr Exerc Metab. 2014;24(5):559-64. [PubMed ID: 25203220]. https://doi.org/10.1123/ijsnem.2014-0041.
-
17.
Lust CAC, Burns JL, Jones MT, Smith SB, Choi SH, Krk M, et al. The Dose-Response Effect of Docosahexaenoic Acid on the Omega-3 Index in American Football Athletes. Med Sci Sports Exerc. 2023;55(5):865-72. [PubMed ID: 36728325]. [PubMed Central ID: PMC10090382]. https://doi.org/10.1249/MSS.0000000000003117.
-
18.
Huang YH, Chiu WC, Hsu YP, Lo YL, Wang YH. Effects of Omega-3 Fatty Acids on Muscle Mass, Muscle Strength and Muscle Performance among the Elderly: A Meta-Analysis. Nutrients. 2020;12(12). [PubMed ID: 33291698]. [PubMed Central ID: PMC7761957]. https://doi.org/10.3390/nu12123739.
-
19.
Heileson JL, Anzalone AJ, Carbuhn AF, Askow AT, Stone JD, Turner SM, et al. The effect of omega-3 fatty acids on a biomarker of head trauma in NCAA football athletes: a multi-site, non-randomized study. J Int Soc Sports Nutr. 2021;18(1):65. [PubMed ID: 34579748]. [PubMed Central ID: PMC8477477]. https://doi.org/10.1186/s12970-021-00461-1.
-
20.
Armstrong A, Anzalone AJ, Pethick W, Murray H, Dahlquist DT, Askow AT, et al. An Evaluation of Omega-3 Status and Intake in Canadian Elite Rugby 7s Players. Nutrients. 2021;13(11). [PubMed ID: 34836033]. [PubMed Central ID: PMC8620970]. https://doi.org/10.3390/nu13113777.
-
21.
Rittenhouse M, Sambuughin N, Deuster P. Optimization of Omega-3 Index Levels in Athletes at the US Naval Academy: Personalized Omega-3 Fatty Acid Dosage and Molecular Genetic Approaches. Nutrients. 2022;14(14). [PubMed ID: 35889922]. [PubMed Central ID: PMC9321651]. https://doi.org/10.3390/nu14142966.
-
22.
Daneshvar DH, Nowinski CJ, McKee AC, Cantu RC. The epidemiology of sport-related concussion. Clin Sports Med. 2011;30(1):1-17. vii. [PubMed ID: 21074078]. [PubMed Central ID: PMC2987636]. https://doi.org/10.1016/j.csm.2010.08.006.
-
23.
Montenigro PH, Alosco ML, Martin BM, Daneshvar DH, Mez J, Chaisson CE, et al. Cumulative Head Impact Exposure Predicts Later-Life Depression, Apathy, Executive Dysfunction, and Cognitive Impairment in Former High School and College Football Players. J Neurotrauma. 2017;34(2):328-40. [PubMed ID: 27029716]. [PubMed Central ID: PMC5220530]. https://doi.org/10.1089/neu.2016.4413.
-
24.
Wu A, Ying Z, Gomez-Pinilla F. Exercise facilitates the action of dietary DHA on functional recovery after brain trauma. Neuroscience. 2013;248:655-63. [PubMed ID: 23811071]. [PubMed Central ID: PMC3951948]. https://doi.org/10.1016/j.neuroscience.2013.06.041.
-
25.
Desai A, Kevala K, Kim HY. Depletion of brain docosahexaenoic acid impairs recovery from traumatic brain injury. PLoS One. 2014;9(1). e86472. [PubMed ID: 24475126]. [PubMed Central ID: PMC3903526]. https://doi.org/10.1371/journal.pone.0086472.
-
26.
Lewis M, Ghassemi P, Hibbeln J. Therapeutic use of omega-3 fatty acids in severe head trauma. Am J Emerg Med. 2013;31(1):273 e5-8. [PubMed ID: 22867826]. [PubMed Central ID: PMC3518659]. https://doi.org/10.1016/j.ajem.2012.05.014.
-
27.
Roberts L, Bailes J, Dedhia H, Zikos A, Singh A, McDowell D, et al. Surviving a mine explosion. J Am Coll Surg. 2008;207(2):276-83. [PubMed ID: 18656058]. https://doi.org/10.1016/j.jamcollsurg.2008.02.015.
-
28.
Fenton JI, Gurzell EA, Davidson EA, Harris WS. Red blood cell PUFAs reflect the phospholipid PUFA composition of major organs. Prostaglandins Leukot Essent Fatty Acids. 2016;112:12-23. [PubMed ID: 27637336]. https://doi.org/10.1016/j.plefa.2016.06.004.
-
29.
Lembke P, Capodice J, Hebert K, Swenson T. Influence of omega-3 (n3) index on performance and wellbeing in young adults after heavy eccentric exercise. J Sports Sci Med. 2014;13(1):151-6. [PubMed ID: 24570619]. [PubMed Central ID: PMC3918552].
-
30.
Hingley L, Macartney MJ, Brown MA, McLennan PL, Peoples GE. DHA-rich Fish Oil Increases the Omega-3 Index and Lowers the Oxygen Cost of Physiologically Stressful Cycling in Trained Individuals. Int J Sport Nutr Exerc Metab. 2017;27(4):335-43. [PubMed ID: 28338369]. https://doi.org/10.1123/ijsnem.2016-0150.
-
31.
Johnston DT, Deuster PA, Harris WS, Macrae H, Dretsch MN. Red blood cell omega-3 fatty acid levels and neurocognitive performance in deployed U.S. Servicemembers. Nutr Neurosci. 2013;16(1):30-8. [PubMed ID: 22748167]. https://doi.org/10.1179/1476830512Y.0000000025.
-
32.
Santos HO, Price JC, Bueno AA. Beyond Fish Oil Supplementation: The Effects of Alternative Plant Sources of Omega-3 Polyunsaturated Fatty Acids upon Lipid Indexes and Cardiometabolic Biomarkers-An Overview. Nutrients. 2020;12(10). [PubMed ID: 33081119]. [PubMed Central ID: PMC7602731]. https://doi.org/10.3390/nu12103159.
-
33.
Philpott JD, Donnelly C, Walshe IH, MacKinley EE, Dick J, Galloway SDR, et al. Adding Fish Oil to Whey Protein, Leucine, and Carbohydrate Over a Six-Week Supplementation Period Attenuates Muscle Soreness Following Eccentric Exercise in Competitive Soccer Players. Int J Sport Nutr Exerc Metab. 2018;28(1):26-36. [PubMed ID: 28871832]. https://doi.org/10.1123/ijsnem.2017-0161.
-
34.
Escribano-Ott I, Calleja-Gonzalez J, Mielgo-Ayuso J. Ergo-Nutritional Intervention in Basketball: A Systematic Review. Nutrients. 2022;14(3). [PubMed ID: 35276997]. [PubMed Central ID: PMC8839588]. https://doi.org/10.3390/nu14030638.
-
35.
Simopoulos AP. The importance of the ratio of omega-6/omega-3 essential fatty acids. Biomed Pharmacother. 2002;56(8):365-79. [PubMed ID: 12442909]. https://doi.org/10.1016/s0753-3322(02)00253-6.
-
36.
Davinelli S, Intrieri M, Ali S, Righetti S, Mondazzi L, Scapagnini G, et al. Omega-3 index and AA/EPA ratio as biomarkers of running-related injuries: An observational study in recreational runners. Eur J Sport Sci. 2023;23(1):134-42. [PubMed ID: 34694208]. https://doi.org/10.1080/17461391.2021.1998643.
-
37.
Essman M, Christifano D, Sullivan DK, Chalise P, Carbuhn A. Assessing Omega-3 Intake in Sport: the Brief Food Frequency Questionnaire and the Omega-3 Index in Collegiate Women Soccer Players. J Athl Train. 2022;57(11-12):1079-84. [PubMed ID: 35192716]. [PubMed Central ID: PMC9875705]. https://doi.org/10.4085/1062-6050-0549.21.