Abstract
Context:
Peroxisome proliferator-activated receptor γ (PPAR-γ) plays a crucial role in regulating oxidative phosphorylation and energy utilization, both of which are diminished in obesity and type 2 diabetes (T2DM), major risk factors for cardiovascular disease (CVD). It is well established that exercise, as one of the significant modifiable lifestyle factors, is associated with the expression and activity of PPAR-γ-controlled genes.Evidence Acquisition:
In this systematic review, we conducted comprehensive searches across various databases, including PubMed, ScienceDirect, and Google Scholar, for relevant literature published between January 2000 and May 2022. This study focused on published articles (in-vivo and human) in English. This review encompasses 16 studies (4 involving humans and 12 involving animals).Results:
The findings from 14 studies indicated that various types of exercise programs, including regular exercise, resistance exercise, swimming, climbing, and treadmill running, effectively improved PPAR-γ levels in individuals with T2DM, obese populations, and healthy subjects. Only two studies reported no significant improvement in PPAR-γ levels following physical activity. Notably, all studies involving subjects with T2DM showed improved PPAR-γ levels after exercise training.Conclusions:
Upon comparing various types of exercise training, the collected data suggest that all forms of aerobic exercise, regardless of their type and duration, have the potential to up-regulate PPAR-γ messenger ribonucleic acid (mRNA) expression. This study lays the foundation for further research into the association between different types of exercise training and PPAR-γ levels/activity across various human populations. However, given the limited number of human studies, further high-quality research is necessary to draw firm conclusions.Keywords
1. Context
Peroxisome proliferator-activated receptor γ (PPAR-γ) is a transcription factor that belongs to the nuclear fatty acid receptor family (1, 2). In humans, four PPAR-γ isoforms have been identified: PPAR-γ1, PPAR-γ2, PPAR-γ3, and PPAR-γ4, with PPAR-γ1, 3, and 4 encoding the same protein; nevertheless, PPAR-γ2 is exclusively expressed in adipose tissue (3). Peroxisome proliferator-activated receptor γ plays a crucial role in various physiological functions, including glucose homeostasis, lipid metabolism, and protection against oxidative stress. Disruptions in these metabolic pathways can lead to conditions such as obesity, diabetes, and cardiovascular disease (CVD).
Several drugs that activate PPAR-γ have already received approval for sale, and their cardio-protective effects have been extensively investigated, making them potential therapeutic targets for CVDs (2, 4, 5). Thiazolidinediones (TZDs), such as rosiglitazone and pioglitazone, are specific PPAR-γ ligands that act as insulin sensitizers and are currently used to treat hyperglycemia in patients with type 2 diabetes (T2DM) (6, 7). Peroxisome proliferator-activated receptor γ is reported to have a relatively high expression level in vascular smooth muscle cells (8). Due to its presence in atherosclerotic plaques, a direct link between PPAR-γ and atherosclerosis, the primary cause of coronary artery disease (CAD), has been proposed (9). With the increasing prevalence of risk factors, such as diabetes, hypertension, and obesity, and the established connection between PPAR-γ and these risk factors, PPAR-γ is emerging as a critical factor.
Regular exercise training and physical activity (PA) are important modifiable lifestyle factors that can significantly reduce the risk of CVD-related events and death by improving CVD risk factors, such as lipid profiles, blood pressure, body weight, and diabetes (10-19). Peroxisome proliferator-activated receptor γ gene expression in human adipose tissue is associated with insulin resistance and CVD risk markers. Insulin resistance is known to be caused by reduced fatty acid oxidation, particularly in skeletal muscle (20, 21). Increased PPAR-γ expression in muscle and adipose tissue in response to physical exercise might contribute to the beneficial effects of exercise on insulin sensitivity. Therefore, investigating the effect of different types of exercise training on PPAR-γ levels as a key candidate for treatment and improvement of CVD risk factors is an important area of interest.
Exercise can also help prevent muscle atrophy (22). Biking for 8 weeks has been shown to up-regulate PPAR-γ-controlled genes and induce beneficial effects in skeletal muscle in healthy individuals (23). It has been reported that exercise protects against angiotensin II-induced muscle atrophy by activating PPAR-γ and suppressing miR-29b (22). Available evidence suggests that standardized exercise bouts also increase PPAR-γ activity. This systematic review investigates, for the first time, the effect of different types of PA on PPAR-γ expression and activity, aiming to determine whether the type of PA or health condition can influence the effectiveness of PPAR-γ.
2. Evidence Acquisition
2.1. Search Strategy
The PubMed, ScienceDirect, and Google Scholar databases were searched for relevant literature published between January 2000 and May 2022. The phrases “training", “physical activity", “exercise", AND “Peroxisome proliferator activator receptor gamma", “PPAR-γ", were searched for in the title, keywords, and abstract. Boolean operators 'AND' and 'OR' were used to connect the search terms. Keywords from separate categories were combined with “AND", whereas those from the same category were combined with “OR". Additionally, the literature and references listed in relevant reviews were checked. In the search, subject headers and unrestricted text were integrated, and the search algorithm was modified in accordance with the characteristics of the various databases. The search strategy in PubMed as an example has been mentioned here: (((Training [Title/Abstract]) OR (physical activity [Title/Abstract])) OR (exercise [Title/Abstract])) AND (Peroxisome proliferator activator receptor gamma (PPAR-γ [Title/Abstract]))).
2.2. Study Selection
The study selection process followed the Preferred Reporting Items for Systematic Reviews and Meta-Analyses (PRISMA) guidelines (24). Selected articles were imported into the EndNote Reference Manager (version 8.1), and both automated and manual procedures were used to remove duplicates. Two independent reviewers initially assessed the papers based on their titles and abstracts, followed by a full-text screening in accordance with the qualifying criteria. Any disagreements during the study selection process were resolved through consensus with a third reviewer.
2.3. Eligibility Criteria
For inclusion in this review, studies (cohort, clinical trials, and experimental) had to meet the following criteria: Studies that were written in English, included subjects (both humans and animals) who underwent PA and exercise training programs, and assessed PPAR-γ levels after the intervention. This review excluded case reports, editorials, case series, and review articles.
2.4. Study Selection
The full text of the included studies was carefully reviewed, and information was extracted, including the first author’s name, publication date, study design, details of PA and training programs, reported outcomes regarding PPAR-γ changes, and any other relevant information.
3. Results
3.1. Search Results and Study Selection
The initial database search yielded a total of 118 articles. Figure 1 illustrates the detailed study selection process. In summary, after screening the titles and abstracts, 41 of the retrieved articles underwent full-text screening. Ultimately, 16 studies met the inclusion criteria and were included in this review, with 4 involving humans (23, 25-27) and the remainder involving animals. Given the limited number of human studies on this topic and the desire for a comprehensive review, the current review included all studies, regardless of their quality. Tables 1 and 2 show the characteristics of the included studies and the extracted data.
PRISMA flowchart for study selection (Abbreviations: PPAR-γ, peroxisome proliferator-activated receptor γ; PA, physical activity)
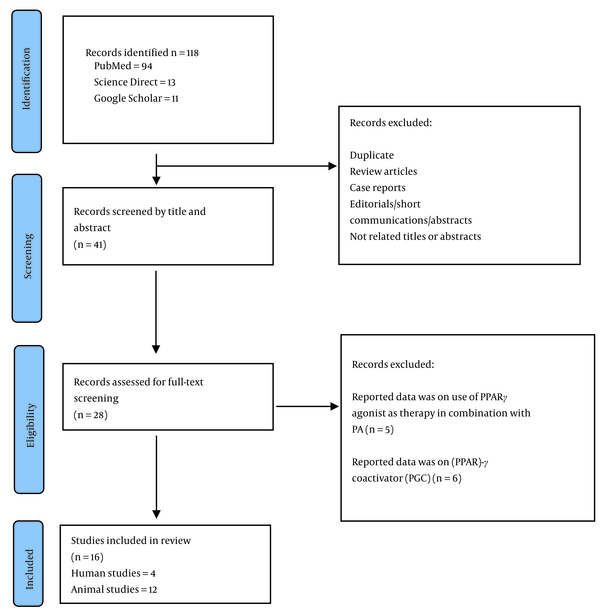
Characteristics and Extracted Data of the Included Human Studies
Authors/Year | Country | Design | Population | Intervention | Outcomes |
---|---|---|---|---|---|
Hoseini et al. 2022 (26) | Iran | Single-blinded, controlled RCT | Human/T2DM (48 males) (aged 35 - 50 years) | AE for 20-40 min/day, 3 days per week for 8 weeks | A significant increase of PPAR-γ mRNA expression level after exercise |
Fatone et al. 2010 (27) | Italy | Comparative study | Human/T2DM; 8 subjects (2 females and 6 males), aged 38 to 74 years | 1-year exercise program consisting of 2 weekly sessions of 140 min that combined aerobic and resistance circuit training | A significant increase in PPAR-γ mRNA expression level after exercise (P = 0.024) |
Tunstall et al. 2002 (25) | Australia | Clinical trial | Human/healthy (N =7) (3 males, 4 females, 28.9 ± 3.1 years | 9-day AE (60 min cycling per day) | PPAR-γ mRNA expression level was unaltered after AE and at 3 h post-AE |
Thomas et al. 2012 (23) | UK | Cohort | Human/healthy individuals (n= 9, 32 ± 8 years) | 8-week exercise (45 min of cycling) | PPAR-γ mRNA expression levels were up-regulated |
Characteristics and Extracted Data of the Included Animal Studies
Authors/Year | Country | Design | Population/sample size | Intervention | Outcomes |
---|---|---|---|---|---|
Kawamura et al. 2004 (28) | Japan | Experimental | SHR-high fructose-fed rats (n = 40); Experimental group (n = 20) Control group (n = 20) | AE for 20 m/min, 0% grade, 60 min/day, 5 days/week | Exercise significantly upregulated the PPAR-γ mRNA expression level in all tissues and skeletal muscles, which was attenuated by temocapril. |
Kawanishi et al. 2018 (29) | Japan | Experimental | Male C57BL/6 mice; Normal diet (ND) and sedentary (n = 7), ND with exercise training (n = 5), high-fat, high-fructose water diet (HFF) and sedentary (n = 11), and HFF with exercise training (n = 11) | AE or running on a treadmill | Exercise downregulated PPAR-γ expression in the liver and macrophages. |
Kushkestani et al. 2022 (30) | Iran | Experimental | Obese T2DM-induced rats (n = 12) | 6 weeks of high-intensity AE | A significant increase in PPAR-γ mRNA expression level (P = 0.007) |
Liu et al. 2015 (31) | China | Experimental | Male C57BL/6 mice (n = 24) (n = 8 for each group) (diet + SED); (diet + EXE); (diet + EXE + a selective PPAR-γ antagonist) | 12 weeks of AE | Exercise significantly upregulated the PPAR-γ mRNA expression level in the colon. Exercise prevents colonic inflammation in HFD-induced obesity by up-regulating PPAR-γ activity. |
Motta et al. 2016 (32) | Brazil | Experimental | Obese C57BL/6 mice | AE | Exercise significantly upregulated the PPAR-γ mRNA expression level in an HFD. |
Shirvani et al. 2021 (33) | Iran | Experimental | High fructose-fed rats (n = 32) (n = 8 per group): control, swimming, high-fat diet (HFD), swimming with HFD | 8 weeks of swimming | Swimming significantly upregulated the PPAR-γ mRNA expression level in an HFD. |
Chen et al. 2016 (34) | China | Experimental | 12-week-old male C57BL/6J mice (n = 60) | Treadmill running | Exercise is effective for restoring PPAR-γ to normal levels. |
Stotzer et al. 2015 (35) | Brazil | Experimental | Ovariectomized rats (n = 30, (n = 6/group) sham-sedentary, ovariectomized-sedentary, sham-RT, ovariectomized-RT Resistance training (RT) | 10-week climbing training on a ladder with progressive overload | Climbing significantly upregulated the PPAR-γ mRNA expression level. |
Szostak et al. 2016 (36) | France | Experimental | Apolipoprotein E-deficient mice | 3-month endurance swimming (5 days/weeks) in water at 35-36°C | Atherosclerotic lesion size was significantly reduced in the trained group compared to sedentary ones. Swimming significantly increased PPAR-γ expression in the aorta. The PPAR-γ expression was inversely correlated with the atherosclerotic plaque area. |
Zheng, F., Cai, Y. 2019 (37) | China | Experimental | HFD mice (n = 20) healthy mice (n = 10) | 12 weeks of swimming | An increased expression of PPAR-γ protein after swimming |
Amerian et al. 2021 (38) | Iran | Experimental | Male Wistar rats fed with deep frying oil (DFO) (n = 30) Healthy control (n = 6), DFO (n = 6), aerobic training + DFO (n = 6), octopamine + DFO (n = 6) and aerobic training + octopamine + DFO (n = 6) | AE for 4 weeks, 5 sessions per week | AE caused a significant decrease in reactive oxygen species (ROS) and a significant increase in PPAR-γ gene expression. |
Yazdanpazhooh et al. 2019 (39) | Iran | Comparative experimental study | T2DM induced rats (n = 14) exercise (n = 7) and control (n=7) groups | 6 weeks RT, including climbing on a stepladder (5 days/weeks) | Resistance training significantly increased PPAR-γ compared to control (P = 0.013). |
4. Discussion
In this systematic review, we investigated the association between physical exercise and PPAR-γ. The investigated 4 human studies included 1 randomized clinical trial (RCT), 1 experimental study, 1 cohort study, and 1 clinical trial. Each of these studies had a different study design. Due to the diverse nature of the studies, including human and animal research and observational and randomized trials, conducting a meta-analysis on this topic was not feasible. Therefore, a systematic review approach was adopted to collect and analyze the available literature, ensuring a comprehensive and unbiased examination of the existing research.
The current results from the 4 human studies indicated that in 75% of cases, moderate-term aerobic exercise (AE) and long-term AE significantly increased PPAR-γ mRNA expression levels (23, 26). However, one human study demonstrated that short-term AE (9 days) had no impact on PPAR-γ mRNA expression levels (25). Furthermore, the results of human studies revealed that AE significantly improved PPAR-γ levels in both individuals with T2DM and healthy subjects.
Among the 12 included animal studies, 11 papers (91%) demonstrated that various types of exercise and training programs, such as regular exercise, resistance exercise, swimming, climbing, and treadmill running, effectively improved PPAR-γ levels (28, 30-39). Only one study by Kawanishi et al. reported that AE downregulated PPAR-γ expression in the liver and macrophages (29). Both human and animal studies consistently showed that AE improved PPAR-γ mRNA or protein levels, consistent with previous reports that AE, regardless of type or duration, might up-regulate PPAR-γ mRNA expression in fat deposits (40-43).
Additionally, the present review included the study by Hoseini et al., which was the only human randomized controlled trial (RCT) in this review (26). In their study, 48 T2DM males were randomly assigned to one of four groups, each consisting of 10 subjects: exercise plus vitamin D, exercise, vitamin D, and control. Their findings after 8 weeks indicated that all groups, except the control group, experienced significant increases in antioxidant capacity (TAC), superoxide dismutase (SOD), and catalase (CAT), in addition to significant decreases in insulin resistance (homeostatic model assessment of insulin resistance [HOMA-IR]) and fasting blood glucose (FBG). Eight weeks of exercise also increased gene expression of PPAR-γ in T2DM patients, compared to the control group (26). Another study conducted by Fatone et al., involving 8 patients with T2DM who underwent 1-year combined AE and resistance training, revealed a significant increase in PPAR-γ and PPARα mRNA levels after 6 months of intervention, along with a significant reduction in FBG and HOMA-IR (27).
In a cohort study involving healthy individuals participating in an 8-week AE program, samples were collected before (pre) and after (post) standardized submaximal activity bouts (45 minutes of cycling at 70% of maximum O2 uptake, calculated at baseline) at weeks 0, 4, and 8. Plasma samples were added to peroxisome proliferator-activated receptor γ response element (PPRE)-luciferase reporter gene assays, revealing higher PPAR-γ activity after standardized exercise bouts, indicating the generation of PPAR-γ ligands during exercise. However, during the training program, increases in PPAR-γ/PPRE-luciferase activity in response to the same standardized exercise bout were blunted, suggesting that the relative exercise intensity might affect PPAR-γ ligand generation (23). It has also been suggested that exercise-induced benefits might extend to monocytes, as monocyte PPAR-γ activation has been linked to beneficial anti-diabetic effects. Therefore, exercise-induced monocyte PPAR-γ activation might provide another reason to recommend exercise for patients with T2DM (23).
However, only one study showed that exercise training reduced the amount of macrophages in the liver and down-regulated PPAR-γ mRNA gene expression levels in the liver and macrophages in a non-alcoholic steatohepatitis model in mice (29). The remaining animal experiments confirmed an increase in PPAR-γ mRNA (28, 30-36, 38) and PPAR-γ protein (37, 39) levels after exercise. A study on obese diabetic rats subjected to high-intensity interval training and a control group (5 sessions of 30 minutes per week) showed that the expression level of PPAR-γ 48 hours after the last training session was significantly increased (30). Motta et al. also reported that high-intensity interval training led to significant reductions in body mass index (BMI) and FBG, improvements in the lipid profile, and an increase in PPAR-γ levels (32).
In an experimental study by Amerian et al., 30 adult rats were fed deep-frying oil and then underwent exercise training. Amerian et al. observed that deep-frying oil consumption significantly increased reactive oxygen species (ROS) and significantly reduced PPAR-γ gene expression levels. Reactive oxygen species generated during the cooking process lead to oxidative stress, and because these products are absorbed by food and reach the circulatory system after consumption, they impact mitochondrial activity. An increase in ROS inhibits the ability of PPAR-γ to express and differentiate adipocytes, leading to a decrease in PPAR-γ gene expression. On the other hand, the duration and intensity of AE might have been such that, through brown adipose tissue adaptation and activation of antioxidant pathway factors, antioxidant enzymes, such as catalase and glutathione peroxidase, are increased, subsequently reducing oxidative stress caused by deeply heated oil in the mitochondria and further increasing PPAR-γ gene expression (38). Liu et al. also observed that exercise elevated PPAR-γ gene expression levels and activity in the colons of both high-fat diet and normal animals (31).
Peroxisome proliferator-activated receptor γ is the most extensively researched among the three PPAR subtypes. Due to alternative splicing and various promoters, PPAR-γ has two distinct isoforms, namely PPAR-γ1 and PPAR-γ2, with the latter containing 30 additional amino acids at the N-terminus (44). Peroxisome proliferator-activated receptor γ controls hundreds of genes, many of which are involved in energy, carbohydrate, and lipid metabolism. Additionally, PPAR-γ acts as a modulator of inflammation and fluid homeostasis. It is considered a master regulator of adipogenesis, being necessary and sufficient for the formation of adipocytes (45, 46). Treatment with PPAR-γ ligands inhibits inflammatory mediators (47). Peroxisome proliferator-activated receptor γ co-activator 1-alpha (PGC-1α) has been identified as a transcriptional co-activator of PPARs and is believed to be a key regulator of phenotypic adaptation induced by exercise (48).
Even in the absence of CAD, individuals with T2DM exhibit increased insulin resistance and decreased exercise capacity compared to equally active, age-matched, and body-matched healthy individuals. Additionally, these patients often have excess fat mass, along with reduced exercise capacity. Peroxisome proliferator-activated receptor γ agonists have been shown to improve insulin sensitivity, glycemic control, and lipid profiles while also affecting other CVD indicators (49-52), ultimately leading to improved exercise capacity (53, 54).
Yokota et al. demonstrated that the benefits of PPAR-γ agonists on exercise capacity in individuals with metabolic syndrome might be attributed, at least in part, to improved skeletal muscle energy metabolism, particularly fatty acid metabolism and mitochondrial function (55). However, Bastien et al. observed that in individuals with T2DM and CVD, treatment with the insulin sensitizer PPAR-γ agonist was associated with a worsening of AE capacity. This effect was primarily due to weight gain and expansion of subcutaneous fat mass despite improvements in insulin sensitivity, glycemic control, and metabolic conditions in these patients (56).
4.1. Limitations
This study has several significant limitations, including a limited number of human randomized controlled trials (RCTs) and the inability to conduct a meta-analysis due to the high degree of heterogeneity among the studies, which encompassed various study types, including human, animal, observational, and randomized studies. The aforementioned factors increase the risk of bias.
4.2. Conclusions
The data collected in this systematic review suggests that all forms of AE, regardless of type and duration, might up-regulate PPAR-γ mRNA expression in fat deposits in both healthy subjects and those with T2DM.
References
-
1.
Abbas A, Blandon J, Rude J, Elfar A, Mukherjee D. PPAR- gamma agonist in treatment of diabetes: Cardiovascular safety considerations. Cardiovasc Hematol Agents Med Chem. 2012;10(2):124-34. [PubMed ID: 22471957]. https://doi.org/10.2174/187152512800388948.
-
2.
Das SK, Chakrabarti R. Role of PPAR in cardiovascular diseases. Recent Pat Cardiovasc Drug Discov. 2006;1(2):193-209. [PubMed ID: 18221086]. https://doi.org/10.2174/157489006777442441.
-
3.
Lehrke M, Lazar MA. The many faces of PPARgamma. Cell. 2005;123(6):993-9. [PubMed ID: 16360030]. https://doi.org/10.1016/j.cell.2005.11.026.
-
4.
Mangelsdorf DJ, Evans RM. The RXR heterodimers and orphan receptors. Cell. 1995;83(6):841-50. [PubMed ID: 8521508]. https://doi.org/10.1016/0092-8674(95)90200-7.
-
5.
Takano H, Komuro I. Peroxisome proliferator-activated receptor gamma and cardiovascular diseases. Circ J. 2009;73(2):214-20. [PubMed ID: 19129679]. https://doi.org/10.1253/circj.cj-08-1071.
-
6.
Yki-Jarvinen H. Thiazolidinediones. N Engl J Med. 2004;351(11):1106-18. [PubMed ID: 15356308]. https://doi.org/10.1056/NEJMra041001.
-
7.
Barnett AH. Redefining the role of thiazolidinediones in the management of type 2 diabetes. Vasc Health Risk Manag. 2009;5(1):141-51. [PubMed ID: 19436665]. [PubMed Central ID: PMC2672454]. https://doi.org/10.2147/vhrm.s4664.
-
8.
Marx N, Schonbeck U, Lazar MA, Libby P, Plutzky J. Peroxisome proliferator-activated receptor gamma activators inhibit gene expression and migration in human vascular smooth muscle cells. Circ Res. 1998;83(11):1097-103. [PubMed ID: 9831704]. [PubMed Central ID: PMC4231720]. https://doi.org/10.1161/01.res.83.11.1097.
-
9.
Ohshima K, Mogi M, Horiuchi M. Role of Peroxisome Proliferator-Activated Receptor-gamma in Vascular Inflammation. Int J Vasc Med. 2012;2012:508416. [PubMed ID: 22888436]. [PubMed Central ID: PMC3409528]. https://doi.org/10.1155/2012/508416.
-
10.
Esmailiyan M, Amerizadeh A, Vahdat S, Ghodsi M, Doewes RI, Sundram Y. Effect of different types of aerobic exercise on individuals with and without hypertension: An updated systematic review. Curr Probl Cardiol. 2023;48(3):101034. [PubMed ID: 34718034]. https://doi.org/10.1016/j.cpcardiol.2021.101034.
-
11.
Nystoriak MA, Bhatnagar A. Cardiovascular Effects and Benefits of Exercise. Front Cardiovasc Med. 2018;5:135. [PubMed ID: 30324108]. [PubMed Central ID: PMC6172294]. https://doi.org/10.3389/fcvm.2018.00135.
-
12.
Lee IM, Paffenbarger RJ, Hennekens CH. Physical activity, physical fitness and longevity. Aging (Milano). 1997;9(1-2):2-11. [PubMed ID: 9177581]. https://doi.org/10.1007/BF03340123.
-
13.
Thompson PD, Buchner D, Pina IL, Balady GJ, Williams MA, Marcus BH, et al. Exercise and physical activity in the prevention and treatment of atherosclerotic cardiovascular disease: A statement from the Council on Clinical Cardiology (Subcommittee on Exercise, Rehabilitation, and Prevention) and the Council on Nutrition, Physical Activity, and Metabolism (Subcommittee on Physical Activity). Circulation. 2003;107(24):3109-16. [PubMed ID: 12821592]. https://doi.org/10.1161/01.CIR.0000075572.40158.77.
-
14.
Sesso HD, Paffenbarger RJ, Lee IM. Physical activity and coronary heart disease in men: The Harvard Alumni Health Study. Circulation. 2000;102(9):975-80. [PubMed ID: 10961960]. https://doi.org/10.1161/01.cir.102.9.975.
-
15.
Mora S, Cook N, Buring JE, Ridker PM, Lee IM. Physical activity and reduced risk of cardiovascular events: Potential mediating mechanisms. Circulation. 2007;116(19):2110-8. [PubMed ID: 17967770]. [PubMed Central ID: PMC2117381]. https://doi.org/10.1161/CIRCULATIONAHA.107.729939.
-
16.
Kubota Y, Evenson KR, Maclehose RF, Roetker NS, Joshu CE, Folsom AR. Physical activity and lifetime risk of cardiovascular disease and cancer. Med Sci Sports Exerc. 2017;49(8):1599-605. [PubMed ID: 28350711]. [PubMed Central ID: PMC5511058]. https://doi.org/10.1249/MSS.0000000000001274.
-
17.
Doewes RI, Gharibian G, Zadeh FA, Zaman BA, Vahdat S, Akhavan-Sigari R. An updated systematic review on the effects of aerobic exercise on human blood lipid profile. Curr Probl Cardiol. 2023;48(5):101108. [PubMed ID: 35016988]. https://doi.org/10.1016/j.cpcardiol.2022.101108.
-
18.
Valli G, Minnock D, Tarantino G, Neville RD. Delayed effect of different exercise modalities on glycaemic control in type 1 diabetes mellitus: A systematic review and meta-analysis. Nutr Metab Cardiovasc Dis. 2021;31(3):705-16. [PubMed ID: 33549457]. https://doi.org/10.1016/j.numecd.2020.12.006.
-
19.
Kelley GA, Kelley KS, Roberts S, Haskell W. Comparison of aerobic exercise, diet or both on lipids and lipoproteins in adults: A meta-analysis of randomized controlled trials. Clin Nutr. 2012;31(2):156-67. [PubMed ID: 22154987]. [PubMed Central ID: PMC3311746]. https://doi.org/10.1016/j.clnu.2011.11.011.
-
20.
DeFronzo RA, Bonadonna RC, Ferrannini E. Pathogenesis of NIDDM. A balanced overview. Diabetes Care. 1992;15(3):318-68. [PubMed ID: 1532777]. https://doi.org/10.2337/diacare.15.3.318.
-
21.
Phielix E, Schrauwen-Hinderling VB, Mensink M, Lenaers E, Meex R, Hoeks J, et al. Lower intrinsic ADP-stimulated mitochondrial respiration underlies in vivo mitochondrial dysfunction in muscle of male type 2 diabetic patients. Diabetes. 2008;57(11):2943-9. [PubMed ID: 18678616]. [PubMed Central ID: PMC2570390]. https://doi.org/10.2337/db08-0391.
-
22.
Liu Q, Chen L, Liang X, Cao Y, Zhu X, Wang S, et al. Exercise attenuates angiotensin II-induced muscle atrophy by targeting PPARgamma/miR-29b. J Sport Health Sci. 2022;11(6):696-707. [PubMed ID: 34116237]. [PubMed Central ID: PMC9729927]. https://doi.org/10.1016/j.jshs.2021.06.002.
-
23.
Thomas AW, Davies NA, Moir H, Watkeys L, Ruffino JS, Isa SA, et al. Exercise-associated generation of PPARgamma ligands activates PPARgamma signaling events and upregulates genes related to lipid metabolism. J Appl Physiol (1985). 2012;112(5):806-15. [PubMed ID: 22174394]. https://doi.org/10.1152/japplphysiol.00864.2011.
-
24.
Page MJ, McKenzie JE, Bossuyt PM, Boutron I, Hoffmann TC, Mulrow CD, et al. The PRISMA 2020 statement: An updated guideline for reporting systematic reviews. Syst Rev. 2021;10(1):89. [PubMed ID: 33781348]. [PubMed Central ID: PMC8008539]. https://doi.org/10.1186/s13643-021-01626-4.
-
25.
Tunstall RJ, Mehan KA, Wadley GD, Collier GR, Bonen A, Hargreaves M, et al. Exercise training increases lipid metabolism gene expression in human skeletal muscle. Am J Physiol Endocrinol Metab. 2002;283(1):E66-72. [PubMed ID: 12067844]. https://doi.org/10.1152/ajpendo.00475.2001.
-
26.
Hoseini R, Rahim HA, Ahmed JK. Concurrent alteration in inflammatory biomarker gene expression and oxidative stress: How aerobic training and vitamin D improve T2DM. BMC Complement Med Ther. 2022;22(1):165. [PubMed ID: 35733163]. [PubMed Central ID: PMC9214191]. https://doi.org/10.1186/s12906-022-03645-7.
-
27.
Fatone C, Guescini M, Balducci S, Battistoni S, Settequattrini A, Pippi R, et al. Two weekly sessions of combined aerobic and resistance exercise are sufficient to provide beneficial effects in subjects with Type 2 diabetes mellitus and metabolic syndrome. J Endocrinol Invest. 2010;33(7):489-95. [PubMed ID: 20142634]. https://doi.org/10.1007/BF03346630.
-
28.
Kawamura T, Yoshida K, Sugawara A, Nagasaka M, Mori N, Takeuchi K, et al. Regulation of skeletal muscle peroxisome proliferator-activated receptor gamma expression by exercise and angiotensin-converting enzyme inhibition in fructose-fed hypertensive rats. Hypertens Res. 2004;27(1):61-70. [PubMed ID: 15055257]. https://doi.org/10.1291/hypres.27.61.
-
29.
Kawanishi N, Mizokami T, Yada K, Suzuki K. Exercise training suppresses scavenger receptor CD36 expression in kupffer cells of nonalcoholic steatohepatitis model mice. Physiol Rep. 2018;6(23). e13902. [PubMed ID: 30516003]. [PubMed Central ID: PMC6279959]. https://doi.org/10.14814/phy2.13902.
-
30.
Kushkestani M, Parvani M, Moghadassi M, Baradaran R. The effect of six-week high-intensity interval training on muscle expression of FTO and PPAR-γ in obese diabetic rats. Iran J Health Sci. 2022. https://doi.org/10.18502/jhs.v10i2.9764.
-
31.
Liu WX, Wang T, Zhou F, Wang Y, Xing JW, Zhang S, et al. Voluntary exercise prevents colonic inflammation in high-fat diet-induced obese mice by up-regulating PPAR-gamma activity. Biochem Biophys Res Commun. 2015;459(3):475-80. [PubMed ID: 25701789]. https://doi.org/10.1016/j.bbrc.2015.02.047.
-
32.
Motta VF, Aguila MB, Mandarim-De-Lacerda CA. High-intensity interval training (swimming) significantly improves the adverse metabolism and comorbidities in diet-induced obese mice. J Sports Med Physical Fitness. 2015.
-
33.
Shirvani H, Mirnejad R, Soleimani M, Arabzadeh E. Swimming exercise improves gene expression of PPAR-gamma and downregulates the overexpression of TLR4, MyD88, IL-6, and TNF-alpha after high-fat diet in rat skeletal muscle cells. Gene. 2021;775:145441. [PubMed ID: 33482280]. https://doi.org/10.1016/j.gene.2021.145441.
-
34.
Chen N, Lei T, Xin L, Zhou L, Cheng J, Qin L, et al. Depot-specific effects of treadmill running and rutin on white adipose tissue function in diet-induced obese mice. J Physiol Biochem. 2016;72(3):453-67. [PubMed ID: 27192989]. https://doi.org/10.1007/s13105-016-0493-5.
-
35.
Stotzer US, Rodrigues MF, Domingos MM, Silva GH, Duarte FO, Gatto CV, et al. Resistance training suppresses intra-abdominal fatty acid synthesis in ovariectomized rats. Int J Sports Med. 2015;36(3):226-33. [PubMed ID: 25415388]. https://doi.org/10.1055/s-0034-1390494.
-
36.
Szostak J, Miguet-Alfonsi C, Berthelot A, Laurant P. Training-induced anti-atherosclerotic effects are associated with increased vascular PPARgamma expression in apolipoprotein E-deficient mice. Acta Physiol (Oxf). 2016;216(2):221-30. [PubMed ID: 26467845]. https://doi.org/10.1111/apha.12615.
-
37.
Zheng F, Cai Y. Concurrent exercise improves insulin resistance and nonalcoholic fatty liver disease by upregulating PPAR-gamma and genes involved in the beta-oxidation of fatty acids in ApoE-KO mice fed a high-fat diet. Lipids Health Dis. 2019;18(1):6. [PubMed ID: 30611282]. [PubMed Central ID: PMC6320624]. https://doi.org/10.1186/s12944-018-0933-z.
-
38.
Amerian D, Nemti NA, Azarbayjani MA, BgherPoor T. Effect of aerobic training and octopamine on stress oxidative and PPARγ gene expression in brown adipose tissue of male rats fed with deep frying oil. Razi J Med Sci. 2021;27(12):90-9.
-
39.
Yazdanpazhooh S, Banaeifar A, Arshadi S, Eizadi M. The effect of resistance training on PPARy expression in subcutaneous fat tissue of diabetic rats with high fat diet and STZ. Razi J Med Sci. 2019;26(8):68-77.
-
40.
Madsen MS, Siersbaek R, Boergesen M, Nielsen R, Mandrup S. Peroxisome proliferator-activated receptor gamma and C/EBPalpha synergistically activate key metabolic adipocyte genes by assisted loading. Mol Cell Biol. 2014;34(6):939-54. [PubMed ID: 24379442]. [PubMed Central ID: PMC3958030]. https://doi.org/10.1128/MCB.01344-13.
-
41.
Li M, Bai Y, Jianfei C, Xiaodong X, Yuanyuan D, Jing Z. Effects of different exercise intensity on PPARγ and relative index in adolescent obesity rats. Wei Sheng yan jiu= J Hygiene Res. 2014;43(5):732-7.
-
42.
Kato H, Shibahara T, Rahman N, Takakura H, Ohira Y, Izawa T. Effect of a 9-week exercise training regimen on expression of developmental genes related to growth-dependent fat expansion in juvenile rats. Physiol Rep. 2018;6(19). e13880. [PubMed ID: 30284400]. [PubMed Central ID: PMC6170879]. https://doi.org/10.14814/phy2.13880.
-
43.
Hallal PC, Victora CG, Wells JC, Lima RC. Physical inactivity: Prevalence and associated variables in Brazilian adults. Med Sci Sports Exerc. 2003;35(11):1894-900. [PubMed ID: 14600556]. https://doi.org/10.1249/01.MSS.0000093615.33774.0E.
-
44.
Tontonoz P, Hu E, Spiegelman BM. Stimulation of adipogenesis in fibroblasts by PPAR gamma 2, a lipid-activated transcription factor. Cell. 1994;79(7):1147-56. [PubMed ID: 8001151]. https://doi.org/10.1016/0092-8674(94)90006-x.
-
45.
Savage DB. PPAR gamma as a metabolic regulator: Insights from genomics and pharmacology. Expert Rev Mol Med. 2005;7(1):1-16. [PubMed ID: 15673477]. https://doi.org/10.1017/S1462399405008793.
-
46.
Rosen ED, Sarraf P, Troy AE, Bradwin G, Moore K, Milstone DS, et al. PPAR gamma is required for the differentiation of adipose tissue in vivo and in vitro. Mol Cell. 1999;4(4):611-7. [PubMed ID: 10549292]. https://doi.org/10.1016/s1097-2765(00)80211-7.
-
47.
Penas F, Mirkin GA, Vera M, Cevey A, Gonzalez CD, Gomez MI, et al. Treatment in vitro with PPARalpha and PPARgamma ligands drives M1-to-M2 polarization of macrophages from T. cruzi-infected mice. Biochim Biophys Acta. 2015;1852(5):893-904. [PubMed ID: 25557389]. https://doi.org/10.1016/j.bbadis.2014.12.019.
-
48.
Ferraro E, Giammarioli AM, Chiandotto S, Spoletini I, Rosano G. Exercise-induced skeletal muscle remodeling and metabolic adaptation: Redox signaling and role of autophagy. Antioxid Redox Signal. 2014;21(1):154-76. [PubMed ID: 24450966]. [PubMed Central ID: PMC4048572]. https://doi.org/10.1089/ars.2013.5773.
-
49.
Chigurupati S, Dhanaraj SA, Balakumar P. A step ahead of PPARgamma full agonists to PPARgamma partial agonists: Therapeutic perspectives in the management of diabetic insulin resistance. Eur J Pharmacol. 2015;755:50-7. [PubMed ID: 25748601]. https://doi.org/10.1016/j.ejphar.2015.02.043.
-
50.
Bertrand OF, Poirier P, Rodes-Cabau J, Rinfret S, Title LM, Dzavik V, et al. Cardiometabolic effects of rosiglitazone in patients with type 2 diabetes and coronary artery bypass grafts: A randomized placebo-controlled clinical trial. Atherosclerosis. 2010;211(2):565-73. [PubMed ID: 20594555]. https://doi.org/10.1016/j.atherosclerosis.2010.06.005.
-
51.
Negro R, Mangieri T, Dazzi D, Pezzarossa A, Hassan H. Rosiglitazone effects on blood pressure and metabolic parameters in nondipper diabetic patients. Diabetes Res Clin Pract. 2005;70(1):20-5. [PubMed ID: 16126119]. https://doi.org/10.1016/j.diabres.2005.02.012.
-
52.
Parulkar AA, Pendergrass ML, Granda-Ayala R, Lee TR, Fonseca VA. Nonhypoglycemic effects of thiazolidinediones. Ann Intern Med. 2001;134(1):61-71. [PubMed ID: 11187421]. https://doi.org/10.7326/0003-4819-134-1-200101020-00014.
-
53.
Regensteiner JG, Bauer TA, Reusch JE. Rosiglitazone improves exercise capacity in individuals with type 2 diabetes. Diabetes Care. 2005;28(12):2877-83. [PubMed ID: 16306548]. https://doi.org/10.2337/diacare.28.12.2877.
-
54.
Kadoglou NP, Iliadis F, Angelopoulou N, Perrea D, Liapis CD, Alevizos M. Beneficial effects of rosiglitazone on novel cardiovascular risk factors in patients with Type 2 diabetes mellitus. Diabet Med. 2008;25(3):333-40. [PubMed ID: 18307460]. https://doi.org/10.1111/j.1464-5491.2007.02375.x.
-
55.
Yokota T, Kinugawa S, Hirabayashi K, Suga T, Takada S, Omokawa M, et al. Pioglitazone improves whole-body aerobic capacity and skeletal muscle energy metabolism in patients with metabolic syndrome. J Diabetes Investig. 2017;8(4):535-41. [PubMed ID: 27930876]. [PubMed Central ID: PMC5497029]. https://doi.org/10.1111/jdi.12606.
-
56.
Bastien M, Poirier P, Brassard P, Arsenault BJ, Bertrand OF, Despres JP, et al. Effect of PPARgamma agonist on aerobic exercise capacity in relation to body fat distribution in men with type 2 diabetes mellitus and coronary artery disease: a 1-yr randomized study. Am J Physiol Endocrinol Metab. 2019;317(1):E65-73. [PubMed ID: 30964707]. https://doi.org/10.1152/ajpendo.00505.2018.