Abstract
Background:
Non-steroidal Selective Androgen Receptor Modulators (SARMs) are currently a group of drug candidates with anabolic effects, tissue selectivity, and the potential to improve the therapeutic process in a number of socially significant diseases. There is insufficient data on their effects during exercise and on their adverse side effects.Objectives:
Our objective was to examine the role of selective androgen receptor modulators for the physical working capacity of the body, hematological and biochemical parameters, and to evaluate any side effects of SARMs. We studied the effects of ostarine (S-22, MK-2866, GTx-024, Enobosarm) alone or in combination with submaximal training in sexually mature male Wistar rats.Methods:
The rats used were divided into a treadmill-trained group and a sedentary group. Half of each group received either ostarine or vehicle for 8 weeks (n = 10 each). We performed functional tests and blood analysis at the end of the experiment to analyze clinical chemistry and hematological indicators.Results:
Ostarine did not affect maximal sprint speed, VO2max, and running economy, while submaximal training increased these parameters. Ostarine increased total plasma cholesterol and energy expenditure and decreased plasma glucose concentration. Neither treatment changed the oxygen-carrying capacity of the blood, the respiratory quotient, the concentration of triglycerides and LDL-cholesterol, or creatine kinase. Combined treatment neutralized the negative effect of ostarine on plasma total cholesterol levels.Conclusions:
Ostarine did not affect the examined hematological and physical working capacity parameters, but it changed some clinical chemistry indicators. Training improved the physical working capacity but did not affect the clinical chemistry and hematological parameters. Further research is needed to clarify other aspects of the influence of non-steroidal SARMs on the physical working capacity and possible side effects during long-term use, as their administration, along with physical activity, is becoming popular among healthy adult bodybuilders in gyms.Keywords
Selective Androgen Receptor Modulators (SARMs) Ostarine Wistar Rats VO2max
1. Background
Selective Androgen Receptor Modulators (SARMs) are androgen receptor ligands. They are divided into two main groups: Steroidal and non-steroidal. The steroid group of modulators has been known since the middle of the last century, while the non-steroidal group was discovered just in 1998. The latter shows selectivity of their action and has strong anabolic effects, as well as weak androgenic ones. This gives non-steroidal SARMs an advantage over Anabolic Androgenic Steroids (AAS) in terms of avoiding their side effects, which include inducing left ventricular dysfunction, increasing the incidence of sudden cardiac death, liver damage, stunting, testicular atrophy, thickening of the vocal cords, hair loss, disorders in the menstrual cycle in women, thyroid dysfunction, and acne, among others (1, 2). The number of substances in various classes of the non-steroidal group is constantly increasing. There is no approved drug from this group yet, but non-steroidal SARMs are in various phases of clinical trials (3). Ostarine (also known as S-22, MK-2866, GTx-024, and Enobosarm) is the substance in the most advanced phase of research belonging to the class of arylpropionamides. According to the data available, non-steroidal SARMs are potential candidates for the treatment of some socially significant diseases, such as Lou Gherig's disease, dermatomyositis, osteoporosis, breast cancer, sarcopenia, various types of cachexia, benign prostatic hyperplasia, and hypogonadism (4).
In a study of postmenopausal women and men over 45 years of age with cancer, it was found that in the ostarine-treated groups (after intake of 1 or 3 mg doses for 113 days), a significant increase in the lean body mass was observed, as well as an improvement in physical performance (5, 6). The non-steroidal SARM group increases muscle mass, making them suitable for improving physical work capacity and sports performance. For this reason, since 2008, non-steroid SARMs have been included in the list of prohibited substances of the World Anti-doping Agency (WADA), falling under the category "other anabolic agents" in Section S1.2 (7). However, non-steroidal SARMs can be purchased online by anyone. The most common profile of a SARM user is a male between the ages of 18 - 29 years. Additionally, SARMs are widely used without prior consultation with a doctor. In an online survey, more than 96% of the participants reported an increase in muscle mass (8). There is an increasing number of positive doping tests in professional athletes due to the abuse of the mentioned group of substances. At the moment, the most appropriate methods for identifying representatives of SARMs during a doping test include mass spectrometry, gas/liquid chromatography, and especially the combination of liquid chromatography and mass spectrometry (9, 10).
A study performed in 2017 found that only 52% of 44 products offered online actually contained a non-steroidal SARM (11). In addition, the concentration of non-steroidal SARMs in a given product may differ from what is expected; most often, it is lower. Non-steroidal androgen receptor modulators can be included in the composition of various nutritional supplements, which leads to the risk of a positive doping test if sufficient attention is not paid to the product label. In some cases, users may be misled about the presence of SARMs by the labeling of names other than the trade name (e.g., S-22, MK-2866, GTx-024, or Enobosarm instead of ostarine; LGD 4033 instead of ligandrol, etc.) (11). The most commonly used substances include ostarine, ligandrol, testolone, and andarine.
Non-steroidal selective androgen receptor modulators are promising therapeutic alternatives for a large group of diseases, although there is still no officially approved drug from their group. They could be used as a replacement therapy instead of anabolic steroids and would improve the quality of life of patients. There is insufficient data about the effects of their intake combined with physical exercise. Non-steroidal SARMs are available to both professional athletes and amateurs. There is also data on toxicity associated with the use of non-steroidal SARMs when taken at doses many times higher than the clinically effective ones (12, 13). More information is needed regarding these SARM aspects.
2. Objectives
The purpose of our study was to determine the presence of some adverse side effects of the use of a non-steroidal SARM, ostarine, as well as its effects in combination with training on indices of the physical working capacity and hematological and clinical chemistry indices.
3. Methods
3.1. Experimental Animals
In our study, we used 40 sexually mature (3.5-month-old) male Wistar rats. They had a body weight of 160 - 200 g. The animals were housed in individual metabolic cages at 22 - 24°C, a 12/12 h light/dark cycle, and controlled humidity, with access to standard rat chow and water ad libitum. Rat body weight was monitored weekly, and food intake was monitored daily. The test protocol of the study was approved by the Committee of Scientific Ethics at the Medical University in Plovdiv and the Committee of Ethical Treatment of Animals at the Bulgarian Food Safety Agency (BFSA).
3.2. Training Program
Since treadmill exercise is a skill that must be acquired by test animals, before the start of the experiment, all rats ran on the EXER-3R treadmill for small test animals (Columbus Instruments, Columbus, OHIO, USA) 5 min daily for 3 days per week at a speed of 25 m/min and a 5° declination, for 2 weeks. This duration of exercise does not lead to any adaptive changes (14). Rats that refused to run on the treadmill were not included in the experiment. We selected 40 running rats, which were then divided into 4 groups (n = 10 for each group): Non-training group (sedentary, untrained control) treated with vehicle (S + V), non-training (sedentary) group treated with ostarine (S + O), training group treated with vehicle (trained control, T + V), and training group treated with ostarine (T + O).
Training rats were subjected to submaximal treadmill exercise for 8 weeks. They ran at a treadmill speed of 25 m/min, at a 5° declination for 5 days per week. During the first training day, the duration of the exercise was 20 min. This was increased by 5 minutes each day until a duration of 40 minutes on the fifth training day. This exercise duration was maintained until the end of the experiment. To maintain motor skills, with a view to participating in the functional tests, the rats from the non-training groups also ran on a treadmill 3 days a week for 5 min at the same speed and declination of the treadmill. One rat in the S + O group was withdrawn from the experiment due to health problems not related to the experiment, and another rat in the T + V group dropped out due to injury and inability to complete the training protocol.
3.3. Administration of a Non-steroidal SARM
For 8 weeks, rats in the S + O and T + O groups were injected subcutaneously (s.c.) with 0.4 mg/kg of ostarine dissolved in polyethylene glycol 300 (PEG 300) and dimethyl sulfoxide (DMSO) for 5 days per week. A solution of SARMs with the appropriate solvents can be stored for at least one year at - 20°C (15). Ostarine (Shanghai Biochempartner Co., Ltd., China), dimethyl sulfoxide (Chimtex Ltd., Bulgaria), and PEG 300 (Merck, Germany) were used in the study. Vehicle-treated animals were injected only with vehicles of the same volume s.c. Since rats have different metabolism and smaller body surface area than humans, the human dose sufficient to improve total lean body mass (0.04 mg/kg (16)) was converted to the rat dose using the Nair and Jacob formula and adjusted to the previous study in rats (17, 18).
3.4. Maximal Sprinting Speed
At the beginning and after the end of the experiment, all rats were subjected to a Maximal Sprinting Speed (MSS) test to determine the maximal sprint speed. The test was performed by running on a treadmill with an initial treadmill speed of 25 m/min and a 5° declination for 3 min. The treadmill speed was then increased to 45 m/min for 30 s and by another 10 m/min every 30 s. The test ended when it became impossible for the rat to maintain its position on the treadmill belt. The highest speed maintained by the rat for 15 s was taken as the maximal sprinting speed (19).
Maximal oxygen consumption, Running economy, Respiratory quotient, and Energy expenditure
At the end of the experiment, all groups were subjected to the test for maximal oxygen consumption (VО2max), according to Bedford et al. (20). The test was performed after two days of rest. During each of the exercise steps, to examine the oxygen consumption (VО2) and Respiratory Quotient (RQ), we used an apparatus for studying the gas exchange of small laboratory animals, Oxymax System (Columbus Instruments, Columbus, OH, USA). The volume of supplied air was 4.5 l/min. Before conducting the test, the O2 sensor was calibrated with a reference gas mixture, and the CO2 sensor was calibrated with pure nitrogen and a reference gas mixture. The protocol we used involved a stepwise increase in the speed and declination of the treadmill as follows: Step I: 15 m/min, 5°; step II: 19 m/min, 10°; step III: 27 m/min, 10°; step IV: 27 m/min, 15°; step V: 30 m/min, 15°; step VI: 35 m/min, 15°; VII step: 40 m/min, 15°. The duration of each step was three min (21). Each animal was placed in the isolated chamber on the treadmill for 10 min before the start of the exercise. The VО2 value was measured at each step, and the maximum value recorded was taken as VО2max. The criteria of Bedford et al. (20) were used to determine VO2max. Rats were removed from the test when they reached a plateau effect or when complete exhaustion occurred and they were unable to maintain their position on the treadmill.
The RQ was calculated by software as the ratio of VCO2 exhaled to VO2 consumed (Oxymax System, software v. 5.51, Columbus Instruments, Columbus, OH, USA). For the peak value of RQ, we took the value of the indicator recorded when VO2max was reached. As a method for calculating EE at different load levels and peak loads, we used indirect calorimetry. Energy expenditure was calculated by software as a function of oxygen consumption and RQ (Oxymax System, software v. 5.51, Columbus Instruments, Columbus, OH, USA). The following formula was used: Energy expenditure = (3.815 + 1.232RQ) VO2
3.5. Hematological and Clinical Chemistry Indicators
Mixed blood was collected in monovets after the decapitation of the test animals. Red Blood Cell (RBC), White Blood Cell (WBC), platelet (PLT) count, hemoglobin (HGB) concentration, hematocrit (Hct) values, and Mean Erythrocyte Volume (MCV) were measured with an ADVIA 2120i hematology analyzer (Siemens, Germany).
Serum concentrations of blood glucose, cholesterol, triglycerides, LDL-cholesterol, and Creatine Kinase (CK) were examined with a Konelab 60i clinical chemistry analyzer (Thermo Fisher Scientific, Vantaa, Finland) according to original programs.
3.6. Statistical Analyses
The sample size was calculated with the aid of G-Power analysis (G*Power program, Heinrich-Heine-University Düsseldorf, Germany) using the data from our previous studies (21, 22) F-test (analysis of variance) was applied to calculate the sample size with a power (1-β error) of 80% and α-type I error of 0.05. Ten animals per group were considered sufficient to detect the possible effect of the treatments based on these calculations and our previous experience.
Statistical analyses were performed using SPSS v. 19.0. program. A two-way analysis of variance (ANOVA) was performed to assess the impact of the main effects of ostarine treatment (O) and submaximal endurance training (T), as well as their interactions. In the case of significant interactions between ostarine treatment and submaximal endurance training, the Tukey post hoc test was used to assess between-group differences. A paired t-test was used to analyze the intra-group differences in the results. Differences at P < 0.05 were considered statistically significant. All data are presented as the mean ± Standard Error of the Mean (SEM).
4. Results
4.1. Effects on Body Weight and Food Intake
At the beginning of the experiment, rats from the four groups, S + V, S + O, T + V, and T + O, had practically the same body mass (Appendix 1A). In the course of the experiment, all groups gradually increased their weight. In the weekly weight monitoring of the animals, no significant differences were found either due to endurance training or the administration of ostarine (P > 0.05, two-way ANOVA). In all the experimental weeks, there was also no significant interaction between treatment and exercise (P > 0.05, two-way ANOVA) (Appendix 1A).
Ostarine-treated groups consumed more food than vehicle-treated groups during the second (P = 0.044), fifth (P = 0.036), sixth (P = 0.005), and seventh (P = 0.003) weeks. In the eighth week, there was again a trend towards higher consumption in the ostarine-treated groups (P = 0.054) (two-way ANOVA, Appendix 1B). The training groups had a higher food consumption than the non-training groups at the fifth (P = 0.037, two-way ANOVA) and seventh weeks (P = 0.034, two-way ANOVA). In the eighth week, there was a tendency for higher consumption in the training groups (P = 0.050, two-way ANOVA). There was no significant interaction between the two factors throughout the experiment (P > 0.05, two-way ANOVA) (Appendix 1B).
4.2. Effects on Maximal Sprinting Speed
At the baseline, all groups had a similar maximal sprinting speed (Figure 1, Table 1). In the end, only the trained controls had a significantly higher maximal sprinting speed compared to the baseline (P = 0.019, paired t-test, Figure 1). Endurance training had a significant main effect, with the training groups having a higher maximal sprinting speed than the non-training groups (P = 0.010, two-way ANOVA). The main effect of ostarine, as well as the interaction O with T, were not significant (P > 0.05, two-way ANOVA) (Table 1).
Maximal sprinting speed of the groups at baseline compared to the end of the experiment. * - P = 0.019 for group T + V at the end compared to baseline
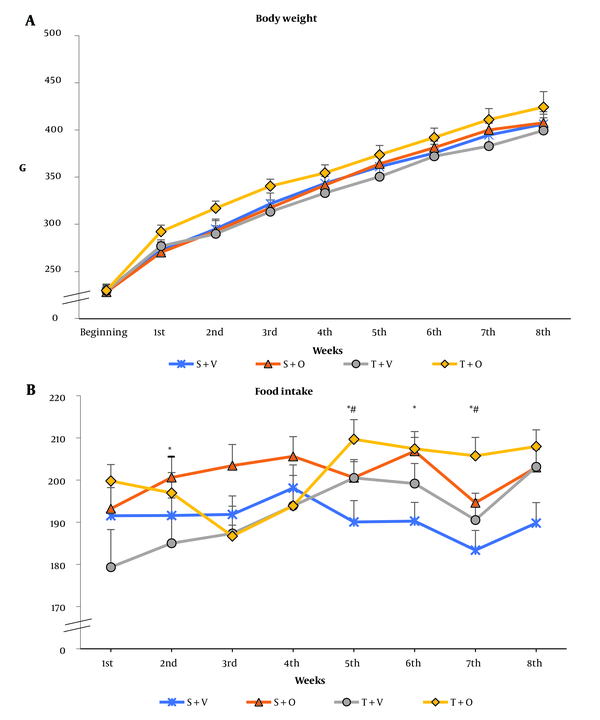
Functional Tests at the End of the Experiment a
Variables | (N = 19) | P-Value | |||||
---|---|---|---|---|---|---|---|
T | S | V | O | T vs. S | V vs. O | T x O | |
MSS (m/min) | 69 ± 1.6 | 62.5 ± 1.6 | 67 ± 1.6 | 64.5 ± 1.6 | 0.01 | 0.304 | 0.153 |
VO2max (ml O2/kg/min) | 66.8 ± 0.9 | 61.2 ± 0.9 | 63.5 ± 0.9 | 64.5 ± 0.9 | 0.000 | 0.443 | 0.231 |
RQ | 1.07 ± 0.006 | 1.08 ± 0.006 | 1.08 ± 0.006 | 1.07 ± 0.006 | 0.405 | 0.427 | 0.113 |
Energy expenditure (kcal) | 1.63 ± 0.05 | 1.19 ± 0.05 | 1.31 ± 0.05 | 1.51 ± 0.05 | 0.000 | 0.011 | 0.055 |
VO2-1 step (ml O2/kg/min) | 32.9 ± 0.8 | 32.1 ± 0.8 | 31.7 ± 0.8 | 33.3 ± 0.8 | 0.524 | 0.196 | 0.598 |
VO2-2 step (ml O2/kg/min) | 42.3 ± 0.8 | 43.7 ± 0.8 | 42.2 ± 0.8 | 43.8 ± 0.8 | 0.261 | 0.201 | 0.576 |
VO2-3 step (ml O2/kg/min) | 52.2 ± 0.9 | 52.8 ± 0.9 | 51.9 ± 0.9 | 53.1 ± 0.9 | 0.638 | 0.347 | 0.652 |
1 step VO2 % of VO2max | 48 ± 1.4 | 54 ± 1.4 | 50 ± 1.4 | 51 ± 1.4 | 0.006 | 0.411 | 0.302 |
2 step VO2 % of VO2max | 63 ± 1.4 | 71 ± 1.4 | 66 ± 1.4 | 68 ± 1.4 | 0.000 | 0.473 | 0.215 |
3 step VO2 % of VO2max | 78 ± 1.3 | 86 ± 1.3 | 82 ± 1.3 | 82 ± 1.3 | 0.000 | 0.732 | 0.578 |
4.3. Effects on Maximal Oxygen Consumption (VO2max)
At the end of the experiment, the maximal oxygen consumption of the test groups was examined (Table 1). The training groups had higher maximal oxygen consumption than the non-training groups (P = 0.000, two-way ANOVA). Ostarine administration had no significant main effect, and the interaction between treatment and exercise was also insignificant (P > 0.05, two-way ANOVA) (Table 1).
4.4. Effects on Running Economy (VO2max)
At the end of the experiment, neither ostarine treatment nor endurance training had a significant impact on the running economy during the first 3 steps of the VO2max test (P > 0.05, two-way ANOVA) (Table 1). There was no significant interaction between ostarine and training (P > 0.05, two-way ANOVA) (Table 1). Submaximal training had a significant main effect, lowering VO2 at each of the exercise steps when analyzed as a percentage of VO2max. In the training animals, the value at the first step was lower by 6% (P = 0.006, two-way ANOVA), at the second step by 8% (P = 0.000, two-way ANOVA), and by 8% at the third step (P = 0.000, two-way ANOVA) (Table 1). Administration of ostarine had no significant effect on VO2 as a percentage of VO2 max, and the interaction between treatment and exercise was also insignificant (P > 0.05, two-way ANOVA) (Table 1). The VO2 values obtained during steps 4, 5, and 6 of the maximal oxygen consumption test were not analyzed due to the very small and unequal number of measurements recorded in different groups.
4.5. Effects on Energy Expenditure During VO2max
The training rats had higher energy expenditure than non-training rats (P = 0.000, two-way ANOVA) (Table 1). Ostarine-treated groups had a higher energy expenditure compared to the vehicle-treated groups (P = 0.011, two-way ANOVA) (Table 1).
There was a clear tendency for interaction between ostarine and exercise (P = 0.055, two-way ANOVA): T + O group had a higher energy expenditure than every other group, S + V group (1.8 ± 0.07 kcal vs. 1.17 ± 0.06 kcal, P = 0.000, Tukey post-hoc test), S + O group (1.8 ± 0.07 kcal vs. 1.22 ± 0.05 kcal, P = 0.000, Tukey post-hoc test), and T + V group (1.8 ± 0.07 kcal vs. 1.46 ± 0.08 kcal, P = 0.013, Tukey post-hoc test), in sequence. The T + V group had greater energy expenditure than the two sedentary groups, the S + V group (1.46 ± 0.08 kcal vs. 1.17 ± 0.06 kcal, P = 0.031, Tukey post-hoc test) and S + O group (1.46 ± 0.08 kcal vs. 1.22 ± 0.05 kcal, P = 0.048, Tukey post-hoc test), in sequence.
4.6. Effects on RQ value at VO2max
Submaximal training and ostarine treatment had no significant main effect on RQ values at VO2max (P > 0.05, two-way ANOVA) (Table 1). We found no significant interaction between treatment and training (P > 0.05, two-way ANOVA) (Table 1).
4.7. Effects on Hematological Parameters
Statistical analysis found no significant main effect of submaximal exercise or ostarine treatment on RBC count, HGB concentration, Hct values, MCV, and PLT count (P > 0.05, two-way ANOVA) (Table 2). There was no significant interaction between the two factors for these parameters (P > 0.05, two-way ANOVA) (Table 2).
Clinical Chemistry and Hematological Indicators at the End of the Experiment a
Variables | N = 19 | P-Value | |||||
---|---|---|---|---|---|---|---|
T | S | V | O | T vs. S | V vs. O | T x O | |
Cholesterol (mmol/l) | 1.66 ± 0.04 | 1.69 ± 0.04 | 1.56 ± 0.04 | 1.80 ± 0.04 | 0.682 | 0.001 | 0.004 |
LDL (mmol/l) | 0.41 ± 0.02 | 0.36 ± 0.02 | 0.38 ± 0.02 | 0.39 ± 0.02 | 0.168 | 0.621 | 0.101 |
Triglycerides (mmol/l) | 0.45 ± 0.03 | 0.43 ± 0.03 | 0.39 ± 0.03 | 0.48 ± 0.03 | 0.743 | 0.095 | 0.430 |
Glucose (mmol/l) | 5.87 ± 0.3 | 5.67 ± 0.3 | 6.48 ± 0.3 | 5.06 ± 0.3 | 0.687 | 0.007 | 0.984 |
CK (U/l) | 5101 ± 260 | 5301 ± 260 | 5199 ± 260 | 5204 ± 260 | 0.591 | 0.989 | 0.312 |
RBC (1012/L) | 8.48 ± 0.22 | 8.14 ± 0.22 | 8.17 ± 0.22 | 8.45 ± 0.22 | 0.286 | 0.398 | 0.976 |
HGB (g/L) | 159.95 ± 4.05 | 155.60 ± 4.05 | 155.15 ± 4.05 | 160.40 ± 4.05 | 0.453 | 0.366 | 0.684 |
Hct (L/L) | 0.46 ± 0.01 | 0.46 ± 0.01 | 0.46 ± 0.01 | 0.46 ± 0.01 | 0.925 | 0.996 | 0.566 |
MCV (fL) | 55.2 ± 1.3 | 57.3 ± 1.3 | 57.6 ± 1.3 | 54.9 ± 1.3 | 0.172 | 0.301 | 0.359 |
WBC (109/L) | 8.23 ± 0.74 | 6.15 ± 0.74 | 7.47 ± 0.74 | 6.91 ± 0.74 | 0.054 | 0.599 | 0.336 |
PLT (109/L) | 594 ± 55.05 | 493 ± 55.05 | 535 ± 55.05 | 552 ± 55.05 | 0.203 | 0.828 | 0.493 |
Ostarine treatment had no significant main effect on WBC count (P > 0.05, two-way ANOVA), whereas a trend was found for a higher number of WBC in the training groups compared to the non-training groups (P = 0.054, two-way ANOVA) (Table 2). There was no significant interaction between ostarine and exercise for WBC (P > 0.05, two-way ANOVA) (Table 2).
4.8. Effects on Clinical Chemistry Indicators
The ostarine-treated rats had a higher total cholesterol concentration than vehicle-treated rats (P = 0.001, two-way ANOVA) (Table 2). Endurance training had no significant main effect on cholesterol level (P > 0.05, two-way ANOVA), whereas the interaction between the two factors was significant (P = 0.004, two-way ANOVA) (Table 2). The serum cholesterol of the rats of the S + O (sedentary + ostarine) group was higher compared to the S + V (sedentary controls) group (1.92 ± 0.07mmol/l с/у 1.47 ± 0.05 mmol/l, P = 0.000, Tukey post-hoc test) and compared to the T + V (training controls) group (1.92 ± 0.07 mmol/l с/у 1.65 ± 0.07 mmol/l, P = 0.045, Tukey post-hoc test). The S + O group showed a tendency for higher levels compared to the T + O (training + ostarine) group (1.92 ± 0.07 mmol/l vs. 1.68 ± 0.07 mmol/l, P = 0.080, Tukey post-hoc test). No significant differences were found between the two training groups (P > 0.05, Tukey post-hoc test).
Serum triglycerides, LDL-cholesterol, and creatine kinase concentrations did not differ between rats treated with ostarine and those treated with vehicle (P > 0.05, two-way ANOVA) (Table 2). There was neither a significant main effect of training nor a significant interaction between ostarine and exercise for the three parameters (P > 0.05, two-way ANOVA) (Table 2).
Rats treated with ostarine had a significantly lower plasma glucose concentration than those taking a placebo (P = 0.007, two-way ANOVA) (Table 2). Statistical analysis revealed neither a significant main effect of training nor a significant interaction between the two factors for glucose (P > 0.05, two-way ANOVA) (Table 2).
5. Discussion
In the present study, we studied the effect of ostarine intake alone or in combination with training on physical working capacity on hematological and clinical chemistry parameters in adult male rats. To our knowledge, this is the first study to examine the effects of treatment with non-steroidal SARMs ostarine combined with submaximal training on VO2max, running economy, and maximal sprinting speed in an adult male rat model. According to our results, ostarine did not induce changes in VO2max and VO2submax in either training or non-training rats. This is a similar result to that observed in the study with nandrolone decanoate (anabolic androgenic steroid) on the same indices of the physical working capacity (21). As known, VO2max depends mainly on the volume of blood reaching the muscles (the cardiac output) and the oxygen capacity of the blood (23, 24). The lack of effect of ostarine on the oxygen-carrying capacity of the blood is probably one of the reasons for the lack of change in VO2max. Also, MSS depends on maximal anaerobic power and other neuromuscular factors (22, 25). In contrast to ostarine, endurance training significantly increased MSS, VO2max, and running economy, which confirms the results of other studies presenting the beneficial effects of training on the physical working capacity of the body (26). Endurance-training athletes have a higher running economy compared to non-training individuals. This means that their VO2submax values are lower, which corresponds to lower energy expenditure and greater efficiency at submaximal effort (23, 24). There is insufficient data on the effects of SARMs on energy expenditure and respiratory quotient. Our results showed that ostarine did not affect the two indices, but further research is needed for a definitive conclusion. The observed increase in energy expenditure in the training groups is well-known (26).
The decrease in glucose levels observed in our study supports the results of a 3-month clinical study that reported a reduction in glucose levels under ostarine treatment in 60 older men and 60 postmenopausal women (16). Submaximal training alone did not affect glucose, and the interaction between training and ostarine effects was also not significant. In another study, it was reported that exogenous dihydrotestosterone intake can impair glucose metabolism by increasing the rate of gluconeogenesis in the liver and decreasing glucose uptake by adipose tissue (27). The molecular mechanisms responsible for the specific effects of SARMs have not been elucidated yet. One possible explanation is the recruitment of a different spectrum of coactivators/corepressors when binding to the androgen receptor in comparison with the anabolic androgenic steroids (28). Further research is needed to clarify the mechanisms.
Further plasma analysis revealed increased total cholesterol concentration in ostarine-treated rats, which could be interpreted as an adverse side effect of the non-steroidal SARM. The negative effect of ostarine on total cholesterol was neutralized by endurance training in our study. Despite the lack of effect of ostarine for 8 weeks on the plasma concentration of triglycerides, the effect could be more pronounced with longer treatment. We did not find any effect of ostarine on LDL-cholesterol concentration. The non-steroidal SARM-2f decreased the concentration of the following plasma lipids after 28 days of administration in monkeys: LDL-cholesterol, triglycerides, and total cholesterol (29). In 76 healthy men, LGD-4033 (ligandrol), at doses of 0.1, 0.3, and 1 mg for 21 days, reduced plasma triglyceride levels but did not affect LDL-cholesterol and total cholesterol (30). The different effects of SARMs on the lipid profile can be explained by the fact that the cellular response depends on the chemical structure of the respective SARM (31) since the SARMs reported in previous studies (28, 29) belong to different classes of non-steroidal selective androgen receptor modulators. For example, ostarine belongs to the arylpropionamide class, whereas ligandrol to the pyrrolidinyl-benzonitrile class (10).
Similar to our study, no effect of ostarine on creatine kinase has been reported in orchiectomized male rats (32). In a study of young, healthy men after administration of GSK2881078 (a non-steroidal member of the indole class), there were side effects such as muscle soreness and creatine kinase elevation. However, these effects were observed after discontinuation of ostarine treatment during the follow-up period and were not considered to be drug-related (33). The CK levels do not increase regularly after exercise, although there is some interindividual variability, and strenuous exercise may increase creatine kinase levels in some individuals (34).
Neither ostarine nor combined treatment changed the number of any blood cell type or the concentration of HGB, Hct, and MCV. In a previous study with nandrolone decanoate, a similar lack of effect on hematological indices was observed (21). The non-steroidal SARM LY305, in healthy volunteers, also did not induce significant changes in hematocrit values (35). A similar lack of effect on platelet concentration was reported in a clinical case of hepatotoxicity due to abuse of ostarine by a middle-aged man (36). Unlike SARMs, anabolic androgenic steroids increase platelet count and the propensity of this type of blood cell to aggregate (37). In a study of patients with prostatectomy due to prostate cancer, the treatment with OPK88004 caused an increase in hemoglobin concentration and hematocrit value but no change in erythrocyte and leukocyte counts (38). The pressure exerted by training increases the leukocyte count, which corresponds to the fact that exercise is known to affect leukocyte count by increasing WBCs during and immediately after training (39). This has been attributed to an increase in blood flow, the release of catecholamines, or an increase in heart rate after exercise (39-41).
In our previous studies, we found that the anabolic androgenic steroid nandrolone decanoate, alone or combined with submaximal training, showed a similar lack of effect on body weight, like ostarine (21). In other studies, ostarine treatments did not affect body weight and food intake in ovariectomized or orchiectomized rats (17, 32). In the present study, submaximal exercise and ostarine increased food intake during some experimental weeks, which could be explained by increased energy expenditure in the rats.
5.1. Limitations
In the present study, we did not examine the liver functions (AST and ALT) as it was not one of our target organs. Another limitation of the present study is the lack of baseline measurement of some parameters such as RQ, energy expenditure, and hematological and clinical chemistry indicators. Only one non-steroidal androgen receptor modulator (ostarine) was used for 8 weeks in this study. Longer studies with different representatives of SARMs could provide additional information about the effects of these molecules. Future studies can examine the dose-dependent effects of SARMs when combined with training on a wider range of clinically important parameters.
5.2. Conclusions
In conclusion, ostarine did not affect the MSS, VO2max, or VO2submax indices, which are factors that determine the physical working capacity of the body. This corresponded to the lack of effect on the erythrocyte count and hemoglobin concentration. Regarding the plasma lipids, ostarine did not affect triglyceride and LDL-cholesterol concentrations but increased plasma cholesterol levels, which can be defined as an adverse side effect. Creatine kinase did not change under ostarine treatment, indicating that the treatment was not harmful to the muscle tissue. Ostarine increased energy expenditure and food intake, reduced plasma glucose concentration, and had no effect on the body weight of the rats. Submaximal training increased MSS, VO2max, VO2submax, energy expenditure, and food intake. Combined treatment had no synergistic effect, except that training neutralized the negative effect of ostarine on plasma total cholesterol levels. Further research is needed to clarify other aspects of the influence of non-steroidal SARMs on the physical working capacity, as well as the manifestation of their adverse effects during long-term use, as their administration, along with physical activity, is becoming popular among healthy adult bodybuilders in gyms.
Acknowledgements
References
-
1.
Chen J, Kim J, Dalton JT. Discovery and therapeutic promise of selective androgen receptor modulators. Mol Interv. 2005;5(3):173-88. [PubMed ID: 15994457]. [PubMed Central ID: PMC2072877]. https://doi.org/10.1124/mi.5.3.7.
-
2.
Sullivan ML, Martinez CM, Gennis P, Gallagher EJ. The cardiac toxicity of anabolic steroids. Prog Cardiovasc Dis. 1998;41(1):1-15. [PubMed ID: 9717856]. https://doi.org/10.1016/s0033-0620(98)80019-4.
-
3.
Mohideen H, Hussain H, Dahiya DS, Wehbe H. Selective Androgen Receptor Modulators: An Emerging Liver Toxin. J Clin Transl Hepatol. 2023;11(1):188-96. [PubMed ID: 36479151]. [PubMed Central ID: PMC9647117]. https://doi.org/10.14218/JCTH.2022.00207.
-
4.
Zhang X, Sui Z. Deciphering the selective androgen receptor modulators paradigm. Expert Opin Drug Discov. 2013;8(2):191-218. [PubMed ID: 23231475]. https://doi.org/10.1517/17460441.2013.741582.
-
5.
Dobs AS, Boccia RV, Croot CC, Gabrail NY, Dalton JT, Hancock ML, et al. Effects of enobosarm on muscle wasting and physical function in patients with cancer: a double-blind, randomised controlled phase 2 trial. Lancet Oncol. 2013;14(4):335-45. [PubMed ID: 23499390]. [PubMed Central ID: PMC4898053]. https://doi.org/10.1016/S1470-2045(13)70055-X.
-
6.
Solomon ZJ, Mirabal JR, Mazur DJ, Kohn TP, Lipshultz LI, Pastuszak AW. Selective Androgen Receptor Modulators: Current Knowledge and Clinical Applications. Sex Med Rev. 2019;7(1):84-94. [PubMed ID: 30503797]. [PubMed Central ID: PMC6326857]. https://doi.org/10.1016/j.sxmr.2018.09.006.
-
7.
Thevis M, Schanzer W. Detection of SARMs in doping control analysis. Mol Cell Endocrinol. 2018;464:34-45. [PubMed ID: 28137616]. https://doi.org/10.1016/j.mce.2017.01.040.
-
8.
Efimenko IV, Valancy D, Dubin JM, Ramasamy R. Adverse effects and potential benefits among selective androgen receptor modulators users: a cross-sectional survey. Int J Impot Res. 2022;34(8):757-61. [PubMed ID: 34471228]. https://doi.org/10.1038/s41443-021-00465-0.
-
9.
Temerdashev AZ, Dmitrieva EV. Methods for the Determination of Selective Androgen Receptor Modulators. Journal of Analytical Chemistry. 2020;75(7):835-50. https://doi.org/10.1134/s1061934820070187.
-
10.
Ventura E, Gadaj A, Monteith G, Ripoche A, Healy J, Botre F, et al. Development and validation of a semi-quantitative ultra-high performance liquid chromatography-tandem mass spectrometry method for screening of selective androgen receptor modulators in urine. J Chromatogr A. 2019;1600:183-96. [PubMed ID: 31053351]. https://doi.org/10.1016/j.chroma.2019.04.050.
-
11.
Van Wagoner RM, Eichner A, Bhasin S, Deuster PA, Eichner D. Chemical Composition and Labeling of Substances Marketed as Selective Androgen Receptor Modulators and Sold via the Internet. JAMA. 2017;318(20):2004-10. [PubMed ID: 29183075]. [PubMed Central ID: PMC5820696]. https://doi.org/10.1001/jama.2017.17069.
-
12.
Barbara M, Dhingra S, Mindikoglu AL. Ligandrol (LGD-4033)-Induced Liver Injury. ACG Case Rep J. 2020;7(6). e00370. [PubMed ID: 32637435]. [PubMed Central ID: PMC7304490]. https://doi.org/10.14309/crj.0000000000000370.
-
13.
Vignali JD, Pak KC, Beverley HR, DeLuca JP, Downs JW, Kress AT, et al. Systematic Review of Safety of Selective Androgen Receptor Modulators in Healthy Adults: Implications for Recreational Users. J Xenobiot. 2023;13(2):218-36. [PubMed ID: 37218811]. [PubMed Central ID: PMC10204391]. https://doi.org/10.3390/jox13020017.
-
14.
Lambert MI, Noakes TD. Dissociation of changes in VO2 max, muscle QO2, and performance with training in rats. J Appl Physiol (1985). 1989;66(4):1620-5. [PubMed ID: 2732155]. https://doi.org/10.1152/jappl.1989.66.4.1620.
-
15.
Ventura E, Gadaj A, Buckley T, Mooney MH. Investigation of stability of selective androgen receptor modulators in urine. Food Addit Contam Part A Chem Anal Control Expo Risk Assess. 2020;37(8):1253-63. [PubMed ID: 32401172]. https://doi.org/10.1080/19440049.2020.1757165.
-
16.
Dalton JT, Barnette KG, Bohl CE, Hancock ML, Rodriguez D, Dodson ST, et al. The selective androgen receptor modulator GTx-024 (enobosarm) improves lean body mass and physical function in healthy elderly men and postmenopausal women: results of a double-blind, placebo-controlled phase II trial. J Cachexia Sarcopenia Muscle. 2011;2(3):153-61. [PubMed ID: 22031847]. [PubMed Central ID: PMC3177038]. https://doi.org/10.1007/s13539-011-0034-6.
-
17.
Roch PJ, Henkies D, Carstens JC, Krischek C, Lehmann W, Komrakova M, et al. Ostarine and Ligandrol Improve Muscle Tissue in an Ovariectomized Rat Model. Front Endocrinol (Lausanne). 2020;11:556581. [PubMed ID: 33042018]. [PubMed Central ID: PMC7528560]. https://doi.org/10.3389/fendo.2020.556581.
-
18.
Nair AB, Jacob S. A simple practice guide for dose conversion between animals and human. J Basic Clin Pharm. 2016;7(2):27-31. [PubMed ID: 27057123]. [PubMed Central ID: PMC4804402]. https://doi.org/10.4103/0976-0105.177703.
-
19.
Lambert MI. The effects of training overload on treadmill trained rats. Doctoral thesis, University of Cape Town Medical School, Cape Town, South Africa; 1990.
-
20.
Bedford TG, Tipton CM, Wilson NC, Oppliger RA, Gisolfi CV. Maximum oxygen consumption of rats and its changes with various experimental procedures. J Appl Physiol Respir Environ Exerc Physiol. 1979;47(6):1278-83. [PubMed ID: 536299]. https://doi.org/10.1152/jappl.1979.47.6.1278.
-
21.
Georgieva KN, Boyadjiev NP. Effects of nandrolone decanoate on VO2max, running economy, and endurance in rats. Med Sci Sports Exerc. 2004;36(8):1336-41. [PubMed ID: 15292741]. https://doi.org/10.1249/01.mss.0000135781.42515.17.
-
22.
Georgieva KN, Angelova PA, Gerginska FD, Terzieva DD, Shishmanova-Doseva MS, Delchev SD, et al. The effect of flutamide on the physical working capacity and activity of some of the key enzymes for the energy supply in adult rats. Asian J Androl. 2017;19(4):444-8. [PubMed ID: 27030085]. [PubMed Central ID: PMC5507090]. https://doi.org/10.4103/1008-682X.177842.
-
23.
Bassett DJ, Howley ET. Limiting factors for maximum oxygen uptake and determinants of endurance performance. Med Sci Sports Exerc. 2000;32(1):70-84. [PubMed ID: 10647532]. https://doi.org/10.1097/00005768-200001000-00012.
-
24.
Jones AM, Carter H. The effect of endurance training on parameters of aerobic fitness. Sports Med. 2000;29(6):373-86. [PubMed ID: 10870864]. https://doi.org/10.2165/00007256-200029060-00001.
-
25.
Psotta R, Blahus P, Cochrane DJ, Martin AJ. The assessment of an intermittent high intensity running test. Journal of sports medicine and physical fitness. 2005;45(3):248.
-
26.
Noakes T. Lore of running. Human kinetics; 2003.
-
27.
Andrisse S, Billings K, Ma Y, Xue P, Wolfe A, Wu S. Low Dose DHT Impairs Glucose Metabolism Via Increased Hepatic Gluconeogenic Output and Decreased Adipose Tissue Glucose Uptake. The FASEB Journal. 2016;30(S1). https://doi.org/10.1096/fasebj.30.1_supplement.1247.11.
-
28.
Furuya K, Yamamoto N, Ohyabu Y, Morikyu T, Ishige H, Albers M, et al. Mechanism of the tissue-specific action of the selective androgen receptor modulator S-101479. Biol Pharm Bull. 2013;36(3):442-51. [PubMed ID: 23449329]. https://doi.org/10.1248/bpb.b12-00885.
-
29.
Morimoto M, Yamaoka M, Hara T. A selective androgen receptor modulator SARM-2f activates androgen receptor, increases lean body mass, and suppresses blood lipid levels in cynomolgus monkeys. Pharmacol Res Perspect. 2020;8(1). e00563. [PubMed ID: 32030892]. [PubMed Central ID: PMC7005530]. https://doi.org/10.1002/prp2.563.
-
30.
Basaria S, Collins L, Dillon EL, Orwoll K, Storer TW, Miciek R, et al. The safety, pharmacokinetics, and effects of LGD-4033, a novel nonsteroidal oral, selective androgen receptor modulator, in healthy young men. J Gerontol A Biol Sci Med Sci. 2013;68(1):87-95. [PubMed ID: 22459616]. [PubMed Central ID: PMC4111291]. https://doi.org/10.1093/gerona/gls078.
-
31.
Nyquist MD, Ang LS, Corella A, Coleman IM, Meers MP, Christiani AJ, et al. Selective androgen receptor modulators activate the canonical prostate cancer androgen receptor program and repress cancer growth. J Clin Invest. 2021;131(10). [PubMed ID: 33998604]. [PubMed Central ID: PMC8121509]. https://doi.org/10.1172/JCI146777.
-
32.
Roch PJ, Wolgast V, Gebhardt MM, Boker KO, Hoffmann DB, Saul D, et al. Combination of selective androgen and estrogen receptor modulators in orchiectomized rats. J Endocrinol Invest. 2022;45(8):1555-68. [PubMed ID: 35429299]. [PubMed Central ID: PMC9270269]. https://doi.org/10.1007/s40618-022-01794-7.
-
33.
Clark RV, Walker AC, Andrews S, Turnbull P, Wald JA, Magee MH. Safety, pharmacokinetics and pharmacological effects of the selective androgen receptor modulator, GSK2881078, in healthy men and postmenopausal women. Br J Clin Pharmacol. 2017;83(10):2179-94. [PubMed ID: 28449232]. [PubMed Central ID: PMC5595940]. https://doi.org/10.1111/bcp.13316.
-
34.
Kindermann W. Creatine Kinase Levels After Exercise. Dtsch Arztebl Int. 2016;113(19):344. [PubMed ID: 27232364]. [PubMed Central ID: PMC4904530]. https://doi.org/10.3238/arztebl.2016.0344a.
-
35.
Krishnan V, Patel NJ, Mackrell JG, Sweetana SA, Bullock H, Ma YL, et al. Development of a selective androgen receptor modulator for transdermal use in hypogonadal patients. Andrology. 2018;6(3):455-64. [PubMed ID: 29527831]. https://doi.org/10.1111/andr.12479.
-
36.
Bedi H, Hammond C, Sanders D, Yang HM, Yoshida EM. Drug-Induced Liver Injury From Enobosarm (Ostarine), a Selective Androgen Receptor Modulator. ACG Case Rep J. 2021;8(1). e00518. [PubMed ID: 34368386]. [PubMed Central ID: PMC8337042]. https://doi.org/10.14309/crj.0000000000000518.
-
37.
Choi SM, Lee BM. Comparative safety evaluation of selective androgen receptor modulators and anabolic androgenic steroids. Expert Opin Drug Saf. 2015;14(11):1773-85. [PubMed ID: 26401842]. https://doi.org/10.1517/14740338.2015.1094052.
-
38.
Pencina KM, Burnett AL, Storer TW, Guo W, Li Z, Kibel AS, et al. A Selective Androgen Receptor Modulator (OPK-88004) in Prostate Cancer Survivors: A Randomized Trial. J Clin Endocrinol Metab. 2021;106(8):2171-86. [PubMed ID: 34019661]. [PubMed Central ID: PMC8277210]. https://doi.org/10.1210/clinem/dgab361.
-
39.
Sand KL, Flatebo T, Andersen MB, Maghazachi AA. Effects of exercise on leukocytosis and blood hemostasis in 800 healthy young females and males. World J Exp Med. 2013;3(1):11-20. [PubMed ID: 24520541]. [PubMed Central ID: PMC3905589]. https://doi.org/10.5493/wjem.v3.i1.11.
-
40.
Hilberg T, Prasa D, Sturzebecher J, Glaser D, Schneider K, Gabriel HH. Blood coagulation and fibrinolysis after extreme short-term exercise. Thromb Res. 2003;109(5-6):271-7. [PubMed ID: 12818250]. https://doi.org/10.1016/s0049-3848(03)00283-4.
-
41.
McCarthy DA, Perry JD, Melsom RD, Dale MM. Leucocytosis induced by exercise. Br Med J (Clin Res Ed). 1987;295(6599):636. [PubMed ID: 3117270]. [PubMed Central ID: PMC1257766]. https://doi.org/10.1136/bmj.295.6599.636.