Abstract
Objectives:
The aerobic energy-delivery system substantially contributes to energy consumption while playing soccer. Fatigue and recovery in soccer are hence closely related to this system’s efficiency, however research emphasizing this relationship is lacking. This study aimed at examining if it is possible to detect post-match fatigue and recovery in soccer players by exploring markers (e.g. oxygen consumption, heart rate, and blood lactate) of the aerobic energy-delivery system’s efficiency during a steady-state running test in the laboratory.Methods:
Seventeen well-trained male soccer players (age of 21.94 (± 3.5) years, body mass of 77.95 (± 6.76) kg, and a stature of 180.2 (± 5.98)) completed a repeated measures analysis where a sub-maximal steady-state running test was performed 3 times; a rested condition (pre-match test) served as baseline values, immediately after match (post-match test) served as the rate of fatigue, and 24 hours later (24-hour post-match test) served as the state of recovery.Results:
The one-way repeated measures analysis of variance (ANOVA) revealed significant effect (P ≤ 0.01) on all variables (body mass, oxygen consumption, heart rate, respiratory exchange ratio, blood lactate, and rating of perceived exertion). Multivariate partial eta squared analysis further indicated that this effect for all variables was interpreted as a very large effect size (R ≥ 0.47). Regarding pairwise comparison, all variables in the post-match test differed (P ≤ 0.05) to the pre-match test. In the example, oxygen consumption increased from pre-match to post-match from 175.59 to 183.02 (mL.kg-0.25·min-1). Concerning the 24-hour post-match test, heart rate and blood lactate was found to be significantly different (P ≤ 0.05) compared to the pre-match test. The remaining variables were however, at this point, about to be re-established to their pre-match values.Conclusions:
In this study, markers of aerobic energy-delivering system were proved functional in detecting post-match fatigue and recovery in soccer players.Keywords
Aerobic Performance Ability Fatigue Recovery Soccer Laboratory Testing
1. Background
The physiological match demands and the number of matches of top-level international soccer seem to have increased in the recent years (1-3). This development has made top-level soccer more physically demanding and in order to enable soccer players to perform at their highest level, and to avoid overload and injuries, previous research has highlighted the need for good recovery between matches (4, 5). Hence, it is considered important to understand the recovering process after match-play (4). The aim in the recovery phase is to restore the players’ performance ability; it is about re-establishing the baseline levels, which the players had before the match took place (6, 7). Three phases of fatigue are identified during soccer (8, 9). First, evidence of a temporary fatigue following the most intense periods of the match is proven to exist (2, 8). In this phase, soccer players’ ability to perform sprints and high-intensity running is reduced (10-12). Various physiological factors, such as glycogen depletion, reduced pH, lowered/empty creatine phosphate stores, and accumulation of extracellular potassium, have been claimed to be possible explanations behind this phase (8, 13, 14).
Secondly, lower levels of high-intensity activity at the beginning of the match’s second half, compared to the other parts of the match, is found (15, 16). The most plausible explanation for this finding is lowered muscle temperature after the 15-minute half-time break (9, 17).
Third, a fatiguing phase visible towards the end of each half of the match, especially towards the end of the second half, is found (10, 15). In this phase a reduction in sprinting and high-intensity running is detected. This phase is mainly explained by a lack of available glycogen (18), indicated by findings of lowered muscle fibre glycogen and lowered blood lactate, as well as increased levels of free fatty acid and plasma glycerol in the blood, towards the end of soccer matches (8, 18). Muscle damage (both mechanical disruptions and subsequent inflammatory processes) (7, 19, 20), dehydration and hyperthermia (16) were also pointed out as possible factors behind this more sustained fatigue phase. Although caused by different underlying mechanisms, the 3 fatiguing phases in soccer should be considered as linked together in a chain (21). An alteration in one part of the chain might effect other parts, but this separateness also implies that the different phases are not necessarily directly related to one another (8). In this paper, only the more sustained fatigue, described as post-match fatigue (6), was scrutinized.
Due to the significant contribution of the aerobic energy-delivering system during soccer matches (22, 23), and the multifactorial variables of physical performance in soccer (10, 24, 25), it seems important to approach fatigue and recovery process after soccer play using markers of this system. Furthermore, it has also been suggested that in sports, such as soccer, knowledge about the recovery process that extends the short-term effects are needed (26). Hence, the main purpose of this study was to scrutinize if it was possible to detect fatigue in soccer players by examining markers of the aerobic energy-delivery systems’ efficiency, immediately after the match. Secondly, another aim was to examine the players’ state of recovery 24 hours later.
2. Methods
2.1. Participants
Twenty-one soccer players provided their written consent to participate in the study. Four players did not complete the study and consequently, there were seventeen well-trained male soccer players with a mean (± SD) anaerobic threshold at 11.75 (± 1.56) km/h, Vo2max of 64.48 (± 7.01) mL/kg/min, age of 21.94 (± 3.5) years, body mass of 77.95 (± 6.76) kg, and a stature of 180.2 (± 5.98) cm, who participated in this study. The study was conducted during the soccer season, implying that all players were training and competing regularly (4 to 8 training sessions per week and 1 to 2 matches per week). This study was approved by the data protection official for research (project number 22048) and the University ethics committee, and was carried out, according to the declaration of Helsinki. Before the experiment was carried out, the players were familiarized with the testing procedures.
2.2. Preliminary Tests
The preliminary tests were conducted to establish the player’s aerobic capacity. To measure Anaerobic Threshold (AT) a protocol of 5 to 7 that increased in speed of 1 km/hour every fifth minute was carried out. Before each increase in speed a blood sample was taken by finger stick and the lactate level analysed. To define AT, a lactate level of 4.0 mmol/L-1 (OBLA), as first introduced by Sjodin and Jacobs, (27), was applied. Then, after a 10-minute pause, a maximal oxygen consumption test (VO2max) was conducted. It was completed as a continuous incremental protocol with load increases every 30 seconds, with a total duration of 5 to 6 minutes. VO2max was defined as the average of the 2 highest oxygen values, and the exact criteria used to determine VO2max was at the point when a plateau in oxygen consumption was observed with increasing load (28). Both these procedures are also described elsewhere (29, 30).
2.3. The Experiment
In the current study a repeated measures experimental design was applied. Before the experiment was conducted, all players completed preliminary tests of anaerobic threshold and maximal oxygen consumption, as well as a familiarization procedure with the experiment. For the participants, the experiment consisted of conducting a regular league soccer match and the completion of the aerobic steady-state running test, 3 times. The first time (the pre-match test) was with a resting condition 2 to 5 days prior to match-play, and served as the baseline test, which the other tests were compared with. The second time (the post-match test) was immediately post-match, and served as the fatigue test and the third time (24 hours post-match test) 24 hours after the match, served as the recovery test. The same laboratory equipment was used in every testing occasion and it was calibrated, according to the manufacturers guidelines. Regarding oxygen consumption and respiratory exchange ratio, a SensorMedics model VMAX29 (VIASYS healthcare respiratory technologies, Yorba Linda, California, USA) was used and for measuring blood lactate, a lactate pro-analyser was used (model LT-1710, Arkray, inc., Kyoto, Japan). Heart rate was measured with a Polar© pulse transmitter T31 and a Polar© pulse clock S610i (Copyright© Polar Electro OY, Kempele, Finland), and the treadmill used in this study was a Roadby RL3500 (Rodby Innovation AB, Hagby, Sweden).
2.4. The Aerobic Steady-State Running Test
The objective of the running test was to measure different markers of the aerobic energy-delivery system at a given external load. To be able to uphold high reliability, and to avoid exhaustion among players, a submaximal steady-state protocol on treadmill in the laboratory was conducted. The individually-based external load was set to a treadmill-speed corresponding to 90% of the speed achieved at anaerobic threshold, which had been detected by preliminary testing. Before the running test was initiated, the players completed a 15-minute standard warm-up session, consisting of a general and a specific part, as well as some light stretching. Furthermore, before initiating the running test, the players were weighted. The players then conducted a 10-minute long running test, where oxygen consumption, respiratory exchange ratio (RER), and heart rate (HR) were measured during the 3 final minutes of the test. Immediately when the running test was finished, a blood lactate sample by a finger prick was taken and the players were also asked to rate their perceived effort during the final three minutes of the test, using the Borg scale (6 to 20 scale).
2.5. Statistical Analysis
All data were exported to SPSS version 24 (IBM SPSS Statistics for Windows, Armonk, New York, USA) for analysis. Differences between the 3 test occasions were assessed using the Shapiro-Wilk test and were found to be normally distributed. The differences were also checked for kurtosis and skewness and found to be within an acceptable range. To explore differences between different test occasions, one-way repeated measures Analysis of Variance (ANOVA) was conducted. The size of the difference was also calculated by Partial Eta Squared and interpreted according to Cohen (31), as 0.01 = small effect, 0.06 = moderate effect, and 0.14 = large effect. The significance level was set to P ≤ 0.05 for all analyses and standard deviation, standard error, and the 95% confidence interval (95% CI) were also calculated.
3. Results
A one-way repeated measures analysis of variance (ANOVA) disclosed significant effect (P ≤ 0.01) for time of all variables (Table 1 and Figure 1). Multivariate partial eta squared further indicated that this effect for all variables should be interpreted as a very large effect size. Regarding the pairwise comparison, all variables in the post-match test differed (P ≤ 0.05) to the pre-match test. Weight, oxygen consumption, and heart rate increased by 0.86 %, 4.2%, and 3.8%, respectively, while RER and blood lactate were reduced by 4.54% and 34.03%, respectively. Also, the players rated the post-match test as being 14.82% more strenuous than the pre-match test. Concerning the 24-hour post-match test, heart rate and blood lactate (P ≤ 0.05) was found to be significantly different compared to the pre-match test. The remaining variables were however, at this point, about to be re-established to their pre-match values.
Data Shown as Mean ± CI
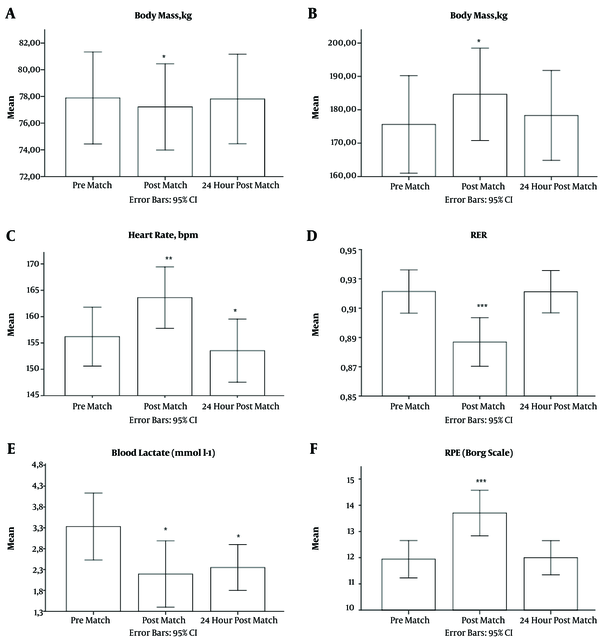
Markers of Aerobic Energy-Delivery System Measured Pre-Match, Post-Match and 24 Hours Post-Match and Assessed With One-Way Repeated Measures Analysis of Variance (ANOVA) and Bonferroni Post-Hoc Testsa
Variables, (N = 17) | Descriptive data | Multivariate tests | Pairwise comparisonb, Post-Match vs. Pre-Match | Pairwise comparisonb, 24HourPost-Match vs. Pre-Match | ||
---|---|---|---|---|---|---|
Pre-Match ± SD | Post-Match ± SD | 24HourPost-Match ± SD | Wilks’ Lambda (F; partial eta squared) | Mean difference ± SE (95% CI) | Mean difference ± SE (95% CI) | |
Body mass, kg | 77.88 ± 6.69 | 77.21 ± 6.29 | 77.80 ± 6.52 | 0.52c (F = 6.76; R = 0.47) | -0.67 ± 0.20d (0.13 to 1.21) | -0.07 ± 0.16 (-0.51 to 0.35) |
Oxygen consumption, mL.kg-0.25·min-1 | 175.59 ± 26.55 | 183.02 ± 26.03 | 176.74 ± 25.31 | 0.19e (F = 31.27; R = 0.80) | 7.42 ± 2.38d (1.06 to 13.79) | 1.15 ± 2.27 (-4.91 to 7.22) |
Heart rate, bpm | 157.24 ± 10.28 | 163.35 ± 10.67 | 152.71 ± 10.55 | 0.18e (F = 34.14; R = 0.82) | 6.11 ± 1.57c (1.89 to 10.33) | -4.52 ± 1.59d (-8.79 to 0.26) |
RER | 0.92 ± 0.02 | 0.88 ± 0.03 | 0.92 ± 0.02 | 0.40e (F = 11.10; R = 0.59) | -0.03 ± 0.008e (-0.05 to -0.01) | 0.00 ± 0.00 (-0.01 to 0.01) |
Blood lactate, mmol L-1 | 3.32 ± 1.45 | 2.19 ± 1.44 | 2.34 ± 0.99 | 0.49c (F = 7.59; R = 0.50) | -1.13 ± 0.35d (-2.07 to -0.19) | 0.98 ± 0.31d (0.15 to 1.81) |
RPE (Borg scale) | 11.94 ± 1.39 | 13.71 ± 1.68 | 12.00 ± 1.27 | 0.32e (F = 15.81; R = 0.67) | 1.76 ± 0.31e (2.60 to 0.92) | 0.05 ± 0.27 (-0.68 to 0.80) |
4. Discussion
By examining markers of the aerobic energy-delivery systems’ efficiency during a constant steady-state running test immediately after soccer match-play, and comparing these results to pre-match measurements conducted in rested condition, this study revealed post-match fatigue in soccer players. Twenty-four hours later, the markers of the aerobic energy-delivery systems efficiency was about to be re-established to pre-match values, although some differences from the rested state were still detected.
4.1. The Fatigue Test
Immediately after the soccer match, a substantial alteration in the players’ aerobic energy-delivery system compared to baseline (pre-match) values was identified. A major finding was that the oxygen consumption increased by 7.42 (mL.kg-0.25·min-1) compared to baseline. Such higher oxygen demand following physical activity is associated with decreased work efficiency and aerobic performance ability, and is often characterised as excess post-exercise oxygen consumption (EPOC) (32). The causes for this condition are numerous (32-34), yet when examining during subsequent activity, some factors have been proven to be especially important. First, an alteration in the muscle fibres’ recruitment pattern could result in less endurance effective fibres (fast twitch) being involved in muscle contraction at an earlier stage than in normal conditions and/or at submaximal intensities (33, 35). Krustrup et al. (33) revealed that changed fibre recruitment pattern caused by decreased slow twitch muscle fibre glycogen storage resulted in an increased oxygen consumption of 9% when cycling at an intensity corresponding to 50% of VO2max. A more sustained fatigue in soccer is closely related to reduced glycogen availability (2, 18, 36), and is thus a possible explanation for the present findings. Another explanation for the fast twitch fibres being involved in muscle contraction earlier than normal is damage to the slow twitch muscle fibres (37).
Secondly, an alteration in the substrate, which is primarily being metabolized, could cause increased demand for oxygen during subsequent activity. If glycogen becomes unavailable, the metabolism to a higher degree has to depend on fat, and to produce a similar amount of energy, fat-metabolism requires more oxygen compared to glycogen-metabolism (38). Thus, a higher degree of energy delivery comes from fat metabolism and is supported by present findings of RER and blood lactate, which were also significantly reduced at the post-match test compared to the pre-match results. Similar findings were also found by Krustrup et al. (33), who conducted a muscle biopsy after a cycling experiment and revealed that one group (CHO-DEP) had ~55% less available glycogen in the slow twitch fibres, and at the same time an ~8% lower RER level and a blood lactate level of approximately 43% lower, compared to another group (CON).
In line with the current findings of increased oxygen consumption at the post-match test, an increased mean heart rate of 6.11 (bpm) was found compared to baseline. Heart rate at submaximal intensities provides a good indicator of cardiovascular stress (39), and is affected by oxygen demand. Factors causing increased oxygen consumption could thus lead to a higher heart rate, and the present findings are therefore not unexpected. However, heart rate has been observed to increase without a matching increase in oxygen consumption (33). However, a gradual regulation of the autonomic nerve system following physical activity (40) and circulatory alterations due to reduced blood volume (i.e. reducing stroke volume) caused by dehydration have also been shown to increase heart rate (41). Hence, dehydration could be a possible explanation behind the current findings. This assumption is strengthened by the finding of a significant reduction in body weight after the match in the present study.
Moreover, the Borg scale was implemented in this study as a way to supply the physiological measurements. Traditionally, RPE scales are used for measuring an activity’s intensity (42), yet, the Borg scale has also been applied as an indicator of fatigue (43), and correlations between the Borg scale and increased oxygen demand and increased heart rate have been reported as r = 0.93 and r = 0.92, respectively (44). Current findings revealed a substantial increase on the Borg scale after the soccer match, compared with values from a rested condition, indicating that players were fatigued from the match, as compared to being rested at baseline. The close link between glycogen accessibility, decrements in physical function, and increases in self-reported perceived exertion was also found in a study by Chtourou et al., (45) and it was discussed that both the decrements in physical function could be causing the increased RPE while this could also be the other way around.
4.2. The Recovery Test
In the 24-hour post-match test, the players’ oxygen consumption was increased by 7.42 mL.kg-0.25·min-1, compared to pre-match measurements. This indicates that full recovery was still not achieved among the players. Causes behind the increased oxygen consumption are not easy to determine, yet the re-synthesis of glycogen storages could be a possible explanation. The replenishment of glycogen has been shown to require up to 48 hours (46), and present blood lactate findings support this, as blood lactate level was still substantially lower under the 24-hour post-match test compared to the pre-match test. A reduced aerobic metabolic efficiency could thus be assumed to be the cause behind the findings of elevated oxygen in the recovery test. On the other hand, the findings of RER 24 hours after the match showed no differences when compared to baseline, and therefore does not support this assumption.
Another possible explanation for the sustained oxygen increase is exercise-induced muscle damage (EIMD). In the present study, neither markers of mechanical fatigue (muscle damage) nor muscle stiffness/soreness (Delayed onset muscle soreness: DOMS) were directly measured, yet relationships between DOMS and ratings of perceived effort (RPE) have previously been proven to exist (47). The results of the Borg scale however showed that the players did not experience the 24-hour post-match test to be more exhausting than the baseline test. Regarding heart rate, the results revealed a decrease, 24 hours after match compared to pre-match results. As previously mentioned, the factors leading to increased oxygen consumption also lead to increased heart rate, and the disparity between oxygen and heart rate findings is thus not easy to interpret. However, it could be speculated in other contributing factors, such as enhanced autonomic nerve system regulation (40), or an improved mechanical efficiency (work efficiency) (48); this may cause the lowered heart rate at this point. Furthermore, dissimilar fluid balance at different testing times (dehydration/rehydration) with its effect on blood volume, might also be an explanation (49). However, body weight at this point was approximately similar to baseline, thus suggesting that this was not a likely cause of the decreased heart rate found in the 24-hour post-match test.
4.3. Limitations of the Study
There are clearly some limitations in the current study. One was the number of participants that ideally could have been higher. Another is that there is no randomization to different experimental conditions and hence no control group. The last limitation is that there could have been more tests between the fatigue test and the recovery test to examine the recovery process more thoroughly. However, the 24-hour duration between the tests was chosen because the tests themselves were tiring, and that implementing additional tests thus could effect the time course of the recovering process. Additionally, it has been proved that the physiological responses to physical activity are altered at different times of the day (50) and hence a 24-hour cycle seems decisive. Nevertheless, these limitations show that further research have to be implemented for understanding these underlying parts of soccer performance.
4.5. Conclusion
Because of the substantial contribution the aerobic-energy delivering system has during soccer matches and due to the complexity of physical performance in soccer, it is important to scrutinize the fatigue and recovery process in soccer with measures of this very system. Furthermore, it also seems important to extend the assessment beyond short-term effects, which have mostly been explored. Hence, the aim of this study was to observe if it was possible to detect both post-match fatigue and recovery in soccer players by examining markers of the aerobic energy-delivery systems efficiency during a steady-state running test. The results revealed that this was possible as the present soccer players proved to be significantly fatigued immediately after the match where all variables differed significantly from baseline values. This enhanced strain on aerobic energy-delivery system found immediately after the match was not fully, although closely, recovered 24 hours later. Hence, by measuring markers of the aerobic energy-delivering system efficiency during a constant steady-state, running this study proved that it is possible to detect post-match fatigue and recovery in soccer players. For future evaluation, it would be interesting to assess soccer players’ rate of fatigue and recovery by the present approach at other times in this lapse of time.
Acknowledgements
References
-
1.
Di Salvo V, Baron R, Tschan H, Calderon Montero FJ, Bachl N, Pigozzi F. Performance characteristics according to playing position in elite soccer. Int J Sports Med. 2007;28(3):222-7. [PubMed ID: 17024626]. https://doi.org/10.1055/s-2006-924294.
-
2.
Bangsbo J, Mohr M, Krustrup P. Physical and metabolic demands of training and match-play in the elite football player. J Sports Sci. 2006;24(7):665-74. [PubMed ID: 16766496]. https://doi.org/10.1080/02640410500482529.
-
3.
Bloomfield J, Polman R, O'Donoghue P. Physical Demands of Different Positions in FA Premier League Soccer. J Sports Sci Med. 2007;6(1):63-70. [PubMed ID: 24149226].
-
4.
Andersson H, Raastad T, Nilsson J, Paulsen G, Garthe I, Kadi F. Neuromuscular fatigue and recovery in elite female soccer: effects of active recovery. Med Sci Sports Exerc. 2008;40(2):372-80. [PubMed ID: 18202563]. https://doi.org/10.1249/mss.0b013e31815b8497.
-
5.
Barnett A. Using recovery modalities between training sessions in elite athletes: does it help? Sports Med. 2006;36(9):781-96. [PubMed ID: 16937953]. https://doi.org/10.2165/00007256-200636090-00005.
-
6.
Nedelec M, McCall A, Carling C, Legall F, Berthoin S, Dupont G. Recovery in soccer: part I - post-match fatigue and time course of recovery. Sports Med. 2012;42(12):997-1015. [PubMed ID: 23046224]. https://doi.org/10.2165/11635270-000000000-00000.
-
7.
Nedelec M, McCall A, Carling C, Legall F, Berthoin S, Dupont G. Recovery in soccer : part ii-recovery strategies. Sports Med. 2013;43(1):9-22. [PubMed ID: 23315753]. https://doi.org/10.1007/s40279-012-0002-0.
-
8.
Mohr M, Krustrup P, Bangsbo J. Fatigue in soccer: a brief review. J Sports Sci. 2005;23(6):593-9. [PubMed ID: 16195008]. https://doi.org/10.1080/02640410400021286.
-
9.
Rampinini E, Bosio A, Ferraresi I, Petruolo A, Morelli A, Sassi A. Match-related fatigue in soccer players. Med Sci Sports Exerc. 2011;43(11):2161-70. [PubMed ID: 21502891]. https://doi.org/10.1249/MSS.0b013e31821e9c5c.
-
10.
Bradley PS, Sheldon W, Wooster B, Olsen P, Boanas P, Krustrup P. High-intensity running in English FA Premier League soccer matches. J Sports Sci. 2009;27(2):159-68. [PubMed ID: 19153866]. https://doi.org/10.1080/02640410802512775.
-
11.
Ingebrigtsen J, Bendiksen M, Randers MB, Castagna C, Krustrup P, Holtermann A. Yo-Yo IR2 testing of elite and sub-elite soccer players: performance, heart rate response and correlations to other interval tests. J Sports Sci. 2012;30(13):1337-45. [PubMed ID: 22867048]. https://doi.org/10.1080/02640414.2012.711484.
-
12.
Mohr M, Krustrup P, Bangsbo J. Match performance of high-standard soccer players with special reference to development of fatigue. J Sports Sci. 2003;21(7):519-28. [PubMed ID: 12848386]. https://doi.org/10.1080/0264041031000071182.
-
13.
Bogdanis GC, Nevill ME, Boobis LH, Lakomy HK. Contribution of phosphocreatine and aerobic metabolism to energy supply during repeated sprint exercise. J Appl Physiol (1985). 1996;80(3):876-84. [PubMed ID: 8964751].
-
14.
Reilly T, Drust B, Clarke N. Muscle fatigue during football match-play. Sports Med. 2008;38(5):357-67. [PubMed ID: 18416591]. https://doi.org/10.2165/00007256-200838050-00001.
-
15.
Krustrup P, Zebis M, Jensen JM, Mohr M. Game-induced fatigue patterns in elite female soccer. J Strength Cond Res. 2010;24(2):437-41. [PubMed ID: 20072057]. https://doi.org/10.1519/JSC.0b013e3181c09b79.
-
16.
Mohr M, Mujika I, Santisteban J, Randers MB, Bischoff R, Solano R, et al. Examination of fatigue development in elite soccer in a hot environment: a multi-experimental approach. Scand J Med Sci Sports. 2010;20 Suppl 3:125-32. [PubMed ID: 21029199]. https://doi.org/10.1111/j.1600-0838.2010.01217.x.
-
17.
Mohr M, Krustrup P, Nybo L, Nielsen JJ, Bangsbo J. Muscle temperature and sprint performance during soccer matches--beneficial effect of re-warm-up at half-time. Scand J Med Sci Sports. 2004;14(3):156-62. [PubMed ID: 15144355]. https://doi.org/10.1111/j.1600-0838.2004.00349.x.
-
18.
Krustrup P, Mohr M, Steensberg A, Bencke J, Kjaer M, Bangsbo J. Muscle and blood metabolites during a soccer game: implications for sprint performance. Med Sci Sports Exerc. 2006;38(6):1165-74. [PubMed ID: 16775559]. https://doi.org/10.1249/01.mss.0000222845.89262.cd.
-
19.
Bishop PA, Jones E, Woods AK. Recovery from training: a brief review: brief review. J Strength Cond Res. 2008;22(3):1015-24. [PubMed ID: 18438210]. https://doi.org/10.1519/JSC.0b013e31816eb518.
-
20.
Sikorski EM, Wilson JM, Lowery RP, Joy JM, Laurent CM, Wilson SM, et al. Changes in perceived recovery status scale following high-volume muscle damaging resistance exercise. J Strength Cond Res. 2013;27(8):2079-85. [PubMed ID: 23287827]. https://doi.org/10.1519/JSC.0b013e31827e8e78.
-
21.
Allen DG, Lamb GD, Westerblad H. Skeletal muscle fatigue: cellular mechanisms. Physiol Rev. 2008;88(1):287-332. [PubMed ID: 18195089]. https://doi.org/10.1152/physrev.00015.2007.
-
22.
Bangsbo J, Iaia FM, Krustrup P. Metabolic response and fatigue in soccer. Int J Sports Physiol Perform. 2007;2(2):111-27. [PubMed ID: 19124899]. https://doi.org/10.1123/ijspp.2.2.111.
-
23.
Helgerud J, Engen LC, Wisloff U, Hoff J. Aerobic endurance training improves soccer performance. Med Sci Sports Exerc. 2001;33(11):1925-31. [PubMed ID: 11689745]. https://doi.org/10.1097/00005768-200111000-00019.
-
24.
Bangsbo J, Norregaard L, Thorso F. Activity profile of competition soccer. Can J Sport Sci. 1991;16(2):110-6. [PubMed ID: 1647856].
-
25.
Bradley PS, Di Mascio M, Peart D, Olsen P, Sheldon B. High-intensity activity profiles of elite soccer players at different performance levels. J Strength Cond Res. 2010;24(9):2343-51. [PubMed ID: 19918194]. https://doi.org/10.1519/JSC.0b013e3181aeb1b3.
-
26.
Dawson B, Cow S, Modra S, Bishop D, Stewart G. Effects of immediate post-game recovery procedures on muscle soreness, power and flexiblity levels over the next 48 hours. J Sci Med Sport. 2005;8(2):210-21. [PubMed ID: 16075781]. https://doi.org/10.1016/S1440-2440(05)80012-X.
-
27.
Sjodin B, Jacobs I. Onset of blood lactate accumulation and marathon running performance. Int J Sports Med. 1981;2(1):23-6. [PubMed ID: 7333732]. https://doi.org/10.1055/s-2008-1034579.
-
28.
Svensson M, Drust B. Testing soccer players. J Sports Sci. 2005;23(6):601-18. [PubMed ID: 16195009]. https://doi.org/10.1080/02640410400021294.
-
29.
Dillern T, Ingebrigtsen J, Shalfawi SAI. Aerobic capacity and anthropometric characteristics of elite-recruit female soccer players. Serbian J Sport Sci. 2012;6(2):43-9.
-
30.
Ingebrigtsen J, Dillern T, Shalfawi SA. Aerobic capacities and anthropometric characteristics of elite female soccer players. J Strength Cond Res. 2011;25(12):3352-7. [PubMed ID: 22076087]. https://doi.org/10.1519/JSC.0b013e318215f763.
-
31.
Cohen J. Statistical power analysis for the behavioral sciences. 2 ed. Lawrence Erlbaum Associates; 1988.
-
32.
Borsheim E, Bahr R. Effect of exercise intensity, duration and mode on post-exercise oxygen consumption. Sports Med. 2003;33(14):1037-60. [PubMed ID: 14599232]. https://doi.org/10.2165/00007256-200333140-00002.
-
33.
Krustrup P, Soderlund K, Mohr M, Bangsbo J. Slow-twitch fiber glycogen depletion elevates moderate-exercise fast-twitch fiber activity and O2 uptake. Med Sci Sports Exerc. 2004;36(6):973-82. [PubMed ID: 15179167]. https://doi.org/10.1249/01.MSS.0000128246.20242.8B.
-
34.
LaForgia J, Withers RT, Gore CJ. Effects of exercise intensity and duration on the excess post-exercise oxygen consumption. J Sports Sci. 2006;24(12):1247-64. [PubMed ID: 17101527]. https://doi.org/10.1080/02640410600552064.
-
35.
Ratkevicius A, Mizuno M, Povilonis E, Quistorff B. Energy metabolism of the gastrocnemius and soleus muscles during isometric voluntary and electrically induced contractions in man. J Physiol. 1998;507 ( Pt 2):593-602. [PubMed ID: 9518716]. https://doi.org/10.1111/j.1469-7793.1998.593bt.x.
-
36.
Reilly T, Ekblom B. The use of recovery methods post-exercise. J Sports Sci. 2005;23(6):619-27. [PubMed ID: 16195010]. https://doi.org/10.1080/02640410400021302.
-
37.
Horita T, Komi PV, Hamalainen I, Avela J. Exhausting stretch-shortening cycle (SSC) exercise causes greater impairment in SSC performance than in pure concentric performance. Eur J Appl Physiol. 2003;88(6):527-34. [PubMed ID: 12560951]. https://doi.org/10.1007/s00421-002-0716-z.
-
38.
Bahr R, Hansson P, Sejersted OM. Triglyceride/fatty acid cycling is increased after exercise. Metabolism. 1990;39(9):993-9. [PubMed ID: 2392063]. https://doi.org/10.1016/0026-0495(90)90313-2.
-
39.
Borresen J, Lambert MI. Changes in heart rate recovery in response to acute changes in training load. Eur J Appl Physiol. 2007;101(4):503-11. [PubMed ID: 17687564]. https://doi.org/10.1007/s00421-007-0516-6.
-
40.
Borresen J, Lambert MI. Autonomic control of heart rate during and after exercise : measurements and implications for monitoring training status. Sports Med. 2008;38(8):633-46. [PubMed ID: 18620464]. https://doi.org/10.2165/00007256-200838080-00002.
-
41.
Short KR, Sedlock DA. Excess postexercise oxygen consumption and recovery rate in trained and untrained subjects. J Appl Physiol (1985). 1997;83(1):153-9. [PubMed ID: 9216958].
-
42.
Zouhal H, Lemoal E, Wong del P, Benounis O, Castagna C, Duluc C, et al. Physiological Responses of General vs. Specific Aerobic Endurance Exercises in Soccer. Asian J Sports Med. 2013;4(3):213-20. [PubMed ID: 24427481]. https://doi.org/10.5812/asjsm.34285.
-
43.
Polito LFT, Figueira AJ, Miranda MLJ, Chtourou H, Miranda JM, Brandão MRF. Psychophysiological indicators of fatigue in soccer players: A systematic review. Sci Sport. 2017;32(1):1-13. https://doi.org/10.1016/j.scispo.2016.09.003.
-
44.
Grant S, Aitchison T, Henderson E, Christie J, Zare S, McMurray J, et al. A comparison of the reproducibility and the sensitivity to change of visual analogue scales, Borg scales, and Likert scales in normal subjects during submaximal exercise. Chest. 1999;116(5):1208-17. [PubMed ID: 10559077]. https://doi.org/10.1378/chest.116.5.1208.
-
45.
Chtourou H, Hammouda O, Souissi H, Chamari K, Chaouachi A, Souissi N. The effect of ramadan fasting on physical performances, mood state and perceived exertion in young footballers. Asian J Sports Med. 2011;2(3):177-85. [PubMed ID: 22375237]. https://doi.org/10.5812/asjsm.34757.
-
46.
Krustrup P, Ortenblad N, Nielsen J, Nybo L, Gunnarsson TP, Iaia FM, et al. Maximal voluntary contraction force, SR function and glycogen resynthesis during the first 72 h after a high-level competitive soccer game. Eur J Appl Physiol. 2011;111(12):2987-95. [PubMed ID: 21448723]. https://doi.org/10.1007/s00421-011-1919-y.
-
47.
Scott KE, Rozenek R, Russo AC, Crussemeyer JA, Lacourse MG. Effects of delayed onset muscle soreness on selected physiological responses to submaximal running. J Strength Cond Res. 2003;17(4):652-8. [PubMed ID: 14636096]. https://doi.org/10.1519/1533-4287(2003)017<0652:EODOMS>2.0.CO;2.
-
48.
Russell AP, Wadley G, Hesselink MK, Schaart G, Lo S, Leger B, et al. UCP3 protein expression is lower in type I, IIa and IIx muscle fiber types of endurance-trained compared to untrained subjects. Pflugers Arch. 2003;445(5):563-9. [PubMed ID: 12634927]. https://doi.org/10.1007/s00424-002-0943-5.
-
49.
Gonzalez-Alonso J, Calbet JA, Nielsen B. Muscle blood flow is reduced with dehydration during prolonged exercise in humans. J Physiol. 1998;513 ( Pt 3):895-905. [PubMed ID: 9824726]. https://doi.org/10.1111/j.1469-7793.1998.895ba.x.
-
50.
Chtourou H, Hammouda O, Souissi H, Chamari K, Chaouachi A, Souissi N. Diurnal variations in physical performances related to football in young soccer players. Asian J Sports Med. 2012;3(3):139-44. [PubMed ID: 23012632]. https://doi.org/10.5812/asjsm.34604.