Abstract
Background:
Microvascular changes and precursors of atherosclerosis are frequently observed in young patients with early-onset type 1 diabetes (T1DM). Due to their known beneficial effects, physical activity is strongly recommended for its management.Objectives:
This study aimed to investigate the effects of eight weeks of organized exercise (OE) and non-organized leisure time activity (NOLTA) on determinants of cardiovascular disease and physical fitness in children with type 1 diabetes.Methods:
In this quasi-experimental study, 20 children with T1DM were randomly divided into two groups of 10: OE (n = 10, mean age = 12.60 ± 1.34 years) and NOLTA (n = 10, mean age = 12.40 ± 0.84 years). Subjects in both groups participated in their respective activities 3 days per week. Blood samples and physiological measurements were taken 48 hours before and after the 8-week training period.Results:
High-density lipoprotein (HDL) levels and physical fitness factors increased significantly in both exercise groups. Fasting blood glucose, C-reactive protein, and the cholesterol/HDL ratio were also significantly reduced in both groups. Waist circumference, glycosylated hemoglobin, and the LDL/HDL ratio were significantly reduced only in the OE group. However, the decrease in the acute phase protein pentraxin 3 was minimal and statistically insignificant.Conclusions:
In children and adolescents with T1DM, regular exercise—due to its anti-inflammatory effects and its ability to improve cardiorespiratory fitness, lipid profile, and blood glucose levels—may help reduce the severity and likelihood of cardiovascular disease in adulthood.Keywords
Diabetes Mellitus Type 1 C-reactive Protein Exercises Pentraxin3 Inflammation Lipid Profile
1. Background
Type 1 diabetes mellitus (T1DM) is a chronic autoimmune disease characterized by the destruction of beta cells in the pancreas, resulting in an absolute insulin deficiency. Studies indicate that inflammatory mediators play a significant role in T1DM, and inflammation is considered a possible factor in the pathogenesis of hyperglycemia (1). Type 1 diabetes mellitus is associated with accelerated atherosclerosis and increased cardiovascular disease (CVD), making CVD the most common cause of premature death and disability in this population (2).
Pentraxin-3 (PTX3) is a recently discovered inflammatory mediator that, by interacting with various ligands, can play a role in inflammatory responses and vascular diseases (3). Pentraxin-3 is expressed in a variety of cells, including phagocytes, smooth muscle cells, fibroblasts, glial cells, and endothelial cells, and plays an important role in immune responses (4). This versatile protein is positively associated with insulin resistance and is elevated in diabetes and diabetic nephropathy (5). Todoric et al. were the first to demonstrate in diabetic pregnant women that serum PTX3 levels are positively correlated with elevated glucose levels and negatively correlated with insulin sensitivity (6).
Increased serum PTX3 in individuals with T1DM has been identified as an indicator of diabetic nephropathy, with these patients exhibiting high PTX3 levels (7). A recent study by Li et al. also reported that elevated PTX3 levels act as an inflammatory marker in patients with T2DM and contribute to the pathogenesis of diabetic kidney disease (8). Thus, elevated PTX3 levels are observed as a cause of inflammatory conditions and kidney failure in different types of diabetes. Additionally, increased C-reactive protein (CRP) and pro-atherogenic activity of monocytes are evident in patients with T1DM, and diabetic conditions may accelerate atherogenesis and coronary disease in these individuals (9).
High-sensitivity C-reactive protein (HS-CRP) is a marker of systemic inflammation, primarily produced by hepatocytes, and is a strong predictor of cardiovascular diseases (2). High-sensitivity C-reactive protein levels are significantly higher in individuals with diabetes compared to healthy individuals, and these levels are related to BMI and abdominal fat in children with T1DM (10). Hyperglycemia and dyslipidemia are common metabolic disorders in young people with T1DM, both of which increase the risk of cardiovascular disease (CVD) (11).
Physical activity can improve metabolic profiles, cardiovascular status, and insulin sensitivity, thereby reducing the risk of mortality in children with T1DM. The health benefits associated with exercise can extend into adulthood (12). Many recent studies have reported positive effects of exercise training on HS-CRP and lipid profiles (13, 14), but results regarding PTX3 have been conflicting (15, 16).
2. Objectives
However, research on the effects of exercise on HS-CRP and PTX3 in patients with T1DM is limited. Given the beneficial effects of exercise on lipid profiles, body composition, and inflammatory status (17), it is expected that regular physical activity can reduce the severity of CVD risk. This study aimed to investigate the effects of eight weeks of organized exercise (OE) and non-organized leisure time activity (NOLTA) on inflammation-related biomarkers such as PTX3, CRP, lipid profile, blood glucose, and physical fitness in children with T1DM.
3. Methods
This study is quasi-experimental. The statistical sample consists of 20 children with T1DM, with a mean age of 12.50 ± 1.10 years (7 boys and 13 girls). The study was conducted over an 8-week period starting in June 2022, after the end of the COVID-19 quarantine restrictions. None of the subjects had participated in any regular exercise program in the past two years, and at least two years had passed since their diagnosis of T1DM. According to the health survey, none of the volunteers reported an illness or physical injury that would prevent them from exercising. Subject characteristics are shown in Table 1. Subjects were selected from eligible patients on a non-random basis. Before participation, their parents completed a general and health information questionnaire and signed written consent to participate voluntarily in the study.
Descriptive and Physiological Characteristics of Subjects a
Variables | Height (cm) | BMI (kg/m2) | Body Fat Percentage (%) | VO2 peak (mL/kg/min) | ||||
---|---|---|---|---|---|---|---|---|
Pre-test | Post-test | Pre-test | Post-test | Pre-test | Post-test | Pre-test | Post-test | |
NOLTA | 159.40 ± 11.87 | 159.77 ± 11.84 | 21.96 ± 6.61 | 22.40 ± 6.93 | 26.64 ± 8.17 | 27.04 ± 8.22 | 39.68 ± 3.72 | 42.26 ± 3.55 |
OE | 157.35 ± 8.87 | 157.85 ± 8.92 | 18.17 ± 3.61 | 18.23 ± 3.75 | 24.14 ± 8.72 | 24.43 ± 8.52 | 40.97 ± 4.54 | 43.08 ± 3.98 |
At the time of the study, the spread of coronavirus had not yet completely ceased, but due to control measures and adherence to health practices, voluntary training sessions were permitted. However, some parents preferred their children to exercise in a safer environment, such as at home. The subjects were divided into two groups of 10 each. One group engaged in organized exercise (OE) and performed calisthenics (body weight resistance exercises) at home, while the other group engaged in non-organized leisure time activities. In the OE group, resistance exercises were scheduled three times a week in the form of home training, with the intensity, duration, and load of the exercises being closely monitored.
Thus, the subjects performed their exercises once a week under the supervision of the researcher to ensure proper execution and to regulate exercise intensity and pace during their independent training sessions at home, which occurred twice a week. They recorded their exercises and sent videos of all activities to the research team weekly. To progressively increase the training load biweekly, a strategy was implemented where an additional five repetitions were added to each exercise, or the duration of each exercise was extended by five seconds. The training program for the OE group is shown in Figure 1.
Training Program for the OE group. Abbreviations: REPs, Repetitions, SECs, Seconds, OE, organized exercises.
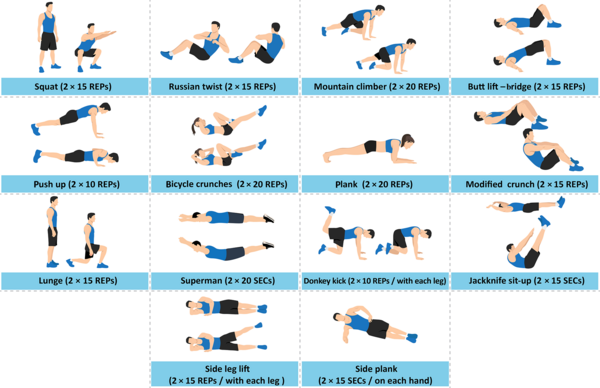
In the NOLTA group, subjects participated in leisure time activities, such as walking, running, cycling, swimming, and ball sports, three days a week, with a requirement of a total of 150 minutes of regular physical activity each week. In this group, the intensity and type of exercises were not controlled, and subjects engaged in physical activities of their choice. According to reports from the NOLTA group participants, 3 of the 10 individuals were actively involved in volleyball, 1 in basketball, 3 in soccer, 2 in swimming, and 1 in cycling. The choice of exercise forms in the NOLTA group was adapted to the participants' lifestyles and preferences, emphasizing enjoyment and satisfaction from the activity while maintaining continued participation. Participants were asked to schedule their training sessions at least 48 hours apart to ensure adequate rest between consecutive sessions. This study was conducted with the approval of the University of Isfahan Research Ethics Committee, with the number IR.UI.REC.1400.115 and it is registered in the Clinical Research Center of Iran with the number IRCT20200326046861N2.
3.1. Measurement of Physical Fitness Factors and Laboratory Methods
To measure physical fitness factors, various tests and devices were used. These included the sit-up test (in 60 seconds) for abdominal muscle endurance, the press-up test (in 60 seconds) for shoulder muscle endurance, the sit and reach test for trunk muscle flexibility, a dynamometer device for static hand muscle strength, and a body composition analyzer device by InBody for body fat percentage. The shuttle run test and the following equation were used to calculate maximum oxygen consumption (18): VO2 peak = 61.1 – 2.20 gender – 0.462 age – 0.862 BMI + 0.192 TL.
Where TL is the total number of laps covered in the 20-MST and gender is 1 for female and 0 for male. Waist circumference (WC) and hip circumference (HC) were measured with a non-elastic tape measure, with WC measured at the midpoint between the bottom of the ribs and the top of the iliac crest, and HC measured at the most prominent part of the buttocks. Body Mass Index (BMI) was calculated by dividing weight (kg) by height (m²). Blood samples and physiological index measurements were taken 48 hours before the first training session and repeated 48 hours after the last training session. PTX3 serum levels were measured using the ELISA method with the Zellbio kit from Germany.
3.2. Statistical Analysis
Statistical analysis was performed using SPSS version 25. The Shapiro-Wilk test was used to check the normality of the data, the paired sample t-test was used to assess changes within groups, and analysis of covariance (ANCOVA) was used to compare between groups. A significance level of (P < 0.05) was considered for all tests.
4. Results
The mean and standard deviation values of physical fitness factors and blood measurements in the pre- and post-test phases, as well as the percentage changes observed after eight weeks of exercise training, are shown in Tables 2 and 3. The results of the covariance test indicate that the effects of the two exercise methods are generally not significantly different, except for VO2 peak, where a significant difference was observed. The increase in VO2 peak was slightly greater in the NOLTA group. Additionally, the results of the paired t-test for within-group changes revealed a significant increase in BMI in the NOLTA group after eight weeks of training. Both training groups showed significant improvements in sit-ups, push-ups, static strength of hand muscles, VO2 peak, and HDL levels. Fasting blood sugar (FBS), hs-CRP, and the cholesterol/HDL ratio decreased significantly in both training groups. However, waist circumference (WC), glycosylated hemoglobin (HbA1c), and the LDL/HDL ratio decreased significantly only in the OE group. The decrease in PTX3, hip circumference (HC), LDL, total cholesterol, triglycerides, and body fat percentage was not statistically significant in either training group, and there was a small, insignificant increase in flexibility. The details of the paired t-test and covariance results are presented in Table 4.
Measured Physical Fitness Factors in Pre- and Post-test a
Measured Variables and Stages | NOLTA (n = 10) | OE (n = 10) |
---|---|---|
Sit-ups (No.) | ||
Pre-test | 21.30 ± 5.96 | 20.80 ± 9.51 |
Post-test | 25.20 ± 6.16 | 24.60 ± 9.10 |
% Changes | 18 | 18 |
Push up (No.) | ||
Pre-test | 16.30 ± 6.63 | 15.90 ± 4.06 |
Post-test | 19.10 ± 4.67 | 17.80 ± 4.82 |
% Changes | 17 | 11 |
Flexibility (cm) | ||
Pre-test | 35.10 ± 4.84 | 25.80 ± 5.88 |
Post-test | 35.82 ± 3.73 | 27.30 ± 5.63 |
% Changes | 2 | 5 |
Strength of right hand (kg) | ||
Pre-test | 8.80 ± 2.28 | 5.65 ± 3.26 |
Post-test | 11.00 ± 3.46 | 7.40 ± 3.23 |
% Changes | 25 | 30 |
Strength of left hand (kg) | ||
Pre-test | 6.30 ± 2.64 | 4.60 ± 2.45 |
Post-test | 7.50 ± 2.67 | 5.60 ± 2.05 |
% Changes | 19 | 21 |
WC (cm) | ||
Pre-test | 74.15 ± 8.98 | 72.10 ± 11.29 |
Post-test | 72.60 ± 7.35 | 71.10 ± 10.75 |
% Changes | -2 | -1 |
HC (cm) | ||
Pre-test | 91.35 ± 11.32 | 81.50 ± 11.59 |
Post-test | 90.97 ± 10.65 | 81.65 ± 11.54 |
% Changes | -0.4 | 0.1 |
Measured Blood Factors in Pre- and Post-test a
Measured Variables and Stages | NOLTA (n = 10) | OE (n = 10) |
---|---|---|
Fasting blood glucose (mg/dL) | ||
Pre-test | 215.80 ± 66.05 | 244.70 ± 83.57 |
Post-test | 180.70 ± 52.33 | 214.50 ± 80.37 |
% Changes | -16 | -12 |
Hemoglobin A1C (mmol/mol) | ||
Pre-test | 75.70 ± 15.24 | 79.00 ± 21.84 |
Post-test | 72.40 ± 16.44 | 71.60 ± 19.21 |
% Changes | -4 | -9 |
PTX3 (pg/mL) | ||
Pre-test | 2.75 ± 0.84 | 2.60 ± 0.94 |
Post-test | 2.73 ± 0.87 | 2.59 ± 0.94 |
% Changes | -0.007 | -0.003 |
hs-CRP (mg/L) | ||
Pre-test | 3.50 ± 1.27 | 3.36 ± 0.89 |
Post-test | 2.39 ± 0.62 | 2.47 ± 0.68 |
% Changes | -31 | -26 |
Cholesterol total (mg/dL) | ||
Pre-test | 165.30 ± 22.41 | 153.90 ± 19.55 |
Post-test | 160.10 ± 22.24 | 150.30 ± 25.32 |
% Changes | -3 | -2 |
Triglycerides (mg/dL) | ||
Pre-test | 117.60 ± 38.50 | 92.70 ± 10.42 |
Post-test | 116.30 ± 44.20 | 92.40 ± 13.09 |
% Changes | -1 | -0.3 |
HDL (mg/dL) | ||
Pre-test | 46.80 ± 11.29 | 42.80 ± 8.76 |
Post-test | 57.00 ± 8.21 | 52.10 ± 9.51 |
% Changes | 21 | 21 |
LDL (mg/dL) | ||
Pre-test | 104.40 ± 31.59 | 87.00 ± 16.51 |
Post-test | 94.20 ± 18.43 | 81.60 ± 16.37 |
% Changes | -9 | -6 |
LDL to HDL ratio | ||
Pre-test | 2.39 ± 1.17 | 2.13 ± 0.69 |
Post-test | 1.69 ± 0.38 | 1.56 ± 0.217 |
% Changes | -29 | -26 |
Cholesterol to HDL ratio | ||
Pre-test | 3.73 ± 1.73 | 3.70 ± 0.73 |
Post-test | 2.88 ± 0.46 | 2.89 ± 0.26 |
% Changes | -22 | -21 |
The Results Related to the Statistical Analysis of Data
Measured Variables | t-test | ANCOVA | ||||
---|---|---|---|---|---|---|
NOLTA | OE | |||||
t | P-Value | t | P-Value | F | P-Value | |
Fasting blood glucose | 2.643 | 0.027a | 2.312 | 0.046a | 0.475 | 0.500 |
Hemoglobin A1C | 1.996 | 0.077 | 4.384 | 0.002a | 2.701 | 0.119 |
PTX3 | 1.410 | 0.192 | 1.561 | 0.153 | 1.366 | 0.259 |
hs-CRP | 2.64 | 0.027a | 4.11 | 0.003a | 0.151 | 0.702 |
Cholesterol total | 0.677 | 0.516 | 0.632 | 0.543 | 0.079 | 0.782 |
Triglycerides | 0.324 | 0.754 | 0.075 | 0.942 | 0.175 | 0.681 |
HDL | -2.88 | 0.018a | -3.906 | 0.004a | 0.750 | 0.398 |
LDL | 1.866 | 0.095 | 1.220 | 0.253 | 0.352 | 0.561 |
LDL to HDL ratio | 2.090 | 0.066 | 2.423 | 0.038a | 0.560 | 0.464 |
Cholesterol to HDL ratio | 2.406 | 0.040a | 3.162 | 0.012a | 0.002 | 0.963 |
VO2 peak | -4.411 | 0.002a | -2.841 | 0.019a | 6.29 | 0.023a |
Sit-ups | -3.074 | 0.013a | -6.626 | 0.001a | 0.012 | 0.916 |
Push up | -2.537 | 0.032a | -5.460 | 0.001a | 0.937 | 0.347 |
Flexibility | -0.717 | 0.491 | -2.098 | 0.065 | 0.881 | 0.361 |
Strength of right hand | -3.869 | 0.004a | -4.768 | 0.001a | 0.102 | 0.753 |
Strength of left hand | -1.935 | 0.085 | -2.582 | 0.030a | 0.826 | 0.376 |
WC | 2.140 | 0.061 | 3.354 | 0.008a | 0.272 | 0.609 |
HC | 1.004 | 0.342 | -1.152 | 0.279 | 0.244 | 0.628 |
BMI | -2.926 | 0.017a | -0.579 | 0.576 | 1.27 | 0.276 |
Body fat percentage | -1.057 | 0.318 | -0.870 | 0.407 | 0.091 | 0.767 |
5. Discussion
Our results showed a small and non-significant decrease in PTX3 levels in the training groups. The impact of sports training on PTX3 levels remains contradictory and limited. Zempo et al. reported an increase in PTX3 plasma levels in middle-aged individuals after eight weeks of aerobic exercise (16). In contrast, Estebanez et al. observed a decrease in PTX3 expression following eight weeks of aerobic training in the elderly (15). It is important to note that exercise training, both acute and chronic, is recognized as a significant modulator of PTX3, but the biological and molecular mechanisms underlying these changes have not yet been fully determined (15). Although the main mechanisms involved in the synthesis of PTX3 and the effects of physical exercise on this process are not completely elucidated, some of the elevation in PTX3 levels is attributed to muscle damage induced by chronic exercise training, which helps modulate immune responses (19). Various forms of exercise cause muscle cell damage, prompting rapid PTX3 production in the affected tissue. This increase in PTX3 levels, along with creatine kinase, myoglobin, and interleukins in the bloodstream, may indicate a tissue inflammation response involving adipocytes, smooth muscle, and skeletal muscle. Therefore, these findings suggest that the signaling mechanisms mediated by PTX3 may vary, and in muscle tissue, this protein plays a role in regulating the innate immune system (19, 20).
Our results show a significant decrease in hs-CRP levels in both exercise groups. Research indicates that exercise can significantly reduce hs-CRP levels in both healthy individuals and those with cardiovascular diseases. Epidemiological studies suggest that increased physical activity is associated with lower hs-CRP levels and a reduced risk of heart disease (21). A systematic review examining the effect of various exercises on CRP levels concluded that long-term and regular exercise lowers CRP levels by exerting an anti-inflammatory effect (14). Although several studies have reported that exercise interventions can reduce circulating hs-CRP, the mechanisms behind this effect and which groups benefit the most remain unclear (21). Comparisons of different subjects show no significant difference in the effect size of exercise programs with varying characteristics (duration, frequency, intensity) or in factors such as age, gender, and health status on CRP levels. There is general agreement that the greatest improvement in CRP levels is associated with reductions in BMI, body fat percentage, and body weight, and that combining diet with physical activity further decreases CRP levels (21, 22). Various studies have highlighted the benefits of exercise on inflammatory parameters and demonstrated its positive effects at different intensities (23). Our results contribute to the growing evidence that physical activity may reduce inflammation, a key process in the pathogenesis of cardiovascular diseases.
Since the increase in hs-CRP in children with T1DM is related to waist circumference (WC) (10), promoting a healthy weight and reducing abdominal fat can be effective in lowering hs-CRP levels in the subjects of this study. Our results also show improvements in strength in the hand, deltoid, and abdominal muscles, as well as enhanced cardiovascular fitness in the training groups. Previous studies have reported improvements in VO2 max, strength, WC, and resting heart rate in people with T1DM due to regular resistance and high-intensity exercises (24, 25).
It should be noted that diabetic children and adolescents require continuous insulin therapy to achieve optimal conditions. However, excessive insulin use is often associated with weight gain and abdominal obesity. Additionally, the fear of hypoglycemia can affect their lifestyle, leading to inactivity, decreased physical health, and obesity (26). Nevertheless, evidence supports the beneficial effects of exercise in managing blood sugar levels and mitigating the risk of hypoglycemia. Studies indicate that physical activity is an effective tool for preventing overweight/obesity, cardiovascular diseases, and improving physical fitness (27).
The available findings suggest that physical activity increases the ratio of AMP/ATP, which raises the levels of AMP-activated protein kinase (AMPK) (28). This protein acts as a protective sensor for the cell's energy status, and its activation promotes mitochondrial biogenesis, fatty acid oxidation, increased insulin sensitivity, and improved glucose absorption. These conditions contribute to reducing body fat, enhancing lipid profiles, and improving glucose metabolism (29). Additionally, scientific evidence shows that intramuscular AMP signaling and AMPK activity are directly related to exercise intensity (30). Therefore, increasing the intensity of training can maximize the benefits of physical activity in improving body composition, lipid profiles, and glucose metabolism.
Our results show a significant increase in HDL and a decrease in LDL/HDL and Cholesterol/HDL ratios in the exercise groups. Additionally, HbA1c and fasting blood glucose levels decreased significantly. While there is limited information specifically about people with T1DM, studies across different populations consistently show significant benefits of regular, low-to-moderate-intensity physical activities on blood glucose regulation and lipid profile. Most studies report improvements in insulin sensitivity and reductions in insulin dosage following various sports activities (13).
In terms of lipid profile, a key finding in our study is the significant increase in HDL levels in both training groups. Lipid metabolism involves several interdependent pathways, including the hepatic synthesis of very low-density lipoprotein (VLDL), fatty acid absorption by skeletal muscles or adipose tissue, extrahepatic cholesterol transport by low-density lipoproteins, and excess cholesterol removal by HDL (31). High-density lipoprotein levels and the incidence of cardiovascular disease (CVD) are inversely related. High-density lipoprotein levels plays a crucial role in reverse cholesterol transport (RCT), which removes excess cholesterol from peripheral tissues and delivers it to the liver or bile for excretion (32). Evidence indicates that regular exercise enhances lipid metabolism by increasing HDL levels and RCT activity (31).
Regarding blood sugar control, Yardley et al. found that strength training, either alone or combined with aerobic exercises, can significantly reduce HbA1c levels. However, some researchers have not observed positive effects with other forms of exercise (33). Our results also showed that despite minor changes in HbA1c in the NOLTA group, resistance training led to a significant reduction in HbA1c.
Guidelines on the appropriate type, duration, and intensity of exercise are regularly updated by organizations such as the American Diabetes Association and the International Society for Pediatric and Adolescent Diabetes. Exercise for patients with T1DM should not only encourage increased activity but also maximize health benefits.
Our results indicate that for children with T1DM, regularly organized exercise and leisure activities can improve lipid profiles, physical fitness, body composition, and glycemic control, and may also effectively reduce levels of inflammatory factors. Therefore, regular exercise is an important component in the management of T1DM, contributing to the prevention and reduction of cardiovascular disease risk factors in diabetic children and adolescents.
The main limitations of this research include the small sample size, the absence of a control group, the short duration of the exercise intervention, and the lack of precise dietary control. Future researchers should consider these limitations when using the results of this study for further investigation.
References
-
1.
Wang W, Liu H, Xiao S, Liu S, Li X, Yu P. Effects of insulin plus glucagon-like peptide-1 receptor agonists (GLP-1RAs) in treating type 1 diabetes mellitus: A systematic review and meta-analysis. Diabetes Ther. 2017;8(4):727-38. [PubMed ID: 28616805]. [PubMed Central ID: PMC5544618]. https://doi.org/10.1007/s13300-017-0282-3.
-
2.
MacKenzie KE, Wiltshire EJ, Pena AS, Gent R, Hirte C, Piotto L, et al. Hs-CRP is associated with weight, BMI, and female sex but not with endothelial function in children with type 1 diabetes. Pediatr Diabetes. 2009;10(1):44-51. [PubMed ID: 18798827]. https://doi.org/10.1111/j.1399-5448.2008.00456.x.
-
3.
Casula M, Montecucco F, Bonaventura A, Liberale L, Vecchie A, Dallegri F, et al. Update on the role of Pentraxin 3 in atherosclerosis and cardiovascular diseases. Vascul Pharmacol. 2017;99:1-12. [PubMed ID: 29051088]. https://doi.org/10.1016/j.vph.2017.10.003.
-
4.
Gruszka W, Wyskida K, Chudek J, Olszanecka-Glinianowicz M. Pentraxin 3-a potential link between inflammation, obesity and cardiovascular complications in polycystic ovary syndrome. Advances in Hygiene & Experimental Medicine/Postepy Higieny i Medycyny Doswiadczalnej. 2018;72. https://doi.org/10.5604/01.3001.0012.2025.
-
5.
Inforzato A, Reading PC, Barbati E, Bottazzi B, Garlanda C, Mantovani A. The "sweet" side of a long pentraxin: How glycosylation affects PTX3 functions in innate immunity and inflammation. Front Immunol. 2012;3:407. [PubMed ID: 23316195]. [PubMed Central ID: PMC3539679]. https://doi.org/10.3389/fimmu.2012.00407.
-
6.
Todoric J, Handisurya A, Knapp B, Tura A, Pacini G, Kautzky-Willer A. Relationship of pentraxin 3 with insulin sensitivity in gestational diabetes. Eur J Clin Invest. 2013;43(4):341-9. [PubMed ID: 23379644]. https://doi.org/10.1111/eci.12051.
-
7.
Katakami N, Kaneto H, Sakamoto F, Takahara M, Irie Y, Fujisawa K, et al. Plasma pentraxin 3 levels are associated with carotid IMT in type 1 diabetic patients. Diabetes Res Clin Pract. 2013;99(2):185-91. [PubMed ID: 23245734]. https://doi.org/10.1016/j.diabres.2012.11.020.
-
8.
Li B, Tian X, Guo S, Zhang M, Li J, Zhai N, et al. Pentraxin-3 and adropin as inflammatory markers of early renal damage in type 2 diabetes patients. Int Urol Nephrol. 2020;52(11):2145-52. [PubMed ID: 32661627]. https://doi.org/10.1007/s11255-020-02568-x.
-
9.
Devaraj S, Cheung AT, Jialal I, Griffen SC, Nguyen D, Glaser N, et al. Evidence of increased inflammation and microcirculatory abnormalities in patients with type 1 diabetes and their role in microvascular complications. Diabetes. 2007;56(11):2790-6. [PubMed ID: 17686944]. [PubMed Central ID: PMC2692935]. https://doi.org/10.2337/db07-0784.
-
10.
Larsson SC, Wallin A, Hakansson N, Stackelberg O, Back M, Wolk A. Type 1 and type 2 diabetes mellitus and incidence of seven cardiovascular diseases. Int J Cardiol. 2018;262:66-70. [PubMed ID: 29605469]. https://doi.org/10.1016/j.ijcard.2018.03.099.
-
11.
Maahs DM, Wadwa RP, Bishop F, Daniels SR, Rewers M, Klingensmith GJ. Dyslipidemia in youth with diabetes: To treat or not to treat? J Pediatr. 2008;153(4):458-65. [PubMed ID: 18847618]. [PubMed Central ID: PMC2585025]. https://doi.org/10.1016/j.jpeds.2008.05.062.
-
12.
Dimitri P, Joshi K, Jones N, Moving Medicine for Children Working G. Moving more: physical activity and its positive effects on long term conditions in children and young people. Arch Dis Child. 2020;105(11):1035-40. [PubMed ID: 32198161]. https://doi.org/10.1136/archdischild-2019-318017.
-
13.
Aljawarneh YM, Wardell DW, Wood GL, Rozmus CL. A systematic review of physical activity and exercise on physiological and biochemical outcomes in children and adolescents with type 1 diabetes. J Nurs Scholarsh. 2019;51(3):337-45. [PubMed ID: 30895735]. https://doi.org/10.1111/jnu.12472.
-
14.
Kasapis C, Thompson PD. The effects of physical activity on serum C-reactive protein and inflammatory markers: A systematic review. J Am Coll Cardiol. 2005;45(10):1563-9. [PubMed ID: 15893167]. https://doi.org/10.1016/j.jacc.2004.12.077.
-
15.
Estebanez B, Rodriguez AL, Visavadiya NP, Whitehurst M, Cuevas MJ, Gonzalez-Gallego J, et al. Aerobic training down-regulates pentraxin 3 and pentraxin 3/toll-like receptor 4 ratio, irrespective of oxidative stress response, in elderly subjects. Antioxidants (Basel). 2020;9(2). [PubMed ID: 32012711]. [PubMed Central ID: PMC7070734]. https://doi.org/10.3390/antiox9020110.
-
16.
Zempo-Miyaki A, Fujie S, Sato K, Hasegawa N, Sanada K, Maeda S, et al. Elevated pentraxin 3 level at the early stage of exercise training is associated with reduction of arterial stiffness in middle-aged and older adults. J Hum Hypertens. 2016;30(9):521-6. [PubMed ID: 26467819]. https://doi.org/10.1038/jhh.2015.105.
-
17.
Wu N, Bredin SSD, Guan Y, Dickinson K, Kim DD, Chua Z, et al. Cardiovascular health benefits of exercise training in persons living with type 1 diabetes: A systematic review and meta-analysis. J Clin Med. 2019;8(2). [PubMed ID: 30781593]. [PubMed Central ID: PMC6406966]. https://doi.org/10.3390/jcm8020253.
-
18.
Matsuzaka A, Takahashi Y, Yamazoe M, Kumakura N, Ikeda A, Wilk B, et al. Validity of the multistage 20-m shuttle-run test for Japanese children, adolescents, and adults. Pediatric exercise science. 2004;16(2):113-25. https://doi.org/10.1123/pes.16.2.113.
-
19.
Tunc-Ata M, Turgut G, Mergen-Dalyanoglu M, Turgut S. Examination of levels pentraxin-3, interleukin-6, and C-reactive protein in rat model acute and chronic exercise. J Exerc Rehabil. 2017;13(3):279-83. [PubMed ID: 28702438]. [PubMed Central ID: PMC5498083]. https://doi.org/10.12965/jer.1734920.490.
-
20.
Nakajima T, Kurano M, Hasegawa T, Takano H, Iida H, Yasuda T, et al. Pentraxin3 and high-sensitive C-reactive protein are independent inflammatory markers released during high-intensity exercise. Eur J Appl Physiol. 2010;110(5):905-13. [PubMed ID: 20640440]. https://doi.org/10.1007/s00421-010-1572-x.
-
21.
Hammonds TL, Gathright EC, Goldstein CM, Penn MS, Hughes JW. Effects of exercise on c-reactive protein in healthy patients and in patients with heart disease: A meta-analysis. Heart Lung. 2016;45(3):273-82. [PubMed ID: 26916454]. [PubMed Central ID: PMC5873310]. https://doi.org/10.1016/j.hrtlng.2016.01.009.
-
22.
Fedewa MV, Hathaway ED, Ward-Ritacco CL. Effect of exercise training on C reactive protein: A systematic review and meta-analysis of randomised and non-randomised controlled trials. Br J Sports Med. 2017;51(8):670-6. [PubMed ID: 27445361]. https://doi.org/10.1136/bjsports-2016-095999.
-
23.
Wu N, Bredin SSD, Jamnik VK, Koehle MS, Guan Y, Shellington EM, et al. Association between physical activity level and cardiovascular risk factors in adolescents living with type 1 diabetes mellitus: A cross-sectional study. Cardiovasc Diabetol. 2021;20(1):62. [PubMed ID: 33712025]. [PubMed Central ID: PMC7955612]. https://doi.org/10.1186/s12933-021-01255-0.
-
24.
Zinn S, Nelis P, Minnebeck K, Hinder J, Eter N, Brand SM, et al. Effect of high-intensity interval training in patients with type 1 diabetes on physical fitness and retinal microvascular perfusion determined by optical coherence tomography angiography. Microvasc Res. 2020;132:104057. [PubMed ID: 32795467]. https://doi.org/10.1016/j.mvr.2020.104057.
-
25.
Absil H, Baudet L, Robert A, Lysy PA. Benefits of physical activity in children and adolescents with type 1 diabetes: A systematic review. Diabetes Res Clin Pract. 2019;156:107810. [PubMed ID: 31401153]. https://doi.org/10.1016/j.diabres.2019.107810.
-
26.
Calella P, Vitucci D, Zanfardino A, Cozzolino F, Terracciano A, Zanfardino F, et al. Lifestyle and physical fitness in adolescents with type 1 diabetes and obesity. Heliyon. 2023;9(1). e13109. [PubMed ID: 36711296]. [PubMed Central ID: PMC9880399]. https://doi.org/10.1016/j.heliyon.2023.e13109.
-
27.
Chimen M, Kennedy A, Nirantharakumar K, Pang TT, Andrews R, Narendran P. What are the health benefits of physical activity in type 1 diabetes mellitus? A literature review. Diabetologia. 2012;55(3):542-51. [PubMed ID: 22189486]. https://doi.org/10.1007/s00125-011-2403-2.
-
28.
Richter EA, Ruderman NB. AMPK and the biochemistry of exercise: Implications for human health and disease. Biochem J. 2009;418(2):261-75. [PubMed ID: 19196246]. [PubMed Central ID: PMC2779044]. https://doi.org/10.1042/BJ20082055.
-
29.
O'Neill HM. AMPK and Exercise: Glucose Uptake and Insulin Sensitivity. Diabetes Metab J. 2013;37(1):1-21. [PubMed ID: 23441028]. [PubMed Central ID: PMC3579147]. https://doi.org/10.4093/dmj.2013.37.1.1.
-
30.
Wadley GD, Lee-Young RS, Canny BJ, Wasuntarawat C, Chen ZP, Hargreaves M, et al. Effect of exercise intensity and hypoxia on skeletal muscle AMPK signaling and substrate metabolism in humans. Am J Physiol Endocrinol Metab. 2006;290(4):E694-702. [PubMed ID: 16263768]. https://doi.org/10.1152/ajpendo.00464.2005.
-
31.
Marques LR, Diniz TA, Antunes BM, Rossi FE, Caperuto EC, Lira FS, et al. Reverse Cholesterol Transport: Molecular Mechanisms and the Non-medical Approach to Enhance HDL Cholesterol. Front Physiol. 2018;9:526. [PubMed ID: 29867567]. [PubMed Central ID: PMC5962737]. https://doi.org/10.3389/fphys.2018.00526.
-
32.
Chapman MJ, Le Goff W, Guerin M, Kontush A. Cholesteryl ester transfer protein: At the heart of the action of lipid-modulating therapy with statins, fibrates, niacin, and cholesteryl ester transfer protein inhibitors. Eur Heart J. 2010;31(2):149-64. [PubMed ID: 19825813]. [PubMed Central ID: PMC2806550]. https://doi.org/10.1093/eurheartj/ehp399.
-
33.
Yardley JE, Sigal RJ, Perkins BA, Riddell MC, Kenny GP. Resistance exercise in type 1 diabetes. Can J Diabetes. 2013;37(6):420-6. [PubMed ID: 24321724]. https://doi.org/10.1016/j.jcjd.2013.07.020.