Abstract
Context:
The correlation between neuroinflammation and neurodegenerative disorders has been extensively documented. Elevated levels of inflammatory cytokines in the bloodstream have been demonstrated to impair memory function and heighten susceptibility to neurodegenerative disorders. Furthermore, elevated quantities of reactive oxygen species (ROS) in the body, known as oxidative stress, exacerbate neurodegenerative illnesses and negatively affect learning and memory. Neuroprotection prevents neuronal cell death by intervening and blocking the pathogenetic process that leads to cellular malfunction and death.Methods:
We evaluated several studies in the WEB of SCIENCE, SCOPUS, and PubMed. Furthermore, we identified the central genes and signaling pathways associated with neurogenesis, the neural system, and neuroplasticity through data mining, a literature review of artificial intelligence, and an in-silico study.Results:
Physical exercise (PE) benefits various physiological systems, including the central nervous system. The beneficial impacts of physical activity on cognitive performance, neural well-being, and safeguarding neurons against different brain injuries are extensively documented. Furthermore, research has demonstrated that PE is a powerful non-pharmacological intervention that enhances cognitive function, including learning and memory, while decreasing the likelihood of developing neurodegenerative disorders. Additionally, engaging in moderate physical activity that does not result in extreme fatigue has a beneficial impact on reducing inflammation and promoting antioxidant effects. According to the hormesis theory, physical inactivity and extreme overtraining can decrease physiological function.Conclusions:
In summary, a combination of moderate aerobic exercise, HIIT, and resistance training, performed at appropriate intensities, is most beneficial for neuroprotection and cognitive health. Regular engagement in these activities can help mitigate the risk of neurodegenerative diseases and enhance overall brain function.Keywords
Pathomechanisms Signaling Pathways Neuroinflammation Exercise Training Athletics
1. Context
Neuroprotection is a fundamental concept in neuroscience that focuses on the strategic prevention of neuronal cell death. This is achieved by intervening in and inhibiting the pathogenic processes that contribute to cellular dysfunction and eventual cell death (1). This paradigm has attracted significant attention from the scientific community, promoting the investigation of innovative therapeutic strategies aimed at preserving brain tissue and improving overall clinical outcomes (1, 2). Importantly, advanced age is recognized as the primary risk factor for most neurodegenerative diseases, including Alzheimer's disease (AD) and Parkinson's disease (PD), especially in the elderly population (3).
In the United States, the prevalence of AD in individuals aged 95 years and older is approximately 50%, highlighting the urgent need to tackle neurodegenerative challenges within the aging population. Likewise, PD affects 2 - 3% of those aged 65 years and older, reinforcing the importance of addressing age-related neurodegenerative disorders (4). Epidemiological studies have revealed a significant association between physical activity and a decreased risk of AD and dementia. Participation in physical activities is linked to a substantial risk reduction of 45% for AD and 28% for dementia (5). This highlights the potential of lifestyle interventions, particularly exercise, to promote neuroprotection.
The complex relationship between neuroinflammation and neurodegenerative diseases is well-established. The overproduction of inflammatory cytokines, such as interleukin-1β (IL-1β), tumor necrosis factor-α (TNF-α), and interleukin-6 (IL-6) in the brain, along with heightened levels of C-reactive protein (CRP) in plasma, has been associated with memory impairment and an elevated risk of AD (6). Moreover, oxidative stress, marked by increased levels of ROS, is recognized as a crucial factor in neurodegenerative diseases, contributing to deficits in learning and memory (6, 7). An in-depth understanding of these complex mechanisms lays the groundwork for creating targeted interventions designed to alleviate the effects of neurodegenerative diseases, especially within the aging population (8).
This discourse underscores the multifaceted nature of neuroprotection, integrating age-related risk factors, the potential of lifestyle modifications, and the intricate molecular pathways involving neuroinflammation and oxidative stress (9). These considerations serve as the foundation for future research efforts and therapeutic advancements in the pursuit of effective strategies against neurodegenerative diseases (10, 11). Contemporary research reveals that the adult mammalian brain remains dynamically influenced by external stimuli, as demonstrated in human and experimental animal studies (12). Neuroplasticity, a term encompassing the nervous system's potential to modify its structure in response to changes in expected and environmental conditions, has become a focal point of investigation (13).
This phenomenon is observed under various circumstances, including the acquisition of novel strategies, recovery from nervous system injury, or in the absence of sensory stimulation (13). Neuroplasticity is evident at multiple levels of the nervous system, encompassing ion channels, synapses, neurons, neuronal columns, cortical organization, and modes of operation (14). These layers exhibit a complex interconnection and interdependence. Exploring neuroplasticity at various levels of the nervous system broadens our understanding of its intricacies and underscores the dynamic and adaptive nature of the adult mammalian brain in response to diverse stimuli and experiences (15).
Considering the crucial significance of neuroplasticity in adapting to various demands and situations, the primary focus lies in elucidating methods to enhance neuroplastic processes in order to foster learning and memory, prevent cognitive decline throughout life, and facilitate recovery after brain injuries (16). Recent investigations suggest that physical activity and exercise training are viable avenues for enhancing neuroplasticity, thereby contributing to improved cognitive performance, as substantiated by studies involving both human subjects and animals (2, 16). Physical exercise (PE), in particular, has emerged as a potent stimulator of neuroplasticity, potentially fostering positive adaptations in learning and memory (17).
However, it is essential to note that the impact of physical activity extends beyond favorable cognitive outcomes, encompassing its potential to influence negative forms of learning, such as the acquisition of fear or undesirable behaviors (18). Notably, PE has garnered attention in disease modification, with previous research highlighting its promise as a non-pharmacological therapeutic option for various conditions, including neurological, metabolic, mental, and cardiovascular diseases (19).
These findings highlight the diverse benefits of integrating PE into health strategies, indicating its potential for cognitive enhancement and addressing wider health issues (20). As research in this area advances, a deeper understanding of how physical activity affects neuroplasticity provides essential insights for creating targeted interventions aimed at fostering cognitive resilience and enhancing overall well-being throughout the lifespan (21).
This research focuses on neuroprotection and neuroplasticity, particularly in the context of neurodegenerative diseases. Key questions include identifying mechanisms of neuroprotection to prevent neuronal cell death, understanding the risk factors associated with neurodegenerative diseases, and evaluating the impact of physical activity on cognitive decline. Additionally, the research aims to explore the roles of neuroinflammation and oxidative stress in cognitive impairment and assess how exercise can enhance neuroplasticity. The overarching objective is to develop targeted interventions that promote cognitive health and resilience.
2. Methods
2.1. Data Mining and Literature Search
The WEB OF SCIENCE, SCOPUS, and PubMed databases were utilized for data mining and literature search. This study was performed by cross-checking the keywords based on Medical Subjects Headings (MeSH) terms: “Physical exercise,” “exercise training,” “moderate exercise,” “overtraining,” “aerobic training,” “neuroplasticity,” “cognition,” “brain development,” “hippocampal neurogenesis,” “learning,” and “memory.”
Original animal and human studies were included, specifically those that evaluated the role of exercise training and physical activity in regulating neuroinflammation and neurodegenerative diseases.
2.2. Bioinformatic Analysis
To examine the impact of physical mobility on neuronal destruction in dementia, we gathered the related microarray dataset, GSE110298, which compares sedentary individuals with AD to Alzheimer's patients with high mobility throughout their lifetime. The GSE110298 dataset was analyzed using the Bioconductor package in the R programming language, with a significance level of P-value < .05 and logFC ± 2. Using the Cytoscape 3.6.0 software, we implemented visualizer settings, betweenness, and closeness to visually represent essential proteins in the genetic network.
3. Results
3.1. Master Gene Network Analysis Highlights Molecular Pathways Impacted by Sedentary Behavior in Neurodegenerative Disease
Estimating network characteristics related to the impact of mobility on neuronal damage in dementia conditions identified 242 regulatory genes implicated in the development of dementia in a sedentary lifestyle. The protein-protein interactions (PPIs) network was established by 62 hub genes that are specifically engaged in the physical training process. Exploration of master gene networks, elucidating key molecular signaling pathways implicated in dementia, was conducted using the Enrich-R algorithm (Figure 1A and B).
The association between protein protein intraction related to neurogenesis and physical activity. A, the hub genes associated with neurogenesis and exercise training. Based on the in-silico analysis and liturated reiwe exercise training could medite these hub genes; B, the enrichment analysis indicated that exercise training regulate the Neuroactive, Neurotrasnmiter, Neuronal system.
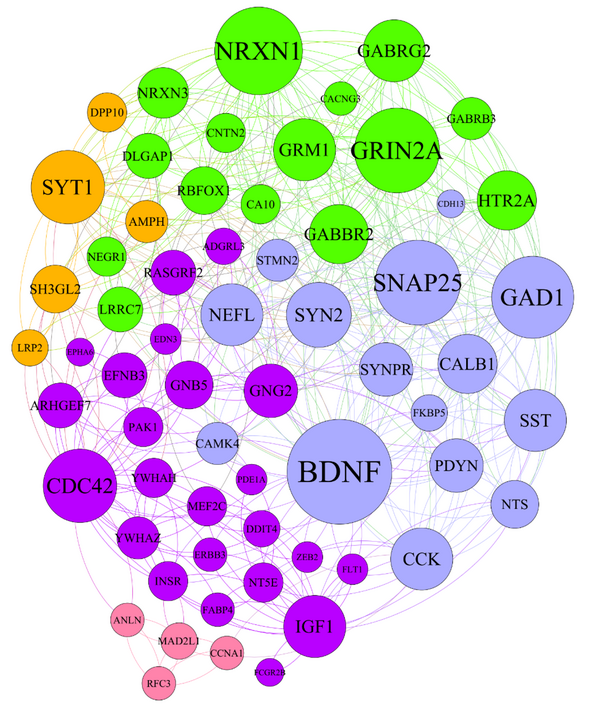
3.2. The Role of Physical Exercise and the Nervous System's Response
Physical exercise constitutes a fundamental component within the framework of a healthy lifestyle (22, 23). Distinguished by its organized, systematic, and repetitive nature, PE is designed to enhance various facets of physical fitness, efficiency, and overall well-being. Prescriptions for PE often encompass specific parameters, including the type of activity, its intensity, frequency, and duration. Diverse training methodologies have been devised to augment cardiorespiratory fitness, musculoskeletal function, and metabolic activity. Among the prevalent exercise training methods are aerobic, endurance, and resistance (strength exercise) regimens (24).
Aerobic exercise, also known as moderate-intensity continuous training (MICT), involves activities such as walking, cycling, running, and swimming at a moderate effort level for extended durations, typically lasting at least 30 minutes (25). While the health benefits of MICT are extensively researched and established in humans, a prevalent impediment to the consistent practice of aerobic exercise is the perceived time constraints faced by the global population (26, 27). Addressing this challenge is pivotal for promoting widespread engagement in aerobic exercise and reaping its associated health advantages.
To address this issue, researchers and fitness experts have focused on investigating the physiological effects of concise exercise sessions to optimize time efficiency, exemplified by high-intensity interval training (HIIT). High-intensity interval training consists of brief bouts of intense exercise interspersed with rest or low-intensity recovery periods. Typically conducted within a session lasting 30 minutes or less, inclusive of warm-up and cool-down phases, HIIT has garnered attention for its potential benefits (26, 28, 29).
The hallmark of HIIT involves engaging in high-intensity intervals approaching maximum effort, reaching intensities between 80 to 100% of the maximal heart rate (HRmax) (30). These high-intensity intervals typically last for a maximum of 60 seconds, followed by recovery periods of up to 4 minutes, encompassing low-intensity exercise or rest. Despite variations in the durations of high- and low-exercise bouts documented in studies (31), all HIIT sessions are consistently confined to 30 minutes or less.
Exploration into the central nervous system's response to HIIT has revealed significant findings in research targeting both the spinal cord (32) and the brain (33, 34). Notably, a 16-week HIIT program demonstrated elevated oxygen consumption and brain oxygenation among older individuals compared to MICT (33). Similar findings were observed in younger adults as well (35). The brain-derived neurotrophic factor (BDNF) response in these studies appeared contingent upon the intensity of the exercise. However, the underlying chemical processes governing these brain adaptations to HIIT remain not fully elucidated. Further research is essential to deepen our comprehension of these mechanisms and their implications for optimizing neurophysiological benefits through HIIT (35).
Researchers and fitness experts have focused on investigating the physiological effects of concise exercise sessions to optimize time efficiency, exemplified by the approach of HIIT. High-intensity interval training, an acronym for HIIT, entails brief bouts of intense exercise interspersed with rest or low-intensity recovery periods. Typically conducted within a session lasting 30 minutes or less, inclusive of warm-up and cool-down phases, HIIT has garnered attention for its potential benefits (26).
The relationship between physical activity and brain function has also gained recent attention, with prior studies primarily focusing on the effects of low to moderate-intensity aerobic exercise. Physical activity emerges as a potent stimulant for neuroplasticity, facilitating the growth of new neurons and enhancing mental well-being. Engaging in regular exercise has been associated with the alleviation of symptoms related to sadness and anxiety, as well as improvements in mood and stress management. Moreover, exercise has demonstrated its efficacy in enhancing hippocampal memory functions, particularly in visuospatial memory tasks (2, 19).
The observed health benefits are partly attributed to the impact of physical activity on the expression or elevation of neurotrophins, pivotal molecules that enhance neuronal structure in the brain. The augmentation of neurotrophin levels, notably BDNF, correlates with improved neuronal structure (36). Endurance training has been shown to elevate BDNF expression, resulting in increased dendritic spine density and dendritic arborization. These structural modifications have been linked to favorable enhancements in memory and learning outcomes (37).
Furthermore, exercise substantially influences brain neurogenesis, with different training methods leading to varied physiological changes, thereby influencing brain plasticity (38). The elaborate interplay between physical activity and neurological adaptations underscores the multifaceted nature of the benefits conferred by exercise, not only on mental well-being but also on the structural and functional aspects of the brain. Continued exploration in this field holds promise for uncovering nuanced mechanisms and refining exercise prescriptions to optimize cognitive and emotional outcomes (38).
The positive impacts of PE extend across various physiological systems, with notable benefits observed in the central nervous system. Extensive documentation underscores the advantageous effects of physical activity on cognitive performance, neural health, and the safeguarding of neurons against brain damage (39). As a potent non-pharmacological therapy, PE has demonstrated its efficacy in enhancing learning and memory while mitigating the risk of developing AD (40).
Consistent engagement in physical activity has been associated with a reduction in amyloid oligomer levels, a pivotal factor in the pathogenesis of AD. Specifically, moderate exercise, which avoids inducing fatigue, exerts both anti-inflammatory and antioxidant effects. This moderation in exercise has been linked to reducing inflammatory cytokines, including TNF-α, IL-1β, IL-6, and CRP, alongside an augmentation of antioxidant activity to counteract oxidative damage (19, 40). The impact of various forms of exercise on brain plasticity is nuanced and contingent on factors such as intensity, timing, the style of workouts, and the species and age of the individual or animal. These findings underscore the versatility of PE as a holistic approach, not only for cognitive enhancement but also for promoting neural resilience and mitigating the risk of neurodegenerative conditions (41).
The hormesis hypothesis posits that both insufficient physical stress (inactivity) and excessive stress (overtraining) can lead to diminished physiological performance. Intense exercise without adequate recovery, or when compounded with other life stressors, may result in overreaching, eventually leading to overtraining syndrome. Overtraining is characterized by pronounced fatigue and reduced performance, often persisting for extended periods ranging from months to several years. This state disrupts the equilibrium of various hormonal, metabolic, and immunologic variables (42).
The impacts of excessive exercise without adequate rest, often associated with overtraining, extend to tissue damage, particularly in muscles and joints, triggering the production of trauma-related cytokines (43). These cytokines, in turn, stimulate circulating monocytes to produce inflammatory cytokines. Studies indicate that high-intensity exercise elevates TNF-α, IL-1β, IL-6, and CRP levels (44). Furthermore, acute exercise and overtraining have been associated with generating reactive oxygen and nitrogen species, leading to oxidative stress. However, when appropriately managed, regular exercise can activate the antioxidant system, providing protection against oxidative damage (45).
Excessive exercise is linked to potential adverse effects such as cognitive impairment, compromised immune systems, and heightened inflammation. While past research has consistently demonstrated the positive impact of physical activity on memory and cognitive functions, the nuanced effects of the type and intensity of exercise on memory, and the potential involvement of mechanisms like inflammation and immune system imbalance, have been subjects of investigation. Nevertheless, the specific impact of excessive exercise on brain inflammation and cognitive function in athletes remains uncertain and warrants further exploration (46).
3.3. The Role of Exercise in the Dopaminergic, Serotonergic, and Noradrenergic Systems
Physical activity significantly impacts the central dopaminergic, noradrenergic, and serotonergic systems, revealing important findings related to neurotransmitter regulation. Studies using homogenized tissues show notable changes in the production and breakdown of monoamines and neurotransmitters during physical activity. Methods such as micro-dialysis and voltammetry highlight how exercise training influences the localized release of neurotransmitters. Specifically, the release of monoamine neurotransmitters such as serotonin (5-HT), dopamine (DA), and noradrenaline (NA) is modulated during exercise (47).
Exercise training also affects extracellular levels of DA, NA, serotonin, γ-amino butyric acid (GABA), and glutamate (GLU). Increased DA levels in the brain during exercise elevate blood calcium levels, influencing DA production via the activation of tyrosine hydroxylase, an enzyme that depends on calcium/calmodulin (48). Exercise training enhances the binding affinity between DA and its receptor, measured by [3H] spiroperidol binding, which indicates a stronger interaction between these molecules. Moreover, exercise triggers a neurophysiological response that leads to adaptations to stress by activating a protective mechanism involving galanin production in the locus coeruleus. This mechanism hyperpolarizes noradrenergic neurons, suppressing the release of norepinephrine (NE) and thereby reducing anxious behavior. Norepinephrine is crucial in memory consolidation and retrieval, acting on the amygdala and frontal brain (48). Chronic and wheel exercise training have shown elevated NE levels in the spinal cord pons-medulla, further underscoring the complex interaction between exercise and neurotransmitter systems. This increase in natural NE activity suggests a connection between NE and the cognitive improvements linked to physical activity. This section emphasizes the intricate relationship between PE and neurochemistry, highlighting the potential benefits for stress management and cognitive abilities (49).
3.4. Impact of Different Types of Physical Activity
Resistance exercise (RE), characterized by muscle contractions against external resistance, is recommended by the World Health Organization as a beneficial exercise modality for older adults (50). Resistance exercise effectively enhances muscle mass, strength, bone density, and overall body power. It also improves functional capacity and balance, mitigating or even reversing sarcopenia and age-related declines in physical function (51). Beyond these physical benefits, RE holds potential for enhancing cognitive function and memory, particularly in older adults and individuals with AD (52). Resistance exercise exerts neuroprotective effects by increasing the release of neurotrophic factors, promoting neurogenesis, modulating inflammatory responses, and reducing amyloid-beta (Aβ) burden in individuals with AD.
Preclinical studies using animal models of AD consistently demonstrate cognitive benefits from RE. Liu et al. reported that RE improved cognitive function in a mouse model of AD by reducing Aβ burden and tau pathology while attenuating neuroinflammation. This neuroprotective effect is attributed to RE’s ability to upregulate presynaptic structural proteins crucial for vesicle recycling, enhancing synaptic transmission (53). Similarly, Pena et al. demonstrated that RE-induced neuroprotective mechanisms reduced Aβ accumulation in the hippocampus, improving learning and memory (54). Ozbeyli et al. suggest that RE-mediated enhancement of working memory may be linked to modulation of oxidative stress pathways and the antioxidant system (55).
Further supporting these findings, Jafarzadeh et al. (2021) used a rat model of streptozotocin-induced cognitive impairment and observed that RE improved learning and memory, along with increased expression of neurotrophic factors and their receptors (56). These preclinical studies provide compelling evidence that RE, through various mechanisms, confers significant cognitive benefits in AD models, corroborating findings from clinical studies involving patients with mild cognitive impairment (MCI) (57).
Brain-derived neurotrophic factor and nerve growth factor (NGF) play crucial roles in neuronal survival and plasticity, making them key targets for investigation in AD, a disease characterized by neuronal loss and synaptic dysfunction. In vitro studies demonstrate that BDNF and NGF enhance the viability of neuronal cultures exposed to Aβ peptides (58). Conversely, reduced BDNF signaling and impaired NGF signaling via TrkA receptors have been linked to elevated levels of Aβ and tau, suggesting a role for these neurotrophins in amyloid precursor protein (APP) processing (58).
In vivo studies further support the neuroprotective effects of BDNF and NGF. Low BDNF levels are associated with cognitive impairments, including deficits in memory and learning (59). Conversely, increasing BDNF levels in AD animal models has been shown to mitigate neuronal loss and improve cognitive performance (60). However, not all studies consistently demonstrate a direct impact of BDNF and NGF on Aβ plaque or neurofibrillary tangle formation (52, 61-63), which may be due to variations in animal models and study methodologies.
Regular PE exerts multifaceted neuroprotective effects, stimulating the synthesis of insulin-like growth factor 1 (IGF-1), mediating hippocampal neurogenesis, and promoting overall neuronal health (64, 65). Notably, RE has been shown to effectively elevate IGF-1 levels in the brains of individuals with MCI, a population characterized by low IGF-1 levels (66, 67). This increase in IGF-1 enhances synaptic plasticity, neuronal survival, and cognitive performance, thus ameliorating AD pathophysiology. Furthermore, RE’s benefits extend beyond direct effects on IGF-1. By enhancing muscle strength, RE indirectly elevates both IGF-1 and BDNF levels, which promotes neurogenesis and improves insulin sensitivity (68).
Preclinical studies using animal models of AD also demonstrate that RE enhances IGF-1 signaling while concurrently reducing APP mRNA and Aβ levels (69). These findings underscore the potent influence of RE on modulating key neurotrophic factors, highlighting its potential as a protective strategy against AD pathology by mitigating Aβ-mediated toxicity in the brain (70).
3.5. Exercise as a Potent Modulator of Neuroinflammation and Neurodegeneration
Exercise, particularly aerobic exercise and HIIT, has emerged as a powerful modulator of neuroinflammation and neurodegeneration (71). These exercise modalities benefit the brain by modulating key signaling pathways involved in neuronal survival, plasticity, and inflammatory responses (72). Specifically, exercise stimulates the expression of BDNF, a crucial molecule for neuronal growth and survival, and peroxisome proliferator-activated receptor gamma coactivator 1-alpha (PGC-1α), a master regulator of mitochondrial biogenesis and antioxidant defense (73). Conversely, exercise effectively reduces the activation of the nuclear factor kappa-light-chain-enhancer of activated B cells (NF-κB), a central mediator of inflammation (74).
It is important to note that different exercise types, including variations in intensity and duration, may differentially impact these signaling pathways and exert distinct effects on neuroinflammation and neurodegeneration (75). Understanding the intricate interplay between exercise modalities and these molecular pathways is crucial for developing targeted interventions to mitigate neuroinflammation and promote brain health (76). While all types of exercise appear beneficial, emerging evidence suggests that the type and intensity of exercise differentially influence neuroinflammation and neurodegeneration via distinct molecular pathways (71).
For instance, aerobic exercise robustly increases circulating levels of BDNF, which promotes neuronal survival and synaptic plasticity. This increase in BDNF is often accompanied by enhanced PGC-1α expression, a key player in mitochondrial biogenesis and antioxidant defense (73). In comparison, HIIT, while also promoting BDNF and PGC-1α, may exert more potent anti-inflammatory effects by suppressing NF-κB signaling more effectively (77, 78). Thus, tailoring exercise regimens to specific intensities and modalities may be critical for optimizing neuroprotective benefits and mitigating neuroinflammation in different contexts.
Further research is warranted to elucidate the precise molecular mechanisms underpinning these distinct responses and to develop personalized exercise prescriptions for individuals at risk for neurodegenerative disorders. In Table 1, we summarize the studies that have investigated the relationship between exercise and neurogenesis.
The Summary of the Study Related to the Physical Activity and Neurogenesis
Type of Study and Duration | Gender | Outcome | Ref. |
---|---|---|---|
Human | |||
Twenty-four weeks | F | Improve cognitive function and enhance the BDNF | (79) |
Late-life PE | F/M | Alternation of gene expression in the Hippocampal | (80) |
Three-times/week during 38 - 42 gestation’s weeks | F | Improve the cognition, learning, memory | (81) |
Physically activity before or during pregnancy | F | Improve the cognition, learning, memory | (82) |
Mouse | |||
30 min/day and 5 times/week, 4 weeks | m | Neurogenesis and memory | (83) |
Three months | m | Hippocampal neurogenesis | (84) |
Three months | m | Cognitive function and promotes microglial glucose metabolism and morphological plasticity | (85) |
Four weeks | m | Neurogenesis and memory and increased the hippocampal plasticity | (86) |
Six weeks, 40 min, 5 d a week | m | Ameliorate brain physiology and cognitive behavior. | (87) |
3.6. Endurance Training and Brain Plasticity
The influence of endurance exercise on brain plasticity is multifaceted, with cellular exercise promoting cell growth in the hippocampus, activating pathways that prevent cell death, and elevating the expression of BDNF (88). Brain-derived neurotrophic factor, a member of the neurotrophin family, plays a crucial role in neuronal restructuring, synaptic plasticity, and neurotransmitter release. Increased BDNF binds to its receptor, tyrosine-related kinase B (TrkB), activating the cyclic adenosine monophosphate (cAMP)-response element-binding protein (CREB). cyclic adenosine monophosphate-response element-binding protein activity enhances the expression of genes like c-fos and JunD, which support the growth of new neurons and the survival of existing neurons (89). Research on mice undergoing a 12-week endurance training regimen demonstrated increased neurogenesis and BDNF expression in the hippocampus. Additionally, aerobic exercise counteracted the negative impacts of obesity on memory and neurogenesis in mice fed a high-fat diet (90). Endurance exercise is also associated with increased growth of brain endothelial cells and angiogenesis, resulting in higher capillary density in the brain—a process uniquely linked to endurance exercise’s impact on brain function (91).
The protein pentraxin 3 (PTX3), a regulator of angiogenesis, has been implicated in promoting neurogenesis and cognitive recovery after an ischemic stroke. Pentraxin 3 -deficient animals exhibited more neuronal death and reduced vascular proliferation (92). Additionally, PTX3 expression in the brain increases after a stroke, aiding recovery by promoting the formation of new blood vessels (93). Recent data suggests a potential connection between the liver and the brain regarding the cognitive benefits of exercise (94). Elevated levels of glycosylphosphatidylinositol (GPI) phospholipase D1 (Gpld1), an enzyme in the liver, have been associated with enhanced cognitive performance and neurogenesis (95). Mice injected with plasma from exercising animals showed increased neuron quantity and improved learning and memory, attributed to elevated Gpld1 protein levels following exercise (95).
The hub genes related to neurogenesis as a result of long-term endurance exercise are illustrated in Figure 1A and B.
The confirmed positive correlation between neurogenesis and cognitive function in animal research aligns with the beneficial impacts of aerobic exercise on cognitive function observed in human studies (96). A meta-analysis of randomized controlled trials involving Alzheimer’s patients and individuals with MCI demonstrated significant improvements in cognitive performance following aerobic exercise interventions (97). Notably, moderate-intensity aerobic exercise had a more substantial impact on cognitive performance than low- and high-intensity exercise in individuals with MCI. These findings collectively emphasize the profound and diverse positive effects of endurance exercise on brain plasticity and cognitive function (98).
3.7. Brain-Derived Neurotrophic Factor Function and Exercise Training
Brain-derived neurotrophic factor is a protein from the neurotrophin family that is found in both the brain and peripheral organs, including skeletal muscle (99). Neurons in the central nervous system (CNS) are the primary producers of BDNF, and it plays a crucial role in memory and learning functions. Brain-derived neurotrophic factor binds to the tyrosine kinase b receptor (TrkB) to enhance processes like long-term potentiation, neurogenesis, axonal development, and synaptogenesis (100).
Scientific evidence suggests that the brain is a primary source of circulating BDNF at rest and during exercise, in addition to its local effects in the brain. Brain-derived neurotrophic factor also influences physiological processes like glucose metabolism and fat oxidation. Various factors can enhance the expression and functionality of BDNF, such as treatments like kainic acid, which elevates BDNF levels in the hippocampus by enhancing glutamatergic signaling (101).
Intermittent hypoxia has been shown to increase BDNF levels in neurons, particularly in the primary motor cortex (102). Additionally, PE, especially MICT, is recognized as an effective trigger for boosting BDNF production in both the brain and body. Several biochemical pathways have been proposed to explain how exercise increases BDNF production, including pathways involving intracellular calcium levels, reactive oxygen species (ROS), and systemic molecules like lactate (103).
One model suggests that exercise raises intracellular calcium levels, which stimulates CaMKII and enhances the MAP-K pathway, leading to the activation of CREB transcription and subsequent BDNF transcription (104). Another theory proposes that exercise increases BDNF production by enhancing the effectiveness of ROS. This model posits that exercise boosts mitochondrial activity, resulting in higher ROS production, which in turn activates cAMP-CREB and promotes BDNF transcription (105).
Lactate produced during PE, particularly during high-intensity exercise, may also stimulate the creation of BDNF (106). Lactate has been shown to increase NMDA receptor activation, elevate neuronal calcium levels, and enhance BDNF transcription. High-intensity interval training, characterized by short periods of intense activity with brief rest intervals, has been demonstrated to elevate brain BDNF production in healthy rats. Evidence suggests that HIIT increases hydrogen peroxide (H₂O₂) and TNF-α in the brain, potentially stimulating BDNF or CREB synthesis (107).
While recent studies have shown the beneficial impact of HIIT on BDNF levels, the specific anatomical areas responsive to increased neurotrophins after HIIT remain to be fully identified. Further research, including a more thorough assessment of the chemical mechanisms involved, is necessary to deepen our understanding of the intricate relationship between exercise, particularly HIIT, and BDNF production in the brain (107). Based on these studies, we can conclude that exercise training may improve neurogenesis, plasticity, and neurotrophin levels (107).
3.8. Practical Recommendations for Implementing Physical Activity Neuroinflammation and Neurodegenerative
Malashenko et al. have created a novel digital model that combines the Zettelkasten pedagogy with an interval repetition method to provide thorough and complete student instruction. The approach aims to optimize the learning experience and efficacy by enhancing student focus and long-term knowledge retention. This study employs a quantitative research approach, integrating experiments and surveys. The model's efficacy was evaluated using achievement tests and metacognitive awareness questionnaires. The concept was shown to be efficacious in augmenting the educational experience and enhancing students' performance. The Zettelkasten mechanism facilitates attention management, while the interval repetition technique enhances memory retention. The students who employed the model achieved superior scores and indicated greater comprehension and awareness of their learning. This work is the pioneering attempt to combine Zettelkasten and the interval repetition method into a unified classroom learning approach. The approach may be integrated into the educational methodology or offered as instructional modules. Nevertheless, the research contains inherent constraints that must be recognized (108).
The COVID-19 epidemic has resulted in the proliferation of online platforms for health colleges in Bali that are available at no cost. Nevertheless, selecting the optimal platform might be difficult because of the plethora of choices accessible on the internet. To tackle this problem, a novel method called the Weighted Product-based CIPP assessment model has been devised. The quality of the model is essential for achieving success in identifying the optimal online platform. The objective of Divayana et al.'s study was to evaluate the efficacy of integrating the Weighted Product method algorithm with the CIPP model in identifying the optimal platform for health colleges during the epidemic. A survey consisting of eight questions was used to evaluate the accuracy of the computation. The research included a panel of 20 specialists from various health institutions in Bali. The descriptive-quantitative analysis assessed the quality % of the computation simulation by comparing it with the quality standard using an eleven-point scale. The findings indicated a quality percentage of 87.250%, classifying it as excellent. This study has a substantial influence on the area of educational assessment by integrating the Weighted Product approach with the CIPP model. This integration simplifies the task of educational evaluators in identifying the optimal online platform for online learning both during and after the COVID-19 pandemic (109).
Hargitai and Bencsik examine the correlation between the culture of a learning company and key elements that impact digital transformation, such as leadership style, training, digital preparedness, and trust. This study used a grounded theory approach to investigate the influence of these elements on the operation of learning organizations. A total of 618 evaluable answers were obtained from the survey administered to managers of industrial enterprises in Eastern Europe. The precise linear structural model was analyzed using the PLS-SEM approach. The findings indicate that supportive leadership behavior and management style promote employee growth and training, enhancing their preparedness for digitalization and Industry 4.0 technologies. Training enhances proficiency in digitalization technologies, while leadership endorsement impacts digital trust and workforce adaptation to digital technology. This study expands upon prior research by explicitly examining the effects on learning organizations within the digitalization framework. This study determines that supportive leadership is essential in influencing the culture of learning business and facilitating digital transformation (110).
The findings underscore moderate physical activity's potent anti-inflammatory and antioxidant effects, highlighting its significance for neuroprotection and overall brain health (111). However, translating these findings into effective public health interventions requires practical recommendations that are accessible to all. Community-based programs promoting brisk walking, cycling, or swimming can be implemented, offering supervised sessions and educational resources on proper technique and individualized goal setting (112). One effective strategy is the implementation of community-based programs that promote accessible and enjoyable forms of moderate-intensity exercise, such as brisk walking groups, cycling clubs, or aquatic fitness classes (113). Effective translation of the neuroprotective advantages of PE into practical advice for those at risk of neurodegenerative illnesses is possible. These recommendations aim to integrate different forms of physical activity into a feasible and enduring lifestyle regimen that can optimize brain health, enhance cognitive abilities, and maybe postpone the development of neurodegenerative disorders such as AD, PD, and other types of dementia (114).
To practically implement these discoveries, it is advisable to include regular aerobic exercise, resistance training sessions, HIIT, mind-body exercises, flexibility and stretching routines, consistency and progression, social involvement and enjoyment, monitoring and adjustment, and seeking healthcare professionals for guidance. Regular aerobic activity, such as brisk walking, running, swimming, or cycling, is suggested to be engaged for a minimum of 150 minutes each week (115, 116). The efficacy of this particular kind of exercise has been shown to augment the volume of the hippocampus, enhance cerebral blood circulation, and elevate the levels of BDNF. These factors are crucial in promoting neurogenesis and synaptic plasticity, particularly with memory and learning processes. Resistance training interventions, including weightlifting, resistance bands, and body-weight exercises such as squats and push-ups, may enhance executive skills, memory, and general cognitive well-being (115).
The suggested frequency for HIIT is one to two times per week. This training involves brief bouts of high-intensity activity followed by periods of rest or low-intensity exercise. The efficacy of this training modality in rapidly increasing levels of BDNF, enhancing brain plasticity, and improving cognitive processes like memory and executive control has been shown. Engaging in mind-body exercises such as yoga, Tai Chi, or Pilates may alleviate stress and anxiety, enhance mental clarity, focus, and emotional control, and promote physical balance and flexibility. Performance of balance and coordination exercises activates brain areas associated with motor control and spatial awareness, promoting the preservation of cognitive skills associated with movement and balance. Regular performance of flexibility and stretching exercises is recommended, preferably daily or after other exercise sessions, for a minimum duration of 10 - 15 minutes (115, 117).
4. Future Research and Limitations
Further investigation is necessary to identify the specific anatomical regions that exhibit responses to elevated neurotrophin levels during HIIT. Addressing this significant limitation is essential for advancing future research. Additionally, subsequent studies should explore the potential consequences of overtraining, as well as the relative benefits and drawbacks of exercise, given its established associations with neuroprotection. Although the link between physical activity and neuroprotection is recognized, the biological mechanisms that underpin this relationship remain insufficiently understood, highlighting the need for further exploration of these pathways.
There is a pressing requirement for additional research examining the effects of various exercise modalities (e.g., resistance training versus aerobic exercise) on neuroinflammation and cognitive outcomes, to identify the most effective intervention strategies. One limitation of the current study is that the reviewed literature often employs heterogeneous methodologies, complicating direct comparisons of results. Variations in exercise types, intensities, durations, and participant demographics can contribute to inconsistent findings. Furthermore, factors such as diet, genetics, and overall lifestyle may significantly influence the neuroprotective effects of physical activity; however, these variables are not consistently controlled in existing studies.
While the advantages of moderate exercise are well-documented, the potential adverse effects of overtraining on cognitive function and neuroinflammation warrant further investigation, as current research may not comprehensively address this issue. Many studies focus on specific populations, such as older adults or individuals with MCI, which may limit the generalizability of the findings to broader demographic groups. Additionally, accurately measuring neuroinflammation and cognitive function presents challenges, and reliance on self-reported physical activity levels may introduce bias into the research outcomes.
Acknowledgements
References
-
1.
Majid A. Neuroprotection in stroke: past, present, and future. ISRN Neurol. 2014;2014:515716. [PubMed ID: 24579051]. [PubMed Central ID: PMC3918861]. https://doi.org/10.1155/2014/515716.
-
2.
Abedpoor N, Taghian F, Hajibabaie F. Physical activity ameliorates the function of organs via adipose tissue in metabolic diseases. Acta Histochem. 2022;124(2):151844. [PubMed ID: 35045377]. https://doi.org/10.1016/j.acthis.2022.151844.
-
3.
Hou Y, Dan X, Babbar M, Wei Y, Hasselbalch SG, Croteau DL, et al. Ageing as a risk factor for neurodegenerative disease. Nat Rev Neurol. 2019;15(10):565-81. [PubMed ID: 31501588]. https://doi.org/10.1038/s41582-019-0244-7.
-
4.
Poewe W, Seppi K, Tanner CM, Halliday GM, Brundin P, Volkmann J, et al. Parkinson disease. Nat Rev Dis Primers. 2017;3:17013. [PubMed ID: 28332488]. https://doi.org/10.1038/nrdp.2017.13.
-
5.
Hamer M, Chida Y. Physical activity and risk of neurodegenerative disease: a systematic review of prospective evidence. Psychol Med. 2009;39(1):3-11. [PubMed ID: 18570697]. https://doi.org/10.1017/S0033291708003681.
-
6.
Diniz BS, Mendes-Silva AP, Silva LB, Bertola L, Vieira MC, Ferreira JD, et al. Oxidative stress markers imbalance in late-life depression. J Psychiatr Res. 2018;102:29-33. [PubMed ID: 29574402]. https://doi.org/10.1016/j.jpsychires.2018.02.023.
-
7.
Singh A, Kukreti R, Saso L, Kukreti S. Oxidative Stress: A Key Modulator in Neurodegenerative Diseases. Molecules. 2019;24(8). [PubMed ID: 31013638]. [PubMed Central ID: PMC6514564]. https://doi.org/10.3390/molecules24081583.
-
8.
Wareham LK, Liddelow SA, Temple S, Benowitz LI, Di Polo A, Wellington C, et al. Solving neurodegeneration: common mechanisms and strategies for new treatments. Mol Neurodegener. 2022;17(1):23. [PubMed ID: 35313950]. [PubMed Central ID: PMC8935795]. https://doi.org/10.1186/s13024-022-00524-0.
-
9.
Nazam F, Shaikh S, Nazam N, Alshahrani AS, Hasan GM, Hassan MI. Mechanistic insights into the pathogenesis of neurodegenerative diseases: towards the development of effective therapy. Mol Cell Biochem. 2021;476(7):2739-52. [PubMed ID: 33687588]. https://doi.org/10.1007/s11010-021-04120-6.
-
10.
Kumar R, Aadil KR, Mondal K, Mishra YK, Oupicky D, Ramakrishna S, et al. Neurodegenerative disorders management: state-of-art and prospects of nano-biotechnology. Crit Rev Biotechnol. 2022;42(8):1180-212. [PubMed ID: 34823433]. https://doi.org/10.1080/07388551.2021.1993126.
-
11.
Grossman SP. A Textbook of Physiological Psychology. Hoboken, New Jersey: Wiley; 1967.
-
12.
Pascual-Leone A, Amedi A, Fregni F, Merabet LB. The plastic human brain cortex. Annu Rev Neurosci. 2005;28:377-401. [PubMed ID: 16022601]. https://doi.org/10.1146/annurev.neuro.27.070203.144216.
-
13.
Bavelier D, Neville HJ. Cross-modal plasticity: where and how? Nat Rev Neurosci. 2002;3(6):443-52. [PubMed ID: 12042879]. https://doi.org/10.1038/nrn848.
-
14.
Cohen EJ, Quarta E, Bravi R, Granato A, Minciacchi D. Neural plasticity and network remodeling: From concepts to pathology. Neuroscience. 2017;344:326-45. [PubMed ID: 28069532]. https://doi.org/10.1016/j.neuroscience.2016.12.048.
-
15.
Dzyubenko E, Hermann DM. Role of glia and extracellular matrix in controlling neuroplasticity in the central nervous system. Seminars Immunopathol. 2023;45(3):377-87. https://doi.org/10.1007/s00281-023-00989-1.
-
16.
Johnson BP, Cohen LG. Chapter 30 - Applied strategies of neuroplasticity. In: Younger DS, editor. Handbook of Clinical Neurology. 196. Amsterdam, Netherlands: Elsevier; 2023. p. 599-609.
-
17.
Phillips C. Lifestyle Modulators of Neuroplasticity: How Physical Activity, Mental Engagement, and Diet Promote Cognitive Health during Aging. Neural Plast. 2017;2017:3589271. [PubMed ID: 28695017]. [PubMed Central ID: PMC5485368]. https://doi.org/10.1155/2017/3589271.
-
18.
Kim DH, Kim JH, Park K. The impact of regular exercise, competition experience, and physical self-efficacy on psychological resilience. J Sport Psychol. 2023;32(3):1-19.
-
19.
Hajibabaie F, Abedpoor N, Taghian F, Safavi K. A Cocktail of Polyherbal Bioactive Compounds and Regular Mobility Training as Senolytic Approaches in Age-dependent Alzheimer's: the In Silico Analysis, Lifestyle Intervention in Old Age. J Mol Neurosci. 2023;73(2-3):171-84. [PubMed ID: 36631703]. https://doi.org/10.1007/s12031-022-02086-8.
-
20.
Huuha AM, Norevik CS, Moreira JBN, Kobro-Flatmoen A, Scrimgeour N, Kivipelto M, et al. Can exercise training teach us how to treat Alzheimer's disease? Ageing Res Rev. 2022;75:101559. [PubMed ID: 34999248]. https://doi.org/10.1016/j.arr.2022.101559.
-
21.
Erickson KI, Donofry SD, Sewell KR, Brown BM, Stillman CM. Cognitive Aging and the Promise of Physical Activity. Annu Rev Clin Psychol. 2022;18:417-42. [PubMed ID: 35044793]. https://doi.org/10.1146/annurev-clinpsy-072720-014213.
-
22.
Caspersen CJ, Powell KE, Christenson GM. Physical activity, exercise, and physical fitness: definitions and distinctions for health-related research. Public Health Rep. 1985;100(2):126-31. [PubMed ID: 3920711]. [PubMed Central ID: PMC1424733].
-
23.
Physical Activity Guidelines Advisory Committee. Physical activity guidelines advisory committee report, 2008. Washington, DC, US; 2008. NaN p.
-
24.
Kang J, Ratamess N. Which comes first? Resistance before aerobic exercise or vice versa? ACSM's Health Fitness J. 2014;18(1):9-14.
-
25.
Garber CE, Blissmer B, Deschenes MR, Franklin BA, Lamonte MJ, Lee I, et al. Quantity and quality of exercise for developing and maintaining cardiorespiratory, musculoskeletal, and neuromotor fitness in apparently healthy adults: guidance for prescribing exercise. Med Sci Sports Exercise. 2011;43(7).
-
26.
Weston KS, Wisloff U, Coombes JS. High-intensity interval training in patients with lifestyle-induced cardiometabolic disease: a systematic review and meta-analysis. Br J Sports Med. 2014;48(16):1227-34. [PubMed ID: 24144531]. https://doi.org/10.1136/bjsports-2013-092576.
-
27.
Fisher G, Brown AW, Bohan Brown MM, Alcorn A, Noles C, Winwood L, et al. High intensity interval-vs moderate intensity-training for improving cardiometabolic health in overweight or obese males: a randomized controlled trial. PloS one. 2015;10(10). e0138853.
-
28.
Gibala MJ, Jones AM. Physiological and performance adaptations to high-intensity interval training. Nestle Nutr Inst Workshop Ser. 2013;76:51-60. [PubMed ID: 23899754]. https://doi.org/10.1159/000350256.
-
29.
Gillen JB, Gibala MJ. Is high-intensity interval training a time-efficient exercise strategy to improve health and fitness? Appl Physiol Nutr Metab. 2014;39(3):409-12. [PubMed ID: 24552392]. https://doi.org/10.1139/apnm-2013-0187.
-
30.
Saanijoki T, Nummenmaa L, Eskelinen JJ, Savolainen AM, Vahlberg T, Kalliokoski KK, et al. Affective Responses to Repeated Sessions of High-Intensity Interval Training. Med Sci Sports Exerc. 2015;47(12):2604-11. [PubMed ID: 26110694]. https://doi.org/10.1249/MSS.0000000000000721.
-
31.
Saucedo Marquez CM, Vanaudenaerde B, Troosters T, Wenderoth N. High-intensity interval training evokes larger serum BDNF levels compared with intense continuous exercise. J Appl Physiol (1985). 2015;119(12):1363-73. [PubMed ID: 26472862]. https://doi.org/10.1152/japplphysiol.00126.2015.
-
32.
Astorino TA, Thum JS. Interval training elicits higher enjoyment versus moderate exercise in persons with spinal cord injury. J Spinal Cord Med. 2018;41(1):77-84. [PubMed ID: 27808004]. [PubMed Central ID: PMC5810810]. https://doi.org/10.1080/10790268.2016.1235754.
-
33.
Coetsee C, Terblanche E. Cerebral oxygenation during cortical activation: the differential influence of three exercise training modalities. A randomized controlled trial. Eur J Appl Physiol. 2017;117(8):1617-27. [PubMed ID: 28567669]. https://doi.org/10.1007/s00421-017-3651-8.
-
34.
Santos-Concejero J, Billaut F, Grobler L, Olivan J, Noakes TD, Tucker R. Brain oxygenation declines in elite Kenyan runners during a maximal interval training session. Eur J Appl Physiol. 2017;117(5):1017-24. [PubMed ID: 28321639]. https://doi.org/10.1007/s00421-017-3590-4.
-
35.
Robinson MM, Lowe VJ, Nair KS. Increased Brain Glucose Uptake After 12 Weeks of Aerobic High-Intensity Interval Training in Young and Older Adults. J Clin Endocrinol Metab. 2018;103(1):221-7. [PubMed ID: 29077855]. [PubMed Central ID: PMC5761491]. https://doi.org/10.1210/jc.2017-01571.
-
36.
Cefis M, Chaney R, Wirtz J, Meloux A, Quirie A, Leger C, et al. Molecular mechanisms underlying physical exercise-induced brain BDNF overproduction. Front Mol Neurosci. 2023;16:1275924. [PubMed ID: 37868812]. [PubMed Central ID: PMC10585026]. https://doi.org/10.3389/fnmol.2023.1275924.
-
37.
Livia Livinț P, Diana C, Aida A, Stefan S, Dafin M. The Modulation of Hippocampus Plasticity. In: Douglas D, editor. Hippocampus. Rijeka: IntechOpen; 2023. Ch. 6 p. https://doi.org/10.5772/intechopen.110386.
-
38.
Zalouli V, Rajavand H, Bayat M, Khaleghnia J, Sharifianjazi F, Jafarinazhad F, et al. Adult hippocampal neurogenesis (AHN) controls central nervous system and promotes peripheral nervous system regeneration via physical exercise. Biomed Pharmacother. 2023;165:115078. [PubMed ID: 37390707]. https://doi.org/10.1016/j.biopha.2023.115078.
-
39.
Groot C, Hooghiemstra AM, Raijmakers PG, van Berckel BN, Scheltens P, Scherder EJ, et al. The effect of physical activity on cognitive function in patients with dementia: A meta-analysis of randomized control trials. Ageing Res Rev. 2016;25:13-23. [PubMed ID: 26607411]. https://doi.org/10.1016/j.arr.2015.11.005.
-
40.
Frederiksen KS, Gjerum L, Waldemar G, Hasselbalch SG. Effects of Physical Exercise on Alzheimer's Disease Biomarkers: A Systematic Review of Intervention Studies. J Alzheimers Dis. 2018;61(1):359-72. [PubMed ID: 29154278]. https://doi.org/10.3233/JAD-170567.
-
41.
Sujkowski A, Hong L, Wessells RJ, Todi SV. The protective role of exercise against age-related neurodegeneration. Ageing Res Rev. 2022;74:101543. [PubMed ID: 34923167]. [PubMed Central ID: PMC8761166]. https://doi.org/10.1016/j.arr.2021.101543.
-
42.
Furrer R, Hawley JA, Handschin C. The molecular athlete: exercise physiology from mechanisms to medals. Physiol Rev. 2023;103(3):1693-787. [PubMed ID: 36603158]. [PubMed Central ID: PMC10110736]. https://doi.org/10.1152/physrev.00017.2022.
-
43.
Chung Y, Hsiao YT, Huang WC. Physiological and Psychological Effects of Treadmill Overtraining Implementation. Biol (Basel). 2021;10(6). [PubMed ID: 34200732]. [PubMed Central ID: PMC8230380]. https://doi.org/10.3390/biology10060515.
-
44.
Qiu Y, Fernandez-Garcia B, Lehmann HI, Li G, Kroemer G, Lopez-Otin C, et al. Exercise sustains the hallmarks of health. J Sport Health Sci. 2023;12(1):8-35. [PubMed ID: 36374766]. [PubMed Central ID: PMC9923435]. https://doi.org/10.1016/j.jshs.2022.10.003.
-
45.
Abdelhamid RF, Nagano S. Crosstalk between Oxidative Stress and Aging in Neurodegeneration Disorders. Cells. 2023;12(5). [PubMed ID: 36899889]. [PubMed Central ID: PMC10001353]. https://doi.org/10.3390/cells12050753.
-
46.
Scheffer DDL, Latini A. Exercise-induced immune system response: Anti-inflammatory status on peripheral and central organs. Biochimica Biophysica Acta (BBA)-Mol Basis Dis. 2020;1866(10):165823. https://doi.org/10.1016/j.bbadis.2020.165823.
-
47.
Cordeiro LMS, Rabelo PCR, Moraes MM, Teixeira-Coelho F, Coimbra CC, Wanner SP, et al. Physical exercise-induced fatigue: the role of serotonergic and dopaminergic systems. Braz J Med Biol Res. 2017;50(12). e6432. [PubMed ID: 29069229]. [PubMed Central ID: PMC5649871]. https://doi.org/10.1590/1414-431X20176432.
-
48.
Ando S, Fujimoto T, Sudo M, Watanuki S, Hiraoka K, Takeda K, et al. The neuromodulatory role of dopamine in improved reaction time by acute cardiovascular exercise. J Physiol. 2024;602(3):461-84. [PubMed ID: 38165254]. https://doi.org/10.1113/JP285173.
-
49.
Perez EC, Gehm KH, Lobo VG, Olvera M, Leasure JL. Adulthood effects of developmental exercise in rats. Dev Psychobiol. 2024;66(1). e22444. [PubMed ID: 38131238]. https://doi.org/10.1002/dev.22444.
-
50.
Burgos-Jara C, Cerda-Kohler H, Aedo-Muñoz E, Miarka B. Eccentric Resistance Training: A Methodological Proposal of Eccentric Muscle Exercise Classification Based on Exercise Complexity, Training Objectives, Methods, and Intensity. Appl Sci. 2023;13(13):7969. [PubMed ID: doi:10.3390/app13137969].
-
51.
Najm A, Niculescu AG, Grumezescu AM, Beuran M. Emerging Therapeutic Strategies in Sarcopenia: An Updated Review on Pathogenesis and Treatment Advances. Int J Mol Sci. 2024;25(8). [PubMed ID: 38673885]. [PubMed Central ID: PMC11050002]. https://doi.org/10.3390/ijms25084300.
-
52.
Azevedo CV, Hashiguchi D, Campos HC, Figueiredo EV, Otaviano S, Penitente AR, et al. The effects of resistance exercise on cognitive function, amyloidogenesis, and neuroinflammation in Alzheimer's disease. Front Neurosci. 2023;17:1131214. [PubMed ID: 36937673]. [PubMed Central ID: PMC10017453]. https://doi.org/10.3389/fnins.2023.1131214.
-
53.
Liu Y, Chu JMT, Yan T, Zhang Y, Chen Y, Chang RCC, et al. Short-term resistance exercise inhibits neuroinflammation and attenuates neuropathological changes in 3xTg Alzheimer's disease mice. J Neuroinflammation. 2020;17(1):4. [PubMed ID: 31900170]. [PubMed Central ID: PMC6942350]. https://doi.org/10.1186/s12974-019-1653-7.
-
54.
Pena GS, Paez HG, Johnson TK, Halle JL, Carzoli JP, Visavadiya NP, et al. Hippocampal Growth Factor and Myokine Cathepsin B Expression following Aerobic and Resistance Training in 3xTg-AD Mice. Int J Chronic Dis. 2020;2020:5919501. [PubMed ID: 32090058]. [PubMed Central ID: PMC7011393]. https://doi.org/10.1155/2020/5919501.
-
55.
Ozbeyli D, Sari G, Ozkan N, Karademir B, Yuksel M, Cilingir Kaya OT, et al. Protective effects of different exercise modalities in an Alzheimer's disease-like model. Behav Brain Res. 2017;328:159-77. [PubMed ID: 28390878]. https://doi.org/10.1016/j.bbr.2017.03.044.
-
56.
Jafarzadeh G, Shakerian S, Farbood Y, Ghanbarzadeh M. Effects of Eight Weeks of Resistance Exercises on Neurotrophins and Trk Receptors in Alzheimer Model Male Wistar Rats. Basic Clin Neurosci. 2021;12(3):349-59. [PubMed ID: 34917294]. [PubMed Central ID: PMC8666928]. https://doi.org/10.32598/bcn.2021.2067.1.
-
57.
Garcia-Garcia I, Fernandez-Andujar M, Narvaez M, Garcia-Casares N. Assessing adipokines as potential biomarkers of dementia, Alzheimer's disease, and mild cognitive impairment: A systematic review and meta-analysis. Obes Rev. 2023;24(8). e13573. [PubMed ID: 37165483]. https://doi.org/10.1111/obr.13573.
-
58.
Gao L, Zhang Y, Sterling K, Song W. Brain-derived neurotrophic factor in Alzheimer’s disease and its pharmaceutical potential. Translational Neurodegeneration. 2022;11(1):4. https://doi.org/10.1186/s40035-022-00279-0.
-
59.
Caffino L, Mottarlini F, Fumagalli F. Born to Protect: Leveraging BDNF Against Cognitive Deficit in Alzheimer's Disease. CNS Drugs. 2020;34(3):281-97. [PubMed ID: 32052374]. https://doi.org/10.1007/s40263-020-00705-9.
-
60.
Usmani MT, Krattli RP, El-Khatib SM, Le ACD, Smith SM, Baulch JE, et al. BDNF Augmentation Using Riluzole Reverses Doxorubicin-Induced Decline in Cognitive Function and Neurogenesis. Neurotherapeutics. 2023;20(3):838-52. [PubMed ID: 36720792]. [PubMed Central ID: PMC10275819]. https://doi.org/10.1007/s13311-022-01339-z.
-
61.
Banerjee M, Shenoy RR. Emphasizing roles of BDNF promoters and inducers in Alzheimer's disease for improving impaired cognition and memory. J Basic Clin Physiol Pharmacol. 2023;34(2):125-36. [PubMed ID: 34751526]. https://doi.org/10.1515/jbcpp-2021-0182.
-
62.
Gholami A. Alzheimer's disease: The role of proteins in formation, mechanisms, and new therapeutic approaches. Neurosci Lett. 2023;817:137532. [PubMed ID: 37866702]. https://doi.org/10.1016/j.neulet.2023.137532.
-
63.
Fonseca-Gomes J, Costa-Coelho T, Ferreira-Manso M, Inteiro-Oliveira S, Vaz SH, Alemãn-Serrano N, et al. A small TAT-TrkB peptide prevents BDNF receptor cleavage and restores synaptic physiology in Alzheimer's disease. Mol Ther. 2024;32(10):3372-401. https://doi.org/10.1016/j.ymthe.2024.08.022.
-
64.
Huang B, Chen K, Li Y. Aerobic exercise, an effective prevention and treatment for mild cognitive impairment. Front Aging Neurosci. 2023;15:1194559. [PubMed ID: 37614470]. [PubMed Central ID: PMC10442561]. https://doi.org/10.3389/fnagi.2023.1194559.
-
65.
Girottto I. On the neuroprotective role of myokines induced by resistance training in adults with Mild Cognitive Impairment. Padova, Italy: University of Padia; 2022.
-
66.
He Y, Wang Q, Wu H, Dong Y, Peng Z, Guo X, et al. The role of IGF-1 in exercise to improve obesity-related cognitive dysfunction. Front Neurosci. 2023;17:1229165. [PubMed ID: 37638322]. [PubMed Central ID: PMC10447980]. https://doi.org/10.3389/fnins.2023.1229165.
-
67.
Hall L. Which type of exercise, Cardiovascular exercise or Resistance exercise is more effective for the prevention of development of Alzheimer's disease?. Groningen, Netherlands: University of Groningen; 2024.
-
68.
Marrano N, Biondi G, Borrelli A, Rella M, Zambetta T, Di Gioia L, et al. Type 2 Diabetes and Alzheimer's Disease: The Emerging Role of Cellular Lipotoxicity. Biomol. 2023;13(1). [PubMed ID: 36671568]. [PubMed Central ID: PMC9855893]. https://doi.org/10.3390/biom13010183.
-
69.
Kim YK, Jo D, Arjunan A, Ryu Y, Lim YH, Choi SY, et al. Identification of IGF-1 Effects on White Adipose Tissue and Hippocampus in Alzheimer's Disease Mice via Transcriptomic and Cellular Analysis. Int J Mol Sci. 2024;25(5). [PubMed ID: 38473814]. [PubMed Central ID: PMC10931577]. https://doi.org/10.3390/ijms25052567.
-
70.
Tan L, Zhang H, Li H, Sun S, Lyu Q, Jiang Y. Blueberry extracts antagonize Abeta(25-35) neurotoxicity and exert a neuroprotective effect through MEK-ERK-BDNF/UCH-L1 signaling pathway in rat and mouse hippocampus. Nutr Neurosci. 2024;27(7):745-60. [PubMed ID: 37647279]. https://doi.org/10.1080/1028415X.2023.2252640.
-
71.
Pahlavani HA. Exercise therapy to prevent and treat Alzheimer's disease. Front Aging Neurosci. 2023;15:1243869. [PubMed ID: 37600508]. [PubMed Central ID: PMC10436316]. https://doi.org/10.3389/fnagi.2023.1243869.
-
72.
Augusto-Oliveira M, Arrifano GP, Leal-Nazare CG, Santos-Sacramento L, Lopes-Araujo A, Royes LFF, et al. Exercise Reshapes the Brain: Molecular, Cellular, and Structural Changes Associated with Cognitive Improvements. Mol Neurobiol. 2023;60(12):6950-74. [PubMed ID: 37518829]. https://doi.org/10.1007/s12035-023-03492-8.
-
73.
Reddy I, Yadav Y, Dey CS. Cellular and Molecular Regulation of Exercise-A Neuronal Perspective. Cell Mol Neurobiol. 2023;43(4):1551-71. [PubMed ID: 35986789]. https://doi.org/10.1007/s10571-022-01272-x.
-
74.
Matsumori A. Nuclear Factor-kappaB is a Prime Candidate for the Diagnosis and Control of Inflammatory Cardiovascular Disease. Eur Cardiol. 2023;18. e40. [PubMed ID: 37456770]. [PubMed Central ID: PMC10345985]. https://doi.org/10.15420/ecr.2023.10.
-
75.
Chen J, Zhu T, Yu D, Yan B, Zhang Y, Jin J, et al. Moderate Intensity of Treadmill Exercise Rescues TBI-Induced Ferroptosis, Neurodegeneration, and Cognitive Impairments via Suppressing STING Pathway. Mol Neurobiol. 2023;60(9):4872-96. [PubMed ID: 37193866]. [PubMed Central ID: PMC10415513]. https://doi.org/10.1007/s12035-023-03379-8.
-
76.
Thakur S, Dhapola R, Sarma P, Medhi B, Reddy DH. Neuroinflammation in Alzheimer's Disease: Current Progress in Molecular Signaling and Therapeutics. Inflammation. 2023;46(1):1-17. [PubMed ID: 35986874]. https://doi.org/10.1007/s10753-022-01721-1.
-
77.
Sabita R, Siagian M, Ibrahim N. High-Intensity Interval Training’s Effect on Cognitive Functions. Indonesian J Multidisciplinary Sci. 2023;2(9):3106-14.
-
78.
Zhu X, Chen W, Thirupathi A. Sprint Interval Training Improves Brain-Derived Neurotropic Factor-Induced Benefits in Brain Health-A Possible Molecular Signaling Intervention. Biol (Basel). 2024;13(8). [PubMed ID: 39194500]. [PubMed Central ID: PMC11351939]. https://doi.org/10.3390/biology13080562.
-
79.
Rondao CAM, Mota MP, Oliveira MM, Peixoto F, Esteves D. Multicomponent exercise program effects on fitness and cognitive function of elderlies with mild cognitive impairment: Involvement of oxidative stress and BDNF. Front Aging Neurosci. 2022;14:950937. [PubMed ID: 36092805]. [PubMed Central ID: PMC9453672]. https://doi.org/10.3389/fnagi.2022.950937.
-
80.
Berchtold NC, Prieto GA, Phelan M, Gillen DL, Baldi P, Bennett DA, et al. Hippocampal gene expression patterns linked to late-life physical activity oppose age and AD-related transcriptional decline. Neurobiol Aging. 2019;78:142-54. [PubMed ID: 30927700]. [PubMed Central ID: PMC6901108]. https://doi.org/10.1016/j.neurobiolaging.2019.02.012.
-
81.
Clapp JF. Morphometric and neurodevelopmental outcome at age five years of the offspring of women who continued to exercise regularly throughout pregnancy. J Pediatr. 1996;129(6):856-63. [PubMed ID: 8969727]. https://doi.org/10.1016/s0022-3476(96)70029-x.
-
82.
Esteban-Cornejo I, Martinez-Gomez D, Tejero-Gonzalez CM, Izquierdo-Gomez R, Carbonell-Baeza A, Castro-Pinero J, et al. Maternal physical activity before and during the prenatal period and the offspring's academic performance in youth. The UP&DOWN study. J Matern Fetal Neonatal Med. 2016;29(9):1414-20. [PubMed ID: 26135457]. https://doi.org/10.3109/14767058.2015.1049525.
-
83.
Yook JS, Rakwal R, Shibato J, Takahashi K, Koizumi H, Shima T, et al. Leptin in hippocampus mediates benefits of mild exercise by an antioxidant on neurogenesis and memory. Proc Natl Acad Sci USA. 2019;116(22):10988-93. [PubMed ID: 31085646]. [PubMed Central ID: PMC6561194]. https://doi.org/10.1073/pnas.1815197116.
-
84.
Zhang TY, Keown CL, Wen X, Li J, Vousden DA, Anacker C, et al. Environmental enrichment increases transcriptional and epigenetic differentiation between mouse dorsal and ventral dentate gyrus. Nat Commun. 2018;9(1):298. [PubMed ID: 29352183]. [PubMed Central ID: PMC5775256]. https://doi.org/10.1038/s41467-017-02748-x.
-
85.
Zhang SS, Zhu L, Peng Y, Zhang L, Chao FL, Jiang L, et al. Long-term running exercise improves cognitive function and promotes microglial glucose metabolism and morphological plasticity in the hippocampus of APP/PS1 mice. J Neuroinflammation. 2022;19(1):34. [PubMed ID: 35123512]. [PubMed Central ID: PMC8817568]. https://doi.org/10.1186/s12974-022-02401-5.
-
86.
Lee MC, Rakwal R, Shibato J, Inoue K, Chang H, Soya H. DNA microarray-based analysis of voluntary resistance wheel running reveals novel transcriptome leading robust hippocampal plasticity. Physiol Rep. 2014;2(11). [PubMed ID: 25413326]. [PubMed Central ID: PMC4255813]. https://doi.org/10.14814/phy2.12206.
-
87.
McGreevy KR, Tezanos P, Ferreiro-Villar I, Palle A, Moreno-Serrano M, Esteve-Codina A, et al. Intergenerational transmission of the positive effects of physical exercise on brain and cognition. Proc Natl Acad Sci U S A. 2019;116(20):10103-12. [PubMed ID: 31010925]. [PubMed Central ID: PMC6525532]. https://doi.org/10.1073/pnas.1816781116.
-
88.
Jaberi S, Fahnestock M. Mechanisms of the Beneficial Effects of Exercise on Brain-Derived Neurotrophic Factor Expression in Alzheimer's Disease. Biomolecules. 2023;13(11). [PubMed ID: 38002258]. [PubMed Central ID: PMC10669442]. https://doi.org/10.3390/biom13111577.
-
89.
Thompson C. The role of the BDNF Val66Met polymorphism in synaptic plasticity and Recognition memory. Auckland, North Island: University of Auckland; 2014.
-
90.
Lee TH, Yau SY. From Obesity to Hippocampal Neurodegeneration: Pathogenesis and Non-Pharmacological Interventions. Int J Mol Sci. 2020;22(1). [PubMed ID: 33379163]. [PubMed Central ID: PMC7796248]. https://doi.org/10.3390/ijms22010201.
-
91.
Bonanni R, Cariati I, Tarantino U, D'Arcangelo G, Tancredi V. Physical Exercise and Health: A Focus on Its Protective Role in Neurodegenerative Diseases. J Funct Morphol Kinesiol. 2022;7(2). [PubMed ID: 35645300]. [PubMed Central ID: PMC9149968]. https://doi.org/10.3390/jfmk7020038.
-
92.
Rodriguez-Grande B, Swana M, Nguyen L, Englezou P, Maysami S, Allan SM, et al. The acute-phase protein PTX3 is an essential mediator of glial scar formation and resolution of brain edema after ischemic injury. J Cereb Blood Flow Metab. 2014;34(3):480-8. [PubMed ID: 24346689]. [PubMed Central ID: PMC3948128]. https://doi.org/10.1038/jcbfm.2013.224.
-
93.
Rajkovic I, Wong R, Lemarchand E, Rivers-Auty J, Rajkovic O, Garlanda C, et al. Pentraxin 3 promotes long-term cerebral blood flow recovery, angiogenesis, and neuronal survival after stroke. J Mol Med (Berl). 2018;96(12):1319-32. [PubMed ID: 30315331]. [PubMed Central ID: PMC6245246]. https://doi.org/10.1007/s00109-018-1698-6.
-
94.
Pedersen BK. Physical activity and muscle-brain crosstalk. Nat Rev Endocrinol. 2019;15(7):383-92. [PubMed ID: 30837717]. https://doi.org/10.1038/s41574-019-0174-x.
-
95.
Horowitz AM, Fan X, Bieri G, Smith LK, Sanchez-Diaz CI, Schroer AB, et al. Blood factors transfer beneficial effects of exercise on neurogenesis and cognition to the aged brain. Sci. 2020;369(6500):167-73. [PubMed ID: 32646997]. [PubMed Central ID: PMC7879650]. https://doi.org/10.1126/science.aaw2622.
-
96.
Islam MR, Valaris S, Young MF, Haley EB, Luo R, Bond SF, et al. Exercise hormone irisin is a critical regulator of cognitive function. Nat Metab. 2021;3(8):1058-70. [PubMed ID: 34417591]. [PubMed Central ID: PMC10317538]. https://doi.org/10.1038/s42255-021-00438-z.
-
97.
Meng Q, Yin H, Wang S, Shang B, Meng X, Yan M, et al. The effect of combined cognitive intervention and physical exercise on cognitive function in older adults with mild cognitive impairment: a meta-analysis of randomized controlled trials. Aging Clin Exp Res. 2022;34(2):261-76. [PubMed ID: 34383248]. https://doi.org/10.1007/s40520-021-01877-0.
-
98.
Ayari S, Abellard A, Carayol M, Guedj E, Gavarry O. A systematic review of exercise modalities that reduce pro-inflammatory cytokines in humans and animals' models with mild cognitive impairment or dementia. Exp Gerontol. 2023;175:112141. [PubMed ID: 36898593]. https://doi.org/10.1016/j.exger.2023.112141.
-
99.
Fernandes J, Arida RM, Gomez-Pinilla F. Physical exercise as an epigenetic modulator of brain plasticity and cognition. Neurosci Biobehav Rev. 2017;80:443-56. [PubMed ID: 28666827]. [PubMed Central ID: PMC5705447]. https://doi.org/10.1016/j.neubiorev.2017.06.012.
-
100.
Numakawa T, Odaka H. Roles of Trk receptors, tyrosine kinase receptors for neurotrophins, in the developing CNS. In: Numakawa T, Odaka H, editors. Receptor Tyrosine Kinases in Neurodegenerative and Psychiatric Disorders. Amsterdam, Netherlands: Elsevier; 2023. p. 79-115.
-
101.
Murawska-Cialowicz E, de Assis GG, Clemente FM, Feito Y, Stastny P, Zuwala-Jagiello J, et al. Effect of four different forms of high intensity training on BDNF response to Wingate and Graded Exercise Test. Sci Rep. 2021;11(1):8599. [PubMed ID: 33883635]. [PubMed Central ID: PMC8060323]. https://doi.org/10.1038/s41598-021-88069-y.
-
102.
Welch JF, Sutor TW, Vose AK, Perim RR, Fox EJ, Mitchell GS. Synergy between Acute Intermittent Hypoxia and Task-Specific Training. Exerc Sport Sci Rev. 2020;48(3):125-32. [PubMed ID: 32412926]. [PubMed Central ID: PMC7416561]. https://doi.org/10.1249/JES.0000000000000222.
-
103.
Buzdagli Y, Ozan M, Baygutalp N, Oget F, Karayigit R, Yuce N, et al. The effect of high-intensity intermittent and moderate-intensity continuous exercises on neurobiological markers and cognitive performance. BMC Sports Sci Med Rehabil. 2024;16(1):39. [PubMed ID: 38326816]. [PubMed Central ID: PMC10848527]. https://doi.org/10.1186/s13102-024-00831-7.
-
104.
Raefsky SM, Mattson MP. Adaptive responses of neuronal mitochondria to bioenergetic challenges: Roles in neuroplasticity and disease resistance. Free Radic Biol Med. 2017;102:203-16. [PubMed ID: 27908782]. [PubMed Central ID: PMC5209274]. https://doi.org/10.1016/j.freeradbiomed.2016.11.045.
-
105.
Jodeiri Farshbaf M, Ghaedi K, Megraw TL, Curtiss J, Shirani Faradonbeh M, Vaziri P, et al. Does PGC1alpha/FNDC5/BDNF Elicit the Beneficial Effects of Exercise on Neurodegenerative Disorders? Neuromolecular Med. 2016;18(1):1-15. [PubMed ID: 26611102]. https://doi.org/10.1007/s12017-015-8370-x.
-
106.
Muller P, Duderstadt Y, Lessmann V, Muller NG. Lactate and BDNF: Key Mediators of Exercise Induced Neuroplasticity? J Clin Med. 2020;9(4). [PubMed ID: 32326586]. [PubMed Central ID: PMC7230639]. https://doi.org/10.3390/jcm9041136.
-
107.
Mahalakshmi B, Maurya N, Lee SD, Bharath Kumar V. Possible Neuroprotective Mechanisms of Physical Exercise in Neurodegeneration. Int J Mol Sci. 2020;21(16). [PubMed ID: 32824367]. [PubMed Central ID: PMC7460620]. https://doi.org/10.3390/ijms21165895.
-
108.
Malashenko GT, Kosov ME, Frumina SV, Grishina OA, Alandarov RA, Ponkratov VV, et al. A Digital Model of Full-Cycle Training Based on the Zettelkasten and Interval Repetition System. Emerg Sci J. 2023;7:1-15.
-
109.
Divayana DGH, Suyasa PWA, Santiyadnya N, Andayani MSL, Sundayana IM, Astawa IND, et al. Utilization of the Weighted Product-Based CIPP Evaluation Model in Determining the Best Online Platform. HighTech Innovation J. 2023;4(1):233-48.
-
110.
Hargitai DM, Bencsik A. The role of leadership in digital learning organizations. Emerging Sci J. 2023;7:111-24.
-
111.
Merecz-Sadowska A, Sitarek P, Zajdel K, Sztandera W, Zajdel R. Genus Sambucus: Exploring Its Potential as a Functional Food Ingredient with Neuroprotective Properties Mediated by Antioxidant and Anti-Inflammatory Mechanisms. Int J Mol Sci. 2024;25(14). [PubMed ID: 39063085]. [PubMed Central ID: PMC11277136]. https://doi.org/10.3390/ijms25147843.
-
112.
Quilico E, Sweet S, Duncan L, Wilkinson S, Bonnell K, Alarie C, et al. Exploring a peer-based physical activity program in the community for adults with moderate-to-severe traumatic brain injury. Brain Injury. 2023;37(8):728-36. https://doi.org/10.1080/02699052.2023.2208375.
-
113.
Prieto LA. Planning and Evaluation of Community-based Physical Activity Programs: Families of Autistic Children & Latino Older Adults. Madison, United States: University of Wisconsin-Madison; 2023.
-
114.
Tsukamoto H, Suga T, Takenaka S, Tanaka D, Takeuchi T, Hamaoka T, et al. Greater impact of acute high-intensity interval exercise on post-exercise executive function compared to moderate-intensity continuous exercise. Physiol Behav. 2016;155:224-30. [PubMed ID: 26723268]. https://doi.org/10.1016/j.physbeh.2015.12.021.
-
115.
Nascimento CM, Pereira JR, de Andrade LP, Garuffi M, Talib LL, Forlenza OV, et al. Physical exercise in MCI elderly promotes reduction of pro-inflammatory cytokines and improvements on cognition and BDNF peripheral levels. Curr Alzheimer Res. 2014;11(8):799-805. [PubMed ID: 25212919]. https://doi.org/10.2174/156720501108140910122849.
-
116.
Bull FC, Al-Ansari SS, Biddle S, Borodulin K, Buman MP, Cardon G, et al. World Health Organization 2020 guidelines on physical activity and sedentary behaviour. Br J Sports Med. 2020;54(24):1451-62. [PubMed ID: 33239350]. [PubMed Central ID: PMC7719906]. https://doi.org/10.1136/bjsports-2020-102955.
-
117.
Wayne PM, Walsh JN, Taylor-Piliae RE, Wells RE, Papp KV, Donovan NJ, et al. Effect of tai chi on cognitive performance in older adults: systematic review and meta-analysis. J Am Geriatr Soc. 2014;62(1):25-39. [PubMed ID: 24383523]. [PubMed Central ID: PMC4055508]. https://doi.org/10.1111/jgs.12611.