Abstract
Background:
It is well accepted that skeletal muscle conforms to exercise stimulus by increasing capillary density and angiogenesis, but there is less evidence regarding the effect of resistance training on capillary density in flexor hallucis longus (FHL) and soleus muscle.Objectives:
In this study, we evaluated the effect of resistance training on capillary density around soleus and FHL muscles in type 1 diabetic rats.Materials and Methods:
Thirty-six male rats were divided into four groups: (1) control; (2) diabetic; (3) diabetic trained and (4) control trained (n = 9 each). A Single intraperitoneal injection of Streptozotocin at a dose of 55 mg/kg was used for induction of diabetes. The rats in the trained group undertook one training session per day for 3 days/week. Training was done with the use of a 1 meter high ladder inclined at 80°. After 4 weeks, the plasma nitrite concentrations were measured. Capillary/fiber ratio was determined around soleus and FHL muscles by immunohistochemistry.Results:
Plasma Nitric Oxide (NO) concentration was increased after resistance training in diabetic animals (P < 0.05). Capillary/fiber ratio around the soleus muscle of diabetic group was more than control rats. Resistance training did not alter capillary/fiber ratio in diabetic animals (1.00 ± 0.6 vs. 1.07 ± 0.07, respectively). Capillary/fiber ratio around FHL muscle was significantly different between diabetic and control and did not alter after exercise (diabetes: 1.1702 ± 0.09; diabetic trained: 1.1714 ± 0.08; control: 0.79 ± 0.08; control trained: 0.73 ± 0.03). There was a positive correlation between plasma NO concentration and capillary density in the soleus muscle (R2 = 0.65).Conclusions:
Resistance training could not improve capillary/fiber ratio in soleus and FHL muscle of diabetic animals in spite of increase in some angiogenic factors including NO.Keywords
1. Background
Type II diabetes is a consequential cause of untimely mortality and morbidity related to cardiovascular disease (CVD), blindness, kidney and nerve disease, and amputation. People with Type 2 diabetes reveal insulin resistance in skeletal muscle (1). Also, hypertension, coronary artery disease, cerebrovascular attacks and effects on micro- and macro circulation in patients with diabetes are more common (2, 3). While diabetes management has largely focused on control of hyperglycemia, the presence of the abnormal feature of angiogenesis could cause or contribute to many of the clinical manifestations of diabetes (4).
The sprouting of new vessels from pre-existing vessels in response to angiogenic molecules and hypoxia is called angiogenesis (5). In recent times, both type 1 and type II diabetes have been shown to influence angiogenic growth factors and inhibitors in skeletal muscle (6). Decreased angiogenesis is thought to affect damaged tissue repair in diabetic patients (7).
Regular exercise has been known to have great benefits, involving increased performance and healthy longevity (8). More recently, exercise has been shown to exert significant positive impacts on an increasing number of diseases in humans, including diabetes, obesity and cardiovascular disease (9). Exercise training makes better cardiovascular function and evaluates vascular transport capacity of skeletal muscle (10). In the past decade, a number of clinical studies have obviously been described in patients with T2DM, resistance training decreases the percentage of glycosylated hemoglobin, increases glucose disposal, and even improves the lipid and cardiovascular disease risk profile (11). Also, in a normal subject, resistance exercise elevated skeletal muscle Vascular Endithelial Growth Factor (VEGF), VEGF receptor. The increases in muscle angiogenic growth factor expression in response to resistance exercises are the same in timing and magnitude as responses to acute aerobic exercise and are consistent with resistance exercise improving muscle angiogenesis (12). Also, this kind of training increases circulating endothelial progenitor cell (EPC) counts and decreases asymmetric dimethylarginine (ADMA) levels reflecting elevated angiogenesis and promoted endothelial function, which might contribute to cardiovascular risk reduction (13).
Skeletal muscle is a compound tissue composed of connective tissue, nerves and muscle fibers. Type I slow-twitch, oxidative fibers are slow in force generation (14) and have an oxidative profile (15, 16). Type IIa fast-twitch, oxidative fibers are fast in force generation but have similar oxidative profiles to the type I fibers (17). Changes among muscle fiber type, oxidative capacity, vascularization, and capillary exchange capacity during exercise, are well recognized (18).
It is well accepted that skeletal muscle conforms to exercise stimulus by increasing capillary density and/or capillary/fiber ratio, but there is less evidence, for the relationship between capillary ratioin Flexor Hallucis Longus (FHL) and soleus muscles and resistance training. Because angiogenesis, by increasing capillary exchange area, contributes to increased blood flow and oxygen uptake, thus representing an essential adaptive reaction of skeletal muscle to exercise for improvement of physical performance, aerobic capacity, facilitating oxygen transport, conductance and muscle extraction. Also, angiogenesis factors such as nitric oxide (NO) in skeletal muscle have a main role in this process and the change of skeletal muscle adaptation in diabetes (19). Therefore, in this study, we evaluated the effect of resistance training on capillary density around soleus and FHL muscles in type 1 diabetic rats.
2. Objectives
In this study, we evaluated the effect of resistance training on capillary density around soleus and FHL muscles in type 1 diabetic rats.
3. Materials and Methods
3.1. Animals and Experimental Groups
In accordance with previous studies, Thirty-six male wistar rats, (288 ± 22 gr) were used in this experiment (20). The rats were purchased from Pasteur’s institute (Tehran, Iran) and kept in the central animal house of the university. The animals were housed three per cage in an air-conditioned animal room with 12 h light/dark cycle, at a room temperature between 22 ± 2°C, and food and water were given ad libitum. They were divided into four groups: (1) control; (2) diabetic; (3) diabetic trained and (4) control trained (n = 9 each). The ethical committee of the Tarbiat Modarres University approved all protocols and procedures.
3.2. Induction of Diabetes
A single intraperitoneal injection of Streptozotocin (STZ) at a dose of 55 mg/kg (Sigma-Aldrich, St. Louis, MO) was used for induction of diabetes. STZ was dissolved (20 mg/mL) in a cold 0.1 M citrate buffer (pH 4.5). Non-diabetic rats received a similar volume of citrate buffer only. Blood glucose concentrations were measured using tail vein following overnight fasting, five days after the STZ injection. Blood glucose level higher than 16 mmol/L was considered as indicative of diabetes (21).
3.3. Resistance Training Protocol
For 4 weeks, the rats in the trained group undertook one training session per day, 3 days/week, plus an initial familiarization session in total as previously described (22). Seven days after injection of Streptozotocin (STZ), the rats started the training protocol, using the climbing ladder and weights which were attached to the base of the tail with adhesive tape and a clip. Training was done with the use of a 1 meter high ladder inclined at 80°. There were 26 rungs on the ladder, evenly spaced. Experimental rats were familiarized with the exercise by practicing climbing from the ladder, before inducing diabetes, in the trained group. The rats were placed at the bottom of the climbing apparatus and motivated to climb the ladder by touching and grooming their tail. For encouraging the rats to execute the exercise training and in order to reduce the stress, we used electrical stimulation, forced air, food restriction/reward, and cold water. The rats rested when they reached the top of the ladder. All animals were weighed once every 4 days to monitor weight gains and, for the resistance trained animals, to estimate the amount of weight to append to their tails for the remainder of the week. In the preliminary phase, the rats were adjusted to climbing the ladder with progressive loading on each consecutive training day. The training group of rats undertook 6 repetitions ascending the ladder interspersed with 1minute rest intervals. After 3 minutes rest, a second set of 6 repetitions was performed with 1min rest intervals. On the first day, the rats trained with the equivalent of 30% body mass (BM) as load appended to their tail (6 repetitions/2 sets). On the second day, the training load was elevated to 50% of BM (6 reps/2 sets), and on the third day, an additional set of repetitions was performed with 50% BM (6 reps/3 sets). The training load was progressively increased until the seventh day, when the training load reached to 100% BM (acquaintance day and six progressive resistance-training days). In the flat load resistance-training phase, the rats continued to train with 100% BM, 6 repetitions per set, 3 sets per day, and 3 days per week until the end of 4th week. Warming-up and cooling down consisted of 2 repetitions climbing the ladder without weights appended to the tail, immediately pre and post each training session. Non- trained (sedentary) rats were controlled on the same days and times as the trained groups in order to minimize any stress imputable to handling. Training protocol is present in Tables 1 and 2 (8, 23).
Training in Rats: Increasing the Load Period (From the Beginning to the End of the Second Week Protocol) a,b,c
Sessions | Set 1 | Set 2 | Set3 |
---|---|---|---|
1 | (30) | (30) | 0 |
2 | (50) | (50) | 0 |
3 | (50) | (50) | (50) |
4 | (75) | (75) | (75) |
5 | (75) | (75) | (75) |
6 | (75) | (75) | (75) |
Training Rats in a Fixed Load Period (From the Beginning to the End of the Third Week)
Warm-Up | SET 1 | SET 2 | SET3 | COOL DOWN |
---|---|---|---|---|
2 × without weights | 6 × 100%body weight | 6 × 100% body weight | 6 × 100% body weight | 2 × without weights |
3.4. Sacrificing and Sampling
After 4 weeks, the rats were anaesthetized using an intraperitoneal injection of a mixture of Ketamine (50 mg/kg) and Xylazine (5 mg/kg). The animals were sacrificed between 9.00 and 12.00 a.m. Approximately 2 mL of blood was obtained from the abdominal vena cava and centrifuged (3000 rpm; 15 minutes).
3.5. Plasma NO Measurement
The plasma NO concentrations were measured by estimation of its stable oxidation product (nitrite) using the Griess reaction method (Promega Corp., Madison, USA, Cat#G2930) as previously described (23). Briefly, the samples were added to a 96-well enzymatic assay plate. Then, sulfanilamide and N-1-naphtylethylenediamine dihydrochloride solutions were added to the samples, respectively. A micro plate reader at the wavelength of 520 nm was used for measured absorbance. The plasma NO concentrations were determined in comparison to nitrite standard reference curve. 2.5 μMnitrite was the limit detection.
Capillary density was expressed as capillaries/fiber ratio. Capillaries/fiber ratio was determined separately for type I and type II fibers by counting the number of capillaries around each individual fiber and then computing the mean. Sequential estimation analyses were also carried out to determine the number of measurements required to be representative of an individual’s number of capillaries per type I and II fibers. Capillaries were described by staining sections for alkaline phosphates as previously described. The capillarization was analyzed using the method of capillary domains. This method does provide the overall indexes of capillary density and capillary/fiber ratio for soleus and FHL (24).
3.6. Statistical Analysis
All data are presented as means± SEM by using SPSS statistical analysis software version 16. Analysis of variance (ANOVA) was used to analyze the differences between groups using Tukey’s posthoc test. Pearson’s coefficient was used for correlation analysis. P less than 0.05 was considered statistically significant.
4. Results
Table 3 shows the descriptive characteristics of the animals. Five days after injection of STZ, the weights were measured. At the end of training program the weight increased in the control and control training groups (25% and 17/87%, respectively), while diabetic and diabetic training lost weight (-12% and -7/75%, respectively).
Descriptive Characteristics of the Animals
Measurement Time | Weight of Rats Before Induction of Diabetes | Weight Rats, 5 Days After Injection STZ | Weight Rats After 4 Weeks of Resistance Training | ||||
---|---|---|---|---|---|---|---|
Groups | number r | Mean. gr | Standard deviation | Mean. gr | Standard deviation | Mean. gr | Standard deviation |
Control | 8 | 291/9 | 18.1 | 302.0 | 21.6 | 364.6 | 30.3 |
Control training | 8 | 291.0 | 36.1 | 303.4 | 40.4 | 343.6 | 49.7 |
Diabetic | 10 | 289.2 | 16.6 | 265.6 | 15.0 | 252.0 | 18.7 |
Diabetic training | 10 | 283.8 | 17.5 | 269.0 | 15.5 | 261.6 | 23.7 |
4.1. Plasma NO Concentration
Plasma NO concentration in diabetic animals was significantly higher than control (P < 0.05). 4 weeks resistance training in diabetic animals led to significant differences (diabetic: 7.2 ± 0.03, diabetic trained: 10.34 ± 2.1 µmol/L), While in control animals, resistance exercise didn’t have significant effect (control trained: 6.45 ± 0.35, control: 6.27 ± 0.03 µmol/L).
4.2. Angiogenesis in Soleus Muscle
Capillary density in soleus muscle of control and diabetic group were 17.45 ± 0.92 and 26.15 ± 1.37/mm2, respectively (P < 0.05). There was no significant effect of resistance training on capillary density of type 1 muscle (soleus muscle) in diabetic rats (26.15 ± 1.37 vs. 25.08 ± 1.37/mm2). A significant effect of resistance training on capillary density was observed between control and control trained group (17.45 ± 0.92 vs. 22.22 ± 1.24/mm2, respectively (P < 0.05) (Figure 1 a). Capillary/fiber ratio in the soleus muscle of diabetic group was more than control rats. Resistance training did not alter capillary/fiber ratio in diabetic animals (1.00 ± 0.6 vs. 1.07 ± 0.07, respectively), while it significantly improved capillary/fiber ratio in control rats (0.56 ± 0.03 vs. 0.69 ± 0.04, respectively; P < 0.05) (Figure 1 b). Samples of immunohistochemical staining of the soleus muscles are presented in Figure 2.
Capillary Density (A) and Capillary/Fiber Ratio (B) in the Soleus Muscle
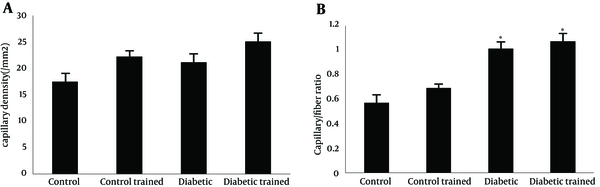
Immunohistochemical Staining Of the Soleus Muscle in Experimental Groups
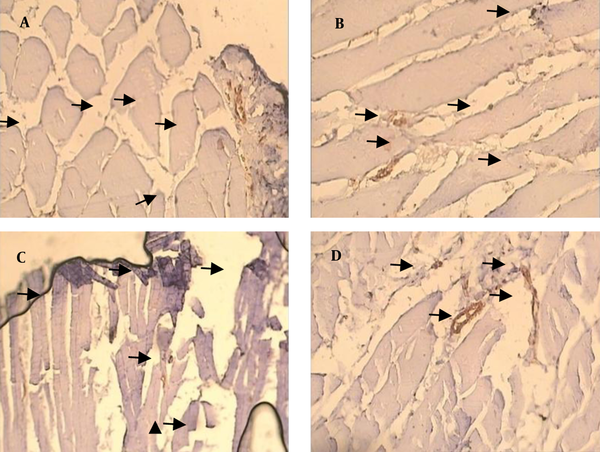
4.3. Angiogenesis in FHL Muscle
Capillary density in FHL muscle of diabetic animals was higher than control (43.62 ± 3.62 and 26.26 ± 2.74 mm2, respectively; P < 0.05). Resistance training reduced capillary density in diabetic rats (26.76 ± 2.25 and 43.62 ± 3.62 mm2, respectively; P < 0.05), while it did not have effect in control group (control: 26.26 ± 2.74; control training: 23.00 ± 1.79 mm2) (P < 0.05) (Figure 3 a).
Capillary/fiber ratio in FHL muscle was significant different between diabetic and control (1.17 ± 0.09 vs. 0.79 ± 0.08). This ratio did not alter in diabetic and control animals after exercise (diabetes: 1.1702 ± 0.09; diabetic trained: 1.1714 ± 0.08; control: 0.79 ± 0.08; control trained 0.73 ± 0.03) (Figure 3 b). Samples of immunohistochemical staining of FHL muscles are presented in Figure 4.
Capillary Density (A) and Capillary/Fiber Ratio (B) in the FHL Muscle
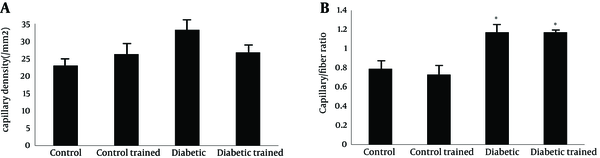
Immunohistochemical Staining of the FHL Muscle in Experimental Groups
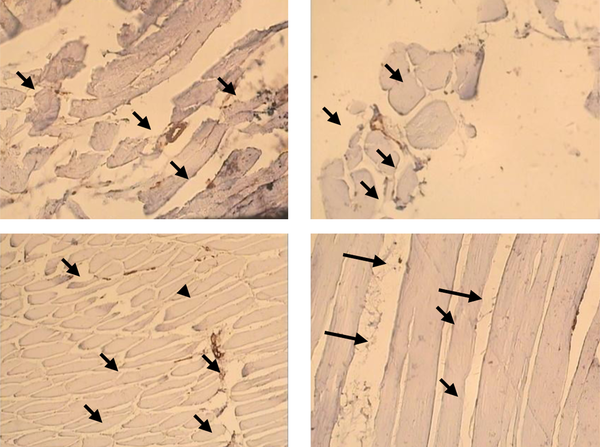
4.4. Correlation Between Plasma Nitrite Level and Capillary Density
We investigated the correlation between plasma nitrite concentration and capillary density in two types of muscle. Results showed that there was a positive correlation between plasma nitrite and capillary density around in soleus muscle (R2 = 0.65), while, there was a weak positive correlation between plasma nitrite and capillary density in FHL muscle (R2 = 0.0039) (Figure 5).
Correlation Between Plasma NO Concentration and Capillary Density in the Soleus (A) and FHL (B) Muscles
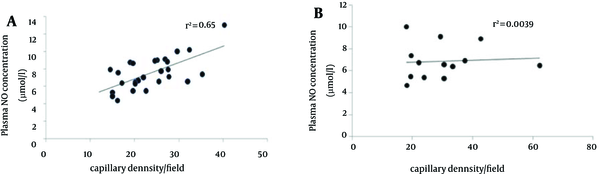
5. Discussion
The aim of the present study was to compare the effect of resistance training on angiogenesis in fast and slow muscles and its correlation with plasma nitrite concentration in type I diabetic rats. Previous studies showed that NO is an important factor for regulation and improving the angiogenesis process (25). In this study, we found reduced plasma NO concentration in diabetic animals compared to control group which support the results of previous studies (26-28). Also, resistance training increased plasma NO concentration in diabetic animals which led to significant differences between diabetic and diabetic trained group. In agreement with our results, previous studies demonstrated that different types of exercise are able to improve plasma NO levels even in diabetic subjects (29). Chronic exercise increases NO production as early as one week after the start of training (30).
To preserve the functional balance between metabolic demand and oxygen delivery, the skeletal and cardiac muscles increase capillary density or capillary-to-fiber ratio. The increased capillary network plays an important role in improving aerobic capacity, facilitating oxygen transport, conductance, and muscle extraction; therefore contributing to increased maximal oxygen uptake and physical performance (19). Exercise-induced angiogenesis is a well-described response observed in several exercised muscles such as tibialis anterior, soleus, gastrocnemius, and vastus lateralis in different subjects (31, 32).
In this study, we found that although resistance training changed capillary density, however, capillary density/fiber ratio did not alter in type I diabetic rats in both soleus and FHL muscles. Previous studies have reported that exercise-induced increase in skeletal muscle capillarization is accompanied with positive effects on the basal levels of angiogenic growth factors in diabetic skeletal muscle (30). In this study, we found that although plasma nitrite level increased after training, however, capillary/fiber ratio did not change.
Previous studies indicated that exercise training enhanced the number of capillaries per square millimeter of muscle in humans and these increases are greatest in slow twitch (ST) (Type I) and fast oxidative-glycolytic (FOG) skeletal muscle (9). Exercise training increases the number of capillaries per square millimeter of muscle and these increases are greatest around SO (Type I) and FOG (Type IIA) skeletal muscle. Results from exercise trained rats demonstrated that exercise training-induced angiogenesis of capillaries within and among skeletal muscles is preeminent in the muscle tissue with the greatest relative evaluation in fiber activity during training bouts (33, 34).
It is indicated that the training program resulted in a considerable proliferation of the capillary network and it is noticeable that the enhanced capillarization was not restricted to a specific fiber-type, since capillary/fiber-type area increased equally for types I, IIA and IIB fibers (35). In agreement with the present study, in the trained rat soleus (84% slow oxidative) in which the area of subsarcolemal mitochondria is increased, there is hardly any change in capillary/fiber ratio and this suggests that the amount of activity superimposed on normally very active soleus is not great enough to produce an increase in capillary density, although it is sufficient to produce an increase in aerobic capacity (36). Also, this kind of training increases circulating EPC counts and decreases ADMA levels, reflecting elevated angiogenesis and promoted endothelial function, which might contribute to cardiovascular risk reduction (13). Also, non vascular effects of exercise are oxidative phosphorylation, muscle hypertrophy, oxygen transport capacity etc. In addition, endothelial vasodilation, nitric oxide prodaction, oxidative stress vasculogenesis by EPCs, capillary vessel formation are other effects of exercise in vascular (37).
The study by Matsunaga et al. provides more evidence that NO plays a critical role in angiogenesis (38). In the present study, there was a strongly positive correlation between plasma NO level and capillary density in the soleus muscle, while, there was a weak positive correlation between plasma no and capillary density in FHL muscle that is in agreement with previous studies with different types of exercise or subject (32).
In conclusion, although resistance training increased plasma NO levels and other important factor that can improve angiogenesis, however, it seems that resistance training could not improve capillary/fiber ratio in both soleus and FHL muscle of diabetic animals.
References
-
1.
DeFronzo RA, Tripathy D. Skeletal muscle insulin resistance is the primary defect in type 2 diabetes. Diabetes Care. 2009;32 Suppl 2:S157-63. [PubMed ID: 19875544]. https://doi.org/10.2337/dc09-S302.
-
2.
Hornick T, Aron DC. Preventing and managing diabetic complications in elderly patients. Cleve Clin J Med. 2008;75(2):153-8. [PubMed ID: 18290359].
-
3.
Aiello LP, Wong JS. Role of vascular endothelial growth factor in diabetic vascular complications. Kidney Int Suppl. 2000;77:S113-9. [PubMed ID: 10997700].
-
4.
Martin A, Komada MR, Sane DC. Abnormal angiogenesis in diabetes mellitus. Med Res Rev. 2003;23(2):117-45. [PubMed ID: 12500286]. https://doi.org/10.1002/med.10024.
-
5.
Yla-Herttuala S, Rissanen TT, Vajanto I, Hartikainen J. Vascular endothelial growth factors: biology and current status of clinical applications in cardiovascular medicine. J Am Coll Cardiol. 2007;49(10):1015-26. [PubMed ID: 17349880]. https://doi.org/10.1016/j.jacc.2006.09.053.
-
6.
Kivela R, Silvennoinen M, Touvra AM, Lehti TM, Kainulainen H, Vihko V. Effects of experimental type 1 diabetes and exercise training on angiogenic gene expression and capillarization in skeletal muscle. FASEB J. 2006;20(9):1570-2. [PubMed ID: 16816123]. https://doi.org/10.1096/fj.05-4780fje.
-
7.
Galiano RD, Tepper OM, Pelo CR, Bhatt KA, Callaghan M, Bastidas N, et al. Topical vascular endothelial growth factor accelerates diabetic wound healing through increased angiogenesis and by mobilizing and recruiting bone marrow-derived cells. Am J Pathol. 2004;164(6):1935-47. [PubMed ID: 15161630]. https://doi.org/10.1016/S0002-9440(10)63754-6.
-
8.
Yan Z, Okutsu M, Akhtar YN, Lira VA. Regulation of exercise-induced fiber type transformation, mitochondrial biogenesis, and angiogenesis in skeletal muscle. J Appl Physiol (1985). 2011;110(1):264-74. [PubMed ID: 21030673]. https://doi.org/10.1152/japplphysiol.00993.2010.
-
9.
Perseghin G, Price TB, Petersen KF, Roden M, Cline GW, Gerow K, et al. Increased glucose transport-phosphorylation and muscle glycogen synthesis after exercise training in insulin-resistant subjects. N Engl J Med. 1996;335(18):1357-62. [PubMed ID: 8857019]. https://doi.org/10.1056/NEJM199610313351804.
-
10.
Adhihetty PJ, Uguccioni G, Leick L, Hidalgo J, Pilegaard H, Hood DA. The role of PGC-1alpha on mitochondrial function and apoptotic susceptibility in muscle. Am J Physiol Cell Physiol. 2009;297(1):C217-25. [PubMed ID: 19439529]. https://doi.org/10.1152/ajpcell.00070.2009.
-
11.
Zanuso S, Jimenez A, Pugliese G, Corigliano G, Balducci S. Exercise for the management of type 2 diabetes: a review of the evidence. Acta Diabetol. 2010;47(1):15-22. [PubMed ID: 19495557]. https://doi.org/10.1007/s00592-009-0126-3.
-
12.
Gavin TP, Drew JL, Kubik CJ, Pofahl WE, Hickner RC. Acute resistance exercise increases skeletal muscle angiogenic growth factor expression. Acta Physiol (Oxf). 2007;191(2):139-46. [PubMed ID: 17565567]. https://doi.org/10.1111/j.1748-1716.2007.01723.x.
-
13.
Schlager O, Giurgea A, Schuhfried O, Seidinger D, Hammer A, Groger M, et al. Exercise training increases endothelial progenitor cells and decreases asymmetric dimethylarginine in peripheral arterial disease: a randomized controlled trial. Atherosclerosis. 2011;217(1):240-8. [PubMed ID: 21481871]. https://doi.org/10.1016/j.atherosclerosis.2011.03.018.
-
14.
Witzmann FA, Kim DH, Fitts RH. Hindlimb immobilization: length-tension and contractile properties of skeletal muscle. J Appl Physiol Respir Environ Exerc Physiol. 1982;53(2):335-45. [PubMed ID: 7118655].
-
15.
Prince FP, Hikida RS, Hagerman FC, Staron RS, Allen WH. A morphometric analysis of human muscle fibers with relation to fiber types and adaptations to exercise. J Neurol Sci. 1981;49(2):165-79. [PubMed ID: 7217980].
-
16.
Sjogaard G, Saltin B. Extra- and intracellular water spaces in muscles of man at rest and with dynamic exercise. Am J Physiol. 1982;243(3):R271-80. [PubMed ID: 7114288].
-
17.
Eddinger TJ, Moss RL. Mechanical properties of skinned single fibers of identified types from rat diaphragm. Am J Physiol. 1987;253(2 Pt 1):C210-8. [PubMed ID: 3303962].
-
18.
Yang HT, Ren J, Laughlin MH, Terjung RL. Prior exercise training produces NO-dependent increases in collateral blood flow after acute arterial occlusion. Am J Physiol Heart Circ Physiol. 2002;282(1):H301-10. [PubMed ID: 11748075]. https://doi.org/10.1152/ajpheart.00160.2001.
-
19.
Richardson RS, Wagner H, Mudaliar SR, Saucedo E, Henry R, Wagner PD. Exercise adaptation attenuates VEGF gene expression in human skeletal muscle. Am J Physiol Heart Circ Physiol. 2000;279(2):H772-8. [PubMed ID: 10924077].
-
20.
Barauna VG, Magalhaes FC, Krieger JE, Oliveira EM. AT1 receptor participates in the cardiac hypertrophy induced by resistance training in rats. Am J Physiol Regul Integr Comp Physiol. 2008;295(2):R381-7. [PubMed ID: 18495827]. https://doi.org/10.1152/ajpregu.00933.2007.
-
21.
Taniyama Y, Morishita R, Hiraoka K, Aoki M, Nakagami H, Yamasaki K, et al. Therapeutic angiogenesis induced by human hepatocyte growth factor gene in rat diabetic hind limb ischemia model: molecular mechanisms of delayed angiogenesis in diabetes. Circulation. 2001;104(19):2344-50. [PubMed ID: 11696476].
-
22.
Lee S, Farrar RP. Resistance training induces muscle-specific changes in muscle mass and function in rat. J Exers Physiol Online. 2003;6(2):80-7.
-
23.
Nematollahi S, Nematbakhsh M, Haghjooyjavanmard S, Khazaei M, Salehi M. Inducible nitric oxide synthase modulates angiogenesis in ischemic hindlimb of rat. Biomed Pap Med Fac Univ Palacky Olomouc Czech Repub. 2009;153(2):125-9. [PubMed ID: 19771137].
-
24.
McCall GE, Byrnes WC, Dickinson A, Pattany PM, Fleck SJ. Muscle fiber hypertrophy, hyperplasia, and capillary density in college men after resistance training. J Appl Physiol (1985). 1996;81(5):2004-12. [PubMed ID: 8941522].
-
25.
Esfahani SP, Gharakhanlou R. Changes of plasma angiogenic factors during chronic resistance exercise in type 1 diabetic rats. Pakistan J Med Sci. 2012;28(2):328-32.
-
26.
Lash JM, Nase GP, Bohlen HG. Acute hyperglycemia depresses arteriolar NO formation in skeletal muscle. Am J Physiol. 1999;277(4 Pt 2):H1513-20. [PubMed ID: 10516190].
-
27.
Brodsky SV, Morrishow AM, Dharia N, Gross SS, Goligorsky MS. Glucose scavenging of nitric oxide. Am J Physiol Renal Physiol. 2001;280(3):F480-6. [PubMed ID: 11181410].
-
28.
Bohlen HG, Nase GP. Arteriolar nitric oxide concentration is decreased during hyperglycemia-induced betaII PKC activation. Am J Physiol Heart Circ Physiol. 2001;280(2):H621-7. [PubMed ID: 11158959].
-
29.
Egginton S. Invited review: activity-induced angiogenesis. Pflugers Arch. 2009;457(5):963-77. [PubMed ID: 18704490]. https://doi.org/10.1007/s00424-008-0563-9.
-
30.
Delp MD. Effects of exercise training on endothelium-dependent peripheral vascular responsiveness. Med Sci Sports Exerc. 1995;27(8):1152-7. [PubMed ID: 7476059].
-
31.
Amaral SL, Papanek PE, Greene AS. Angiotensin II and VEGF are involved in angiogenesis induced by short-term exercise training. Am J Physiol Heart Circ Physiol. 2001;281(3):H1163-9. [PubMed ID: 11514283].
-
32.
Lloyd PG, Prior BM, Yang HT, Terjung RL. Angiogenic growth factor expression in rat skeletal muscle in response to exercise training. Am J Physiol Heart Circ Physiol. 2003;284(5):H1668-78. [PubMed ID: 12543634]. https://doi.org/10.1152/ajpheart.00743.2002.
-
33.
Coyle EF. Improved muscular efficiency displayed as Tour de France champion matures. J Appl Physiol (1985). 2005;98(6):2191-6. [PubMed ID: 15774697]. https://doi.org/10.1152/japplphysiol.00216.2005.
-
34.
Fulco M, Cen Y, Zhao P, Hoffman EP, McBurney MW, Sauve AA, et al. Glucose restriction inhibits skeletal myoblast differentiation by activating SIRT1 through AMPK-mediated regulation of Nampt. Dev Cell. 2008;14(5):661-73. [PubMed ID: 18477450]. https://doi.org/10.1016/j.devcel.2008.02.004.
-
35.
Andersen P, Henriksson J. Capillary supply of the quadriceps femoris muscle of man: adaptive response to exercise. J Physiol. 1977;270(3):677-90. [PubMed ID: 198532].
-
36.
Hudlicka O. Development and adaptability of microvasculature in skeletal muscle. J Exp Biol. 1985;115:215-28. [PubMed ID: 3897444].
-
37.
Marini M, Veicsteinas A. The exercised skeletal muscle: a review. Eur J Trans Myol. 2010;20(3):105-20. https://doi.org/10.4081/bam.2010.3.105.
-
38.
Matsunaga T, Weihrauch DW, Moniz MC, Tessmer J, Warltier DC, Chilian WM. Angiostatin inhibits coronary angiogenesis during impaired production of nitric oxide. Circulation. 2002;105(18):2185-91. [PubMed ID: 11994253].