Abstract
Keywords
Countermovement Jump Handgrip Strength Heart Rate match analysis Rate of Perceived Exertion
1. Background
Boxing is a popular martial combat sport of intermittent nature relying on both aerobic and anaerobic energy transfer systems. The anthropometric and physiological characteristics of boxers have been well studied (1). For instance, it has been shown that boxing competition ranking was correlated with anaerobic threshold and handgrip muscle strength (HGS) (2) and that shoulder strength was correlated with number of wins (3). Also, Hanon et al. have highlighted the importance of a well-developed anaerobic and buffering capacity (4). The abovementioned studies improved our understanding of the physiological profile of boxers. On the other side, it would be very useful to know the variation of measures of anaerobic power (e.g. HGS and countermovement jump, CMJ), because muscle strength and power of upper and lower limbs have been shown to be key to success (1-3). Nevertheless, a lack of data on acute physiological responses to boxing has been observed.
Research in other combat sports, such as taekwondo (5) and kickboxing (6), examined the responses of HGS, CMJ and heart rate (HR) to official or simulated combats. In kickboxing, HGS decreased after an official combat and the magnitude of decrease was larger in winners than in losers (6). In taekwondo, CMJ increased and HGS likely decreased after a simulated combat (5). In addition to the acute physiological responses to boxing, a lack of information has also been shown with regards to the impact of different recovery strategies during breaks on these responses. During breaks, boxers might assume either sitting or standing body position; however, the effect of the body position on physiological aspects, such as CMJ, HGS, HR and rate of perceived exertion (RPE) has not been studied.
Although there were many studies in the literature on profiling physiological characteristics of boxers (3, 7), there was a lack of research regarding the acute physiological responses to boxing and the effect of body position during breaks on physiological parameters, such as CMJ, HGS, HR and RPE. Such knowledge would be of great practical value for coaches and fitness trainers working with boxers, because it would help optimize training procedures. Therefore, the aim of the present study was to examine the effect of two conditions (sitting versus standing) during breaks on HR responses, RPE and neuromuscular fatigue in a simulated boxing combat with three 3-min rounds and 1-min breaks.
2. Methods
A repeated-measures study design was used to examine the effect of body position on physiological aspects in boxers. Amateur male boxers (n = 15) (Table 1), members of an amateur boxing club in Athens (Greece), volunteered to participate in this study. Boxers were excluded in any of the following cases: a) having chronic disease, b) taking medications, or c) having an orthopedic condition that would limit their ability to perform tests. All boxers participated in at least three training sessions weekly. The local Institutional Review Board approved the study protocol which was in accordance with the ethical standards from the Declaration of Helsinki in 2008. Informed written consent from participants was obtained.
Basic Demographic Data of Participants (n = 15)
Parameter | Age, y | Body Mass, kg | Body Height, cm | BMI, kg.m-2 | Training Experience, y |
---|---|---|---|---|---|
Mean (SD) | 21.4 (6.3) | 67.7 (10.0) | 172 (6) | 22.9 (4.2) | 2.9 (1.9) |
Testing procedures were performed in the competitive period of season 2015 - 2016. The participants knew the testing procedures well, because the employed tests had been routinely administered to the members of this boxing club in the past. Mean HR was calculated for each round and break (Figure 1). RPE (6 - 20 Borg scale) was recorded immediately after each round. CMJ and HGS were measured before (two trials) and after combat (one trial). The simulated combat consisted of three 3-min rounds with 1-min breaks. Participants were divided into two groups: those who were sitting (n = 8) and those were standing (n = 7).
Height and body mass were measured with participants being barefoot and wearing minimal clothing. An electronic weight scale (HD-351 Tanita, Illinois, USA) measured body mass (in the nearest 0.1 kg) and a portable stadiometer (SECA, Leicester, UK) was used to evaluate height in the Frankfurt plane (0.001 m). Body mass index (BMI) was calculated as the quotient of body mass to height squared ((kg/m2). The isometric strength testing included two measures: right and left handgrip muscle strength. In the HGS, after having fitted the dynamometer to their hand so that the bar was rested on the phalanx of the index and ring finger, the participants were instructed to squeeze the handle of a handgrip dynamometer (Takei, Tokyo, Japan) as hard as possible standing, while having their elbow flexed at approximately 90°. The Takei handgrip dynamometer showing a systematic bias of 0.02 kg has been found to be more reliable than other dynamometers (8). The participants performed the CMJ test, where they began in an upright position with both feet together and were instructed to jump as high as possible using a fast countermovement. The depth of the countermovement was self-selected and participants were asked to land close to their point of take-off. Height of jump was estimated using the 3-cm version of Opto-jump (Microgate Engineering, Bolzano, Italy). CMJ has been shown to be reliable (Cronbach’s alpha = 0.98) (9). HR was recorded continuously during the simulated combat, including the rounds and the breaks, by Team2 Pro (Polar Electro Oy, Kempele, Finland). The 6-20 Borg scale was used an index of RPE immediately after each round.
Statistical analyses were performed using IBM SPSS v.20.0 (SPSS, Chicago, USA) and GraphPad Prism v. 7.0 (GraphPad Software, San Diego, USA). Data were expressed as mean and standard deviations of the mean (SD). Only the best score of pre-combat CMJ and HGS tests was included in the data analysis and the parametric analysis techniques were used. Two-way analysis of variance (ANOVA) examined the main effects of combat and body position in break and their interaction on physiological parameters. To interpret effect sizes (ES) for statistical differences in the ANOVA we used eta square classified as small (0.01 < η2 ≤ 0.06), medium (0.06 < η2 ≤ 0.14) and large (η2 > 0.14) (10). The level of significance was set at α = 0.05.
Testing Protocol
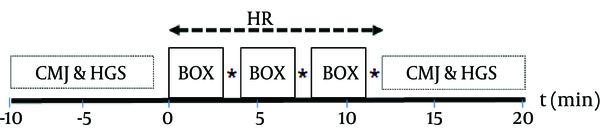
3. Results
A mixed analysis of variance found no time × body position interaction on all variables (P > 0.05). Nevertheless, in the case of RPE the p value almost reached statistical significance (P = 0.062, η2 = 0.426), where, compared to the sitting position, a trend of increased perception of effort for the standing position across time was highlighted (Figure 2). A main effect of time on HR (Figure 3), CMJ (Figure 4) and RPE was observed (P < 0.05), in which (a) CMJ after combat was higher than before (34.2 ± 4.6 vs. 32.2 ± 4.6 cm, respectively, P = 0.007, η2 = 0.443), (b) HR was higher in the third round than the second and the first one (187 ± 7, 184 ± 7 and 177 ± 12 bpm, respectively, P < 0.001, η2 = 0.609), and (c) RPE was higher in the third round than in the first one (14.6 ± 2.7 vs. 12.9 ± 3.0, respectively, P = 0.047, η2 = 0.457), but not than the second round (14.2 ± 2.5). No change was observed in right (Figure 5) and left handgrip muscle strength (Figure 6) from pre- to post-combat.
Rate of Perceived Exertion (RPE) in Each Round of the Simulated Combat with Standing (Triangle) or Sitting Recovery (Circle)
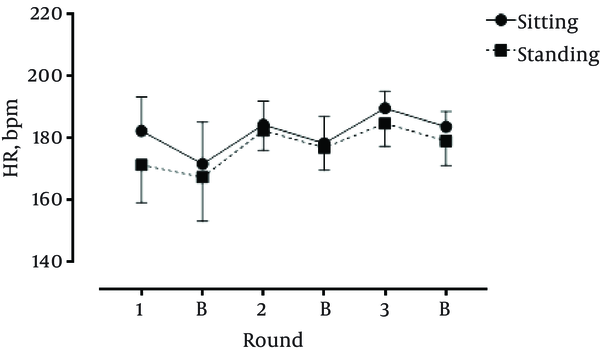
Heart Rate (HR) in Each Round and Break (B) of the Simulated Combat with Standing (Triangle) or Sitting Recovery (Circle)
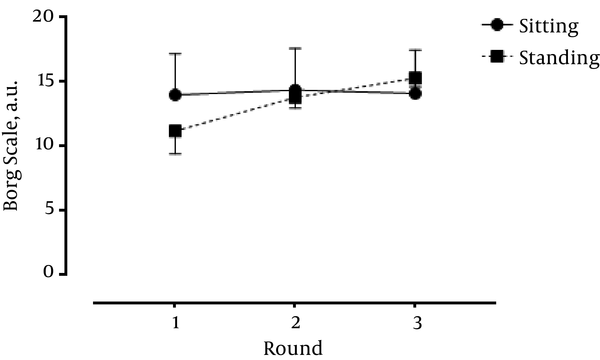
Changes from Pre- to Post-Combat in Countermovement Jump (CMJ) with Sitting (A) and Standing (B) Body Position During Break
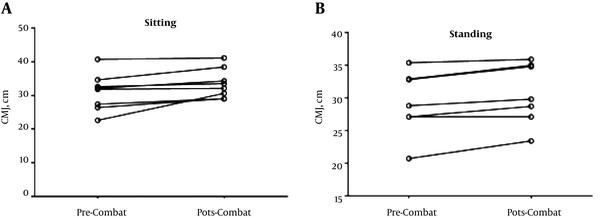
Changes from Pre- to Post-Combat in Right Handgrip Muscle Strength with Sitting (A) and Standing (B) Body Position During Break
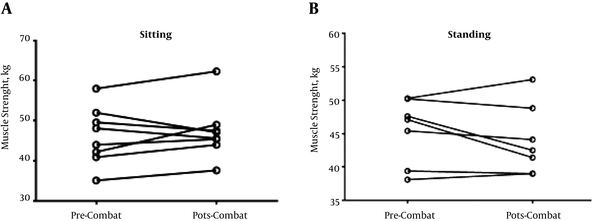
Changes from Pre- to Post-Combat in Left Handgrip Muscle Strength with Sitting (A) and Standing (B) Body Position During Break
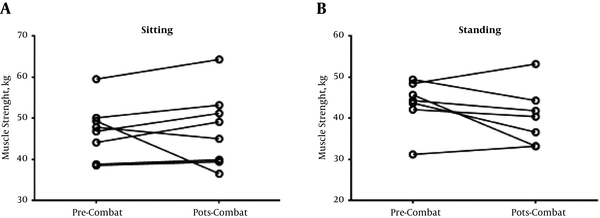
4. Discussion
This study analysed the effects of two different recovery conditions during breaks in boxing. No significant statistical differences between sitting and standing positions were found for the HR and RPE variables, although a large effect size verified in RPE. Significant statistical differences were found between pre and post simulated boxing combat for the HR responses, RPE and lower limb power.
First, the findings of the present study with regards to HR (mean value > 180 bpm) and RPE (mean value ~ 14 in Borg scale) confirmed the energetic profile of boxing. Previous research has indicated that the high-intensity activities in boxing implied a strong participation of the glycolytic system to supply the energy during the three minutes of each round (11). The consequence of such high anaerobic participation was the accumulation of blood lactate, achieving in some cases values between 12 and 14 mmol/L (2). Even the specific conditioning exercises in training sessions revealed a high participation of the glycolytic system, in which regularly observed values were between 9 and 12 mmol/L in sparring and bag punching (12-14). The intermittent nature of this sport (three bouts of three minutes with one minute of rest) implied optimal recovery between bouts and conditioning to achieve the best performance during the rounds. Accordingly, some studies (2, 15) suggested that individual anaerobic threshold and maximal oxygen consumption were related to boxing performance.
The results obtained from HR responses and RPE responses did not reveal statistical differences between positions. Nevertheless, the large effect size obtained in RPE suggested the greater perception of effort in standing position in comparison with sitting. Therefore, recovery in standing position might maximize the perception of fatigue that comes from the round of boxing. The role of a great aerobic capacity was to delay the onset of fatigue by increasing the tolerance to the lactate (shift to the right in the lactate curve) and accelerating the recovery between bouts (12). Based on that, the recovery was a crucial moment to prepare for another round and to decrease some physiological indicators associated with fatigue effect (volume of oxygen consumption, HR and blood lactate concentration). In fact, the perception of environment and the competition might constrain the values obtained. In a study that compared the same training session in two different conditions (in field and in laboratory), it was reported that boxers had greater values of HR and blood lactate concentrations in field (12).
The HR responses and RPE were also monitored during the simulated boxing combat. HR values statistically increased from round to round. As previously discussed, the glycolytic system was highly activated during the activity (2). For that reason, larger values of blood lactate concentration, deficit of oxygen and HR were achieved in this activity (16). Nevertheless, the small period of recovery/rest did not allow the balance the physiological impact of this high-intensity exercise (17). Based on that, it was possible to observe the accumulated effect of fatigue from round to round. Our results also showed that during the recovery periods the HR average was greater in round 2 and 3 than in round 1. This observation was in agreement with previous research, where the small period of recovery and the demanding of exercise led to an increase in HR and volume of oxygen uptake as a function of round (17).
The lower limbs power was also tested before and after the simulated boxing combat. Previous studies in combat sports (kickboxing) analysed the effect of the combat on muscle power by testing the squat jump (SJ) and CMJ (18). In that study, a decrease of performance in both jumps after the kickboxing match was observed. Surprisingly, our results found the opposite; the performance in CMJ was better after the boxing combat. This might be explained by the specificity of each activity. The boxing exercise was focused in the upper body activity, whereas kickboxing demanded a high participation of lower and upper body. This inequality of muscular participation might lead to heterogeneity with regards to lactate concentration in muscles during dynamic exercise (19). In the case of boxing, the decrease of pH and the associated reactions might be not so great than in kickboxing and for that reason the power performance was not conditioned.
A limitation of the present study was the sample characteristics (performance, level and size). The participants were amateur boxers, thus the findings should be generalized with caution to other performance level groups. Moreover, the present study was a pilot and used a relatively small number of participants that did not allow finding statistically significance results despite the large effect sizes. On the other side, the strength of the study was its novelty as it was the first that tested the physiological effects of two recovery methods (sitting and standing) in boxing. The results suggest that the sitting position may contribute to decrease in the perception of exertion and contribute to decrease in the overall perception of fatigue. Moreover, it was also verified that the predominant activity above the ventilatory threshold contributed to a progressive increase of HR from round to round, thus also contributing to a great perception of fatigue in the last rounds.
4.1. Conclusions
Although the present study did not provide evidence for different physiological impacts of body position during break in simulated boxing combat, there were indications that manipulating the body position might result in different perception of effort. This information might have practical value for coaches and trainers working with boxers to optimize training load.
Acknowledgements
References
-
1.
Chaabene H, Tabben M, Mkaouer B, Franchini E, Negra Y, Hammami M, et al. Amateur boxing: physical and physiological attributes. Sports Med. 2015;45(3):337-52. [PubMed ID: 25358529]. https://doi.org/10.1007/s40279-014-0274-7.
-
2.
Guidetti L, Musulin A, Baldari C. Physiological factors in middleweight boxing performance. J Sports Med Phys Fitness. 2002;42(3):309-14. [PubMed ID: 12094121].
-
3.
Tasiopoulos IG, Tripolitsioti A, Skordis D, Nikolaidis PT. The greater the number of wins the greater the peak torque levels of shoulder internal rotators power of dominant hand in amateur boxing athletes. Biol Exerci. 2015;11(1):65-7. https://doi.org/10.4127/jbe.2015.0086.
-
4.
Hanon C, Savarino J, Thomas C. Blood lactate and acid-base balance of world-class amateur boxers after three 3-minute rounds in international competition. J Strength Cond Res. 2015;29(4):942-6. [PubMed ID: 25330081]. https://doi.org/10.1519/JSC.0000000000000736.
-
5.
Nikolaidis PT, Chtourou H, Torres-Luque G, Tasiopoulos IG, Heller J, Padulo J. Effect of a Six-Week Preparation Period on Acute Physiological Responses to a Simulated Combat in Young National-Level Taekwondo Athletes. J Hum Kinet. 2015;47:115-25. [PubMed ID: 26557196]. https://doi.org/10.1515/hukin-2015-0067.
-
6.
Tassiopoulos I, Nikolaidis PT. Acute effect of official kickboxing game on handgrip muscle strength: winners vs. losers. J Phys Educ Sport. 2013;13(2):266.
-
7.
Giovani D, Nikolaidis PT. Differences in Force-velocity Characteristics of Upper and Lower Limbs of Non-competitive Male Boxers. Int J Exerc Sci. 2012;5(2):106-13. [PubMed ID: 27182379].
-
8.
Espana-Romero V, Ortega FB, Vicente-Rodriguez G, Artero EG, Rey JP, Ruiz JR. Elbow position affects handgrip strength in adolescents: validity and reliability of Jamar, DynEx, and TKK dynamometers. J Strength Cond Res. 2010;24(1):272-7. [PubMed ID: 19966590]. https://doi.org/10.1519/JSC.0b013e3181b296a5.
-
9.
Markovic G, Dizdar D, Jukic I, Cardinale M. Reliability and factorial validity of squat and countermovement jump tests. J Strength Cond Res. 2004;18(3):551-5. [PubMed ID: 15320660]. https://doi.org/10.1519/1533-4287(2004)18<551:RAFVOS>2.0.CO;2.
-
10.
Cohen J. Statistical power analysis for the behavioral sciences (revised ed.). New York: Academic Press; 1977.
-
11.
Smith MS. Physiological profile of senior and junior England international amateur boxers. J Sports Sci Med. 2006;5(CSSI):74-89. [PubMed ID: 24357979].
-
12.
Arseneau E, Mekary S, Leger LA. VO(2) requirements of boxing exercises. J Strength Cond Res. 2011;25(2):348-59. [PubMed ID: 21217532]. https://doi.org/10.1519/JSC.0b013e3181ef64cb.
-
13.
Chatterjee P, Banerjee AK, Majumdar P, Chatterjee P. Oxygen Consumption, Heart Rate and Blood Lactate Response during Sparring on Indian Women Boxers. Int J Appl Sports Sci. 2005;17(2).
-
14.
Ghosh AK, Goswami A, Ahuja A. Heart rate & blood lactate response in amateur competitive boxing. Indian J Med Res. 1995;102:179-83. [PubMed ID: 8543363].
-
15.
Khanna GL, Manna I. Study of physiological profile of Indian boxers. J Sports Sci Med. 2006;5(CSSI):90-8. [PubMed ID: 24357980].
-
16.
Davis P, Leithauser RM, Beneke R. The energetics of semicontact 3 x 2-min amateur boxing. Int J Sports Physiol Perform. 2014;9(2):233-9. [PubMed ID: 24572964]. https://doi.org/10.1123/IJSPP.2013-0006.
-
17.
de Lira CA, Peixinho-Pena LF, Vancini RL, de Freitas Guina Fachina RJ, de Almeida AA, Andrade Mdos S, et al. Heart rate response during a simulated Olympic boxing match is predominantly above ventilatory threshold 2: a cross sectional study. Open Access J Sports Med. 2013;4:175-82. [PubMed ID: 24379723]. https://doi.org/10.2147/OAJSM.S44807.
-
18.
Ouergui I, Hammouda O, Chtourou H, Zarrouk N, Rebai H, Chaouachi A. Anaerobic upper and lower body power measurements and perception of fatigue during a kick boxing match. J Sports Med Phys Fitness. 2013;53(5):455-60. [PubMed ID: 23903524].
-
19.
Moxnes JF, Sandbakk O. The kinetics of lactate production and removal during whole-body exercise. Theor Biol Med Model. 2012;9:7. [PubMed ID: 22413898]. https://doi.org/10.1186/1742-4682-9-7.