Abstract
Background:
Exercise training (ET) has a cardioprotective effect and can induce new cardiomyocyte formation in physiological cardiac remodeling. However, following a large myocardial infarction (MI), the changes in cardiac regeneration and the subsequently different intensities of ET remain unexplored. We investigated the effect of different intensities of ET on the expression of C/EBPβ and CITED4 after an MI.Methods:
Adult male Wistar rats were exposed to left coronary artery ligation (LAD). Following an echocardiographic evaluation at four weeks after surgery, MI-operated animals were randomly assigned to either the MI-sedentary (MI-Sed) or the MI-trained groups (low intensity interval training (LIIT), moderate intensity interval training (MIIT), and high intensity interval training (HIIT)). MI-trained animals performed six weeks ET protocols (five days/week).Results:
Ten weeks after surgery, HIIT and MIIT animals had significantly higher LV ejection fraction compared to MI-Sed animals. HIIT animals had significantly diminished mRNA levels of C/EBPβ compared to MI-Sed. The mRNA level of CITED4 in HIIT and MIIT were significantly high compared to MI-Sed.Conclusions:
The present study demonstrates that cardiac functions are ET intensity-dependent, following a MI. It seems that higher intensities of ET are most effective agents in promoting the expression of C/EBPβ and CITED4. Thus, ET has emerged as an important variable in preclinical and clinical investigations.Keywords
Myocardial Infarction Exercise Training Intensity C/EBPβ and CITED4
1. Background
Cardiovascular diseases (CVD) are the main cause of death in developed communities (1, 2). The exceedingly terrible clinical situation of CVD is represented by an intense myocardial infarction (MI) (3, 4). MI brings about a huge loss of cardiomyocytes and increases fibrocytes proliferation resulting in scar tissue formation and cardiac disorders (3). A few years ago, the idea that the heart is a post-mitotic organ with no regenerative limit had grabbed attention (5, 6). Nonetheless, information from animal models and human examinations recommended that the heart additionally has a constrained ability to produce new cardiomyocytes from ancestor cells and existing cardiomyocytes (7, 8). In fact, portions of the cardiomyocytes are supplanted within a typical human longevity (9, 10).
Exercise is a well confirmed intercession for the prevention and treatment of CVD (7, 11). Exercise applies its advantageous impacts by decreasing cardiovascular risk factors, and influencing the cellular and molecular remodeling of the heart (11, 12). Generally, increment in cardiomyocyte size is probably the central mechanism of exercise actuated cardiovascular development (11). Of late, it has been demonstrated that exercise training (ET) determines cardiac growth through the generation of new cardiomyocytes (13-17). It has been clinically proven that exercise is useful in averting and treating CVD. Past investigations have shown that ET could decrease the unfavorable remodeling of the harmed heart and enhance cardiac performance following a MI (12, 13).
The importance of the intensity (MIIT & HIIT) of exercise and its relationship with cardiovascular effects has been investigated in many studies (14-17). Still, the optimal dosage, intensity, frequency, and duration of exercise have remained unclear. Although a few weeks of vigorous ET can significantly augment cardiomyocyte formation (18), alternations in cardiac regeneration capacity following various intensities of ET after MI are yet to be elucidated.
2. Objectives
In this study, we have investigated the effects of low, moderate, and high intensity of ET on cardiac regeneration markers (C/EBPβ and CITED4) after a MI.
3. Methods
3.1. Study Design and Animals
Fifty-five adult male Wistar rats (230 g – 300 g ~ 10 weeks of age) were utilized for this investigation. Food and water were accessible ad libitum. All animal procedures were performed in accordance with the ethics committee of Rajaie Cardiovascular Medical and Research Center (RHC.AC.IR.REC.1393.28). The investigation conformed to the guide for the care and use of laboratory animals published by the United States National Institutes of Health (NIH Publication, 8th edition, 2011).
3.2. Coronary Artery Ligation Procedure
Rats were orally intubated with a 16-gauge intravenous catheter in the wake of being anesthetized with intraperitoneal infusion of a blend of ketamine (75 mg/kg) and xylazine (5 mg/kg). A positive pressure ventilation was kept up with room air and oxygen utilizing a Harvard rodent ventilator (model 683, USA). The site of operation was shaved, and then disinfected with either Betadine or alcohol. Once the animal was unresponsive to toe-pinch, left-sided thoracotomy was performed and the left anterior descending coronary artery (LAD) was for all time ligated utilizing a 6 - 0 prolene (Ethicon Inc., USA) suture. Legitimate ligation of the LAD was affirmed by watching the whitening of the myocardial tissue distal to the suture and dyskinesia of the anterior wall (19). Sham-operated rats experienced a similar operation without coronary artery ligation to serve as the control group (Sham; n = 6). At the end of the operation, cefazolin (25 mg/kg IM), flunixin (1 - 2 mg/kg IM), and warm sterile saline (0.5 - 1 mL, SC) were infused and the rats were left on the warming cushion until the effect of anesthesia wore off. Postoperative care was kept utilizing analgesia and hemodynamic monitoring for 24 h (1, 20).
3.3. Echocardiographic Measurements
Transthoracic echocardiography was performed using a VIVID 7 dimension system (General Electric-Vingmed Ultrasound, Horten Norway), equipped with a 12-MHz electronic transducer.
Echocardiographic assessments were performed a month after surgery and towards the end of the examination protocols. Under a similar anesthesia as for the infarct surgery which was kept up all through the echocardiographic examination, echocardiographic parameters had been recorded according with the American Society of Echocardiography guidelines. Left parasternal short-axis views of the left ventricle at the level of the papillary muscles was used to measure the following parameters: Left ventricular end-diastolic diameter (LVEDD), left ventricular end-systolic diameter (LVESD), posterior wall thickness in diastole (PWTd) and posterior wall thickness in systolic (PWTs). LV ejection fraction (EF) and fractional shortening (FS), was calculated according to the following formulas (21, 22):


Echocardiographic appraisal was used a month after surgery. This was to assess the degree of MI and measure the cardiac contractile performance before initiation of further experimental procedures. Rats with high level of myocardial harm and created MI, assessed at FS ≤ 35%, were incorporated into the examination (23).
3.4. Training Protocol
Out of 55 MI-operated rats, 25 rats which were survived from MI met the inclusion criteria as training protocol. The rats were assigned randomly to MI-trained groups high intensity interval training (HIIT, n = 6), moderate intensity interval training (MIIT, n = 6), and low intensity interval training (LIIT, n = 6). Familiarization with the motorized treadmill (Iranian Danesh Salar Company, Iran) was performed for MI-trained groups in the third and fourth week after surgery by walking for 5 m/min at slow pace five days per week for 5 min/day. The animals had 2 days rest before taking maximal oxygen consumption (VO2max). VO2max for each rat was resolved utilizing a maximal exercise test. VO2max measured and applied at maximal stress test to evaluate the running speed of each rat (24). High, medium and low intensity interval training rats were exercised up to 70 min, five days a week. The training protocol included 10 minutes of warm-up (40% - 50% VO2max) and 60 minutes of interval training (25, 26). Four minutes running protocol was assessed with different intensities such as HIIT (85% - 90%), MIIT (65% - 70%) and LIIT (55% - 60%) of measured VO2max, and 2 minutes of active recovery between each interval HIIT and MIIT (50% - 60%) and LIIT (45% - 50%) of measured VO2max. No ET was gained by MI-Sed and Sham animals during this experiment procedure (25, 26). In previous studies the relationship between maximum VO2 and running speed in similar post-MI rat models was varied for every week (24). In particular, the running speed was expanded continuously for six weeks of training by 0.02 m/s every week (25). Treadmill inclination during training and testing was 0º (26).
3.5. Quantitative RT PCR (qRT-PCR)
The total RNA isolated from cardiac tissue was routinely used for reverse transcription polymerase chain reaction (RT-PCR) or quantitative real-time PCR (qPCR). The total RNA was extracted using EasyPure RNA Kit (TransGen Biotech, China) following the manufacturer’s instructions. For RT-PCR, the cDNAs were synthesized using TransScript First-Strand cDNA Synthesis SuperMix (TransGen Biotech, China) with total RNA as templates. qRT-PCR was performed using TransStart Top Green qPCR SuperMix (TransGen Biotech, China) on Rotor-Gene 6000 system (Corbett, Concorde, NSW, Australia). The PCR-reaction mixtures contained 5 μL TransStart Top Green qPCR SuperMix, 1 μL of template cDNA, 1 μL forward and reverse primers, and 3 μL of sterile distilled water. The thermal cycling conditions comprised an initial denaturation step at 95°C for 2 min, followed by 40 cycles of 95°C for 10 s, 60°C for 30 s. β-actin was used for normalization and 2-ΔΔCt method was applied for the evaluated mRNA expression of the target gene (27) All the reactions were carried out in triplicate. All evaluations were performed by specialists who were unaware of the group assignment. Primer sequences (forward and reverse) are as follows: Cbp/p300-interacting transactivator, with Glu/Asp-rich carboxy-terminal domain, 4 (CITED4), acgagggtggttttgcagtct and caactcagccagacagaggaa; CCAAT/enhancer-binding protein beta (C/EBPβ), caagctgagcgacgagtacaa and acagctgctccaccttcttct; and β-actin, cggtcaggtcatcactatcgg and atgccacaggattccataccca.
3.6. Statistical Analysis
All results are expressed as mean ± SEM. The significant differences between the groups were determined by the one way analysis of variance and unpaired t-tests using the SPSS software version 19. The Bonferroni post-hoc analysis was utilized to find the differences. Significance was set at P < 0.05.
4. Results
Echocardiographic data - from Sham and MI-operated animals at four and 10 weeks after surgery - are presented in Table 1. Four weeks after surgery, we compared echocardiographic data between Sham and MI-operated animals. This comparison showed that fractional shortening, ejection fraction, IVSD, and LVPWs decreased dramatically four weeks after MI induction. In contrast, LVIDs increased significantly in MI-operated compare with Sham (P < 0.05). As found in Table 1, and additionally outlined in Figure 1A, In comparison with sham group, an enlarged ventricular cavity and thinned myocardium was seen in MI-operated group. Ten weeks after surgery, ejection fraction in HIIT and MIIT animals and fractional shortening in HIIT animals increased significantly compared to MI-sed animals. In addition, MI-operated animals (MI-sed, LIIT, MIIT, and HIIT) had significantly lower ejection fraction and fractional shortening compared to Sham animals. Also, LVIDd in HIIT animals and LVIDs in MI-sed, LIIT, and HIIT animals increased significantly compared to MI-sed animals (P < 0.05). As shown in Figure 1B, HIIT exercise-trained group showed the greatest increases in ejection fraction and fractional shortening after MI. These progressions were joined by a 32% and 53% increase in ejection fraction and fractional shortening compared to MI-sed, respectively (Table 1).
Left ventricular M-mode echocardiographic recordings at four and 10 weeks after surgery. A, Samples of m mode echocardiography in Sham-operated and MI-operated (MI-sed, LIIT, MIIT) animals. B, Left ventricular fractional shortening at 4 and 10 weeks after surgery. C, Left ventricular ejection fraction at 4 and 10 weeks after surgery. * P < 0.05 vs. MI-sed and MI-trained, # P < 0.05 vs. MI-sed.
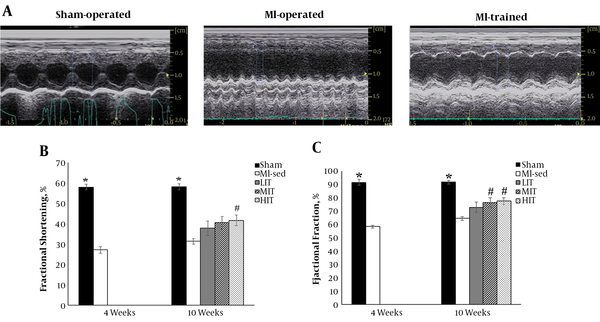
mRNA level of indicated genes from left ventricular at 10 weeks after surgery in Sham-operated and MI-operated (MI-sed, LIIT, MIIT and HIIT) animals. * P < 0.05 vs MI-sed. n = 6 animals per group.
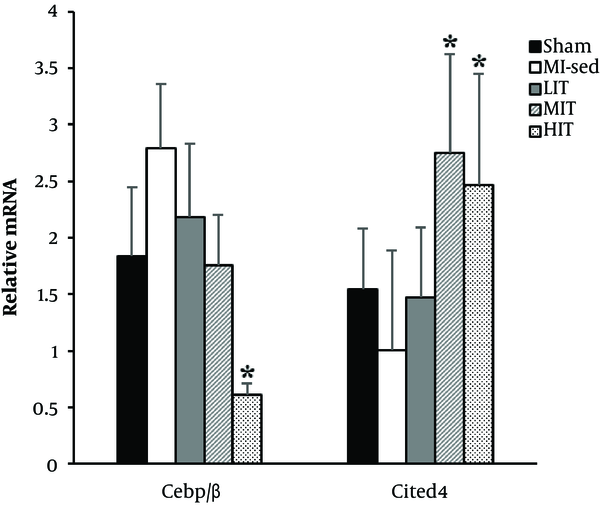
Sham, N = 6 | MI-Operated, N = 24 | ||||
---|---|---|---|---|---|
Four Weeks After Surgery | |||||
IVSD (cm) | 0.17 ± 0.04 | 0.14 ± 0.03b | |||
IVSS (cm) | 0.27 ±. 0.04 | 0.20 ± 0.04 | |||
LVIDd (cm) | 0.50 ± 0.11 | 0.55 ± 0.09 | |||
LVIDs (cm) | 0.22 ± 0.07 | 0.40 ± 0.07b | |||
LVPWd (cm) | 0.15 ± 0.05 | 0.17 ± 0.17 | |||
LVPWs (cm) | 0.23 ± 0.04 | 0.19 ± 0.03b | |||
EF (%) | 90.49 ± 2.44 | 58.30 ± 10.79b | |||
FS (%) | 55.40 ± 3.94 | 27.06 ± 6.58b | |||
Sham, N = 6 | MI-Sed, N = 6 | LIIT, N = 5 | MIIT, N = 6 | HIIT, N = 6 | |
10 Weeks After Surgery | |||||
IVSD (cm) | 0.17 ± 0.02 | 0.16 ± 0.02 | 0.15 ± 0.02 | 0.18 ± 0.03 | 0.15 ± 0.02 |
IVSS (cm) | 0.27 ± 0.03 | 0.22 ± 0.02 | 0.26 ± 0.05 | 0.21 ± 0.02 | 0.26 ± 0.05 |
LVIDd (cm) | 0.51 ± 0.11 | 0.57 ± 0.08 | 0.63 ± 0.07 | 0.53 ± 0.10 | 0.67 ± 0.08b |
LVIDs (cm) | 0.22 ± 0.08 | 0.39 ± 0.05b | 0.39 ± 0.08b | 0.32 ± 0.09 | 0.39 ± 0.07b |
LVPWd (cm) | 0.15 ± 0.04 | 0.17 ± 0.02 | 0.14 ± 0.02 | 0.14 ± 0.04 | 0.16 ± 0.02 |
LVPWs (cm) | 0.22 ± 0.04 | 0.22 ± 0.03 | 0.21 ± 0.01 | 0.19 ± 0.07 | 0.27 ± 0.04 |
EF (%) | 91.59 ± 3.30 | 64.48 ± 3.69b | 72.87 ± 8.95b | 76.41 ± 8.85b,c | 77.46 ± 7.02b,c |
FS (%) | 56.44 ± 3.74 | 31.32 ± 3.46b | 37.72 ± 8.11b | 40.45 ± 7.60b | 41.62 ± 6.84b,c |
In the present study, we have evaluated the expression of mRNA levels of the CITED4 and C/EBPβ genes. These genes are known to induce and inhibit cardiomyocyte proliferation (CITED4 and C/EBPβ) following exercise. HIIT animals significantly diminished mRNA levels C/EBPβ compared to MI-Sed. The mRNA level of CITED4 in HIIT and MIIT was significantly more compared to MI-Sed.
5. Discussion
The present study demonstrates that both moderate intensity- (MIIT, 65% - 70% of VO2max) and high intensity (HIIT, 85% - 90% of VO2max)- ET for up to six weeks can improve cardiac function capacity as measured by ejection fraction and fractional shortening in infarcted heart. As predicted, the HIIT exercise-trained group demonstrated the highest increase. Also six weeks of ET significantly up- regulated mRNA levels of CITED4 and down-regulated mRNA level of C/EBPβ. Collectively, data from this study suggests that higher intensities of ET are the most effective agents in increasing cardiac function and regeneration capacity in an infarcted heart.
Growing evidences have demonstrated that ET usefully adjusts the clinical course of post MI myocardial remodeling and enhances cardiovascular capacity (28, 29). Post-MI training may be associated with weakened ventricular hypertrophy and contractile decay, increased maximal cardiac output, ameliorated fibrosis, and an improvement in the quality of life in these patients (28, 30). Our study also confirmed previous findings that ET for up to six weeks improved cardiac function and cardiac regeneration capacity markers.
Exercise applies its gainful impacts by not simply decreasing the weight of established cardiovascular risk factors, but also straightforwardly influencing the cellular and molecular structure and performance of the heart (11, 18). Various studies demonstrated that ET may stimulate proliferation of preexisting cardiomyocytes and resident cardiac stem cells in vivo (11, 18, 26, 31, 32). Recently, a study proposed that four weeks of ET on myocardial infarcted rats stimulates cardiomyocytes proliferation, hinders cardiomyocytes apoptosis, and enhances cardiac function and remodeling (33).
In confirming these results, our study indicated that six weeks of ET induced markers of CITED4 and C/EBPβ. Also, our result demonstrated that higher intensity of ET (MIIT and HIIT) leads to further up and down-regulation markers of CITED4 and C/EBPβ compared to MI-sed. These results were similar to Waring’s et al. study, which showed high intensity exercise trained animals had the greatest number of newly formed cardiomyocytes after four weeks (26). Various mechanisms and signaling pathways regulate cardiac regeneration which is an intricate behavior (33). In this regard, Bostrom et al. reported that C/EBPβ stifles cardiomyocyte development and proliferation in the grown-up mammalian heart and decreases C/EBPβ in swim trained mice. This seems to be an important signal of physiological cardiomyocyte hypertrophy and proliferation (31). Our results demonstrated the down-regulation of mRNA expression of C/EBPβ in HIIT animals compared to MI-sed. The impact of exercise on cardiomyocyte hypertrophy and proliferation includes decrease in the expression of C/EBPβ, and a connected increment in the expression of CITED4. Down-regulation of C/EBPβ, in turn, releases serum response factor (SRF) to bind target gene promotors. They are also involved in the activation of an exercise gene set (GATA-4, Tbx5, Nkx2.5, and Mef2c), and ultimately cardiac growth. According to results of previous and present studies, the reduction in C/EBPβ expression is sufficient to improve cardiac function and up-regulation of cardiomyocyte hypertrophy and proliferation markers after MI (31). Taken together, these data indicate that higher intensities of ET-induced cardiac regeneration following MI.
5.1. Conclusions
In conclusion, we demonstrate that cardiovascular functions are ET intensity-dependent, following MI. It seems that higher intensities of ET are most effective agents in increasing cardiac regeneration markers (CITED4 and C/EBPβ) in MI rats. Therefore, ET intensity has emerged as a crucial variable in future preclinical and clinical studies.
Acknowledgements
References
-
1.
Samsamshariat SA, Samsamshariat ZA, Movahed MR. A novel method for safe and accurate left anterior descending coronary artery ligation for research in rats. Cardiovasc Revasc Med. 2005;6(3):121-3. [PubMed ID: 16275608]. https://doi.org/10.1016/j.carrev.2005.07.001.
-
2.
Towbin JA, Bowles NE. The failing heart. Nature. 2002;415(6868):227-33. [PubMed ID: 11805847]. https://doi.org/10.1038/415227a.
-
3.
Sharpe N. Cardiac remodeling in coronary artery disease. Am J Cardiol. 2004;93(9A):17B-20B. [PubMed ID: 15144932]. https://doi.org/10.1016/j.amjcard.2004.01.002.
-
4.
Mueller-Hennessen M, Sigl J, Fuhrmann JC, Witt H, Reszka R, Schmitz O, et al. Metabolic profiles in heart failure due to non-ischemic cardiomyopathy at rest and under exercise. ESC Heart Fail. 2017;4(2):178-89. [PubMed ID: 28451455]. [PubMed Central ID: PMC5396036]. https://doi.org/10.1002/ehf2.12133.
-
5.
Anversa P, Nadal-Ginard B. Myocyte renewal and ventricular remodelling. Nature. 2002;415(6868):240-3. [PubMed ID: 11805849]. https://doi.org/10.1038/415240a.
-
6.
Yutzey KE. Cardiomyocyte proliferation: Teaching an old dogma new tricks. Circ Res. 2017;120(4):627-9. [PubMed ID: 28209795]. [PubMed Central ID: PMC5319743]. https://doi.org/10.1161/CIRCRESAHA.116.310058.
-
7.
Ellison GM, Waring CD, Vicinanza C, Torella D. Physiological cardiac remodelling in response to endurance exercise training: Cellular and molecular mechanisms. Heart. 2012;98(1):5-10. [PubMed ID: 21880653]. https://doi.org/10.1136/heartjnl-2011-300639.
-
8.
Abdelwahid E, Kalvelyte A, Stulpinas A, de Carvalho KA, Guarita-Souza LC, Foldes G. Stem cell death and survival in heart regeneration and repair. Apoptosis. 2016;21(3):252-68. [PubMed ID: 26687129]. [PubMed Central ID: PMC5200890]. https://doi.org/10.1007/s10495-015-1203-4.
-
9.
Rosenzweig A. Medicine. Cardiac regeneration. Science. 2012;338(6114):1549-50. [PubMed ID: 23258880]. https://doi.org/10.1126/science.1228951.
-
10.
Senyo SE, Steinhauser ML, Pizzimenti CL, Yang VK, Cai L, Wang M, et al. Mammalian heart renewal by pre-existing cardiomyocytes. Nature. 2013;493(7432):433-6. [PubMed ID: 23222518]. [PubMed Central ID: PMC3548046]. https://doi.org/10.1038/nature11682.
-
11.
Mann N, Rosenzweig A. Can exercise teach us how to treat heart disease? Circulation. 2012;126(22):2625-35. [PubMed ID: 23184282]. [PubMed Central ID: PMC4000019]. https://doi.org/10.1161/CIRCULATIONAHA.111.060376.
-
12.
Haykowsky M, Scott J, Esch B, Schopflocher D, Myers J, Paterson I, et al. A meta-analysis of the effects of exercise training on left ventricular remodeling following myocardial infarction: start early and go longer for greatest exercise benefits on remodeling. Trials. 2011;12:92. [PubMed ID: 21463531]. [PubMed Central ID: PMC3083361]. https://doi.org/10.1186/1745-6215-12-92.
-
13.
Haykowsky MJ, Liang Y, Pechter D, Jones LW, McAlister FA, Clark AM. A meta-analysis of the effect of exercise training on left ventricular remodeling in heart failure patients: the benefit depends on the type of training performed. J Am Coll Cardiol. 2007;49(24):2329-36. [PubMed ID: 17572248]. https://doi.org/10.1016/j.jacc.2007.02.055.
-
14.
Weston KS, Wisloff U, Coombes JS. High-intensity interval training in patients with lifestyle-induced cardiometabolic disease: A systematic review and meta-analysis. Br J Sports Med. 2014;48(16):1227-34. [PubMed ID: 24144531]. https://doi.org/10.1136/bjsports-2013-092576.
-
15.
Weston M, Taylor KL, Batterham AM, Hopkins WG. Effects of low-volume high-intensity interval training (HIT) on fitness in adults: A meta-analysis of controlled and non-controlled trials. Sports Med. 2014;44(7):1005-17. [PubMed ID: 24743927]. [PubMed Central ID: PMC4072920]. https://doi.org/10.1007/s40279-014-0180-z.
-
16.
Angadi SS, Mookadam F, Lee CD, Tucker WJ, Haykowsky MJ, Gaesser GA. High-intensity interval training vs. moderate-intensity continuous exercise training in heart failure with preserved ejection fraction: A pilot study. J Appl Physiol (1985). 2015;119(6):753-8. [PubMed ID: 25190739]. https://doi.org/10.1152/japplphysiol.00518.2014.
-
17.
Ulbrich AZ, Angarten VG, Netto AS, Sties SW, Bündchen DC, de Mara LS, et al. Comparative effects of high intensity interval training versus moderate intensity continuous training on quality of life in patients with heart failure: Study protocol for a randomized controlled trial. Clin Trial Regul Sci Cardiol. 2016;13:21-8. https://doi.org/10.1016/j.ctrsc.2015.11.005.
-
18.
Xiao J, Xu T, Li J, Lv D, Chen P, Zhou Q, et al. Exercise-induced physiological hypertrophy initiates activation of cardiac progenitor cells. Int J Clin Exp Pathol. 2014;7(2):663-9. [PubMed ID: 24551287]. [PubMed Central ID: PMC3925911].
-
19.
Fukuda S, Kaga S, Sasaki H, Zhan L, Zhu L, Otani H, et al. Angiogenic signal triggered by ischemic stress induces myocardial repair in rat during chronic infarction. J Mol Cell Cardiol. 2004;36(4):547-59. [PubMed ID: 15081314]. https://doi.org/10.1016/j.yjmcc.2004.02.002.
-
20.
Virag JA, Lust RM. Coronary artery ligation and intramyocardial injection in a murine model of infarction. J Vis Exp. 2011;(52). [PubMed ID: 21673649]. [PubMed Central ID: PMC3197029]. https://doi.org/10.3791/2581.
-
21.
Rottman JN, Ni G, Brown M. Echocardiographic evaluation of ventricular function in mice. Echocardiography. 2007;24(1):83-9. [PubMed ID: 17214630]. https://doi.org/10.1111/j.1540-8175.2006.00356.x.
-
22.
Scheer P, Sverakova V, Doubek J, Janeckova K, Uhrikova I, Svoboda P. Basic values of M-mode echocardiographic parameters of the left ventricle in outbreed Wistar rats. Vet Med. 2012;57(1):42-52. https://doi.org/10.17221/4971-VETMED.
-
23.
Kemi OJ, Haram PM, Loennechen JP, Osnes JB, Skomedal T, Wisloff U, et al. Moderate vs. high exercise intensity: Differential effects on aerobic fitness, cardiomyocyte contractility, and endothelial function. Cardiovasc Res. 2005;67(1):161-72. [PubMed ID: 15949480]. https://doi.org/10.1016/j.cardiores.2005.03.010.
-
24.
Hoydal MA, Wisloff U, Kemi OJ, Ellingsen O. Running speed and maximal oxygen uptake in rats and mice: Practical implications for exercise training. Eur J Cardiovasc Prev Rehabil. 2007;14(6):753-60. [PubMed ID: 18043295]. https://doi.org/10.1097/HJR.0b013e3281eacef1.
-
25.
Kraljevic J, Marinovic J, Pravdic D, Zubin P, Dujic Z, Wisloff U, et al. Aerobic interval training attenuates remodelling and mitochondrial dysfunction in the post-infarction failing rat heart. Cardiovasc Res. 2013;99(1):55-64. [PubMed ID: 23554460]. https://doi.org/10.1093/cvr/cvt080.
-
26.
Waring CD, Vicinanza C, Papalamprou A, Smith AJ, Purushothaman S, Goldspink DF, et al. The adult heart responds to increased workload with physiologic hypertrophy, cardiac stem cell activation, and new myocyte formation. Eur Heart J. 2014;35(39):2722-31. [PubMed ID: 23100284]. [PubMed Central ID: PMC4196078]. https://doi.org/10.1093/eurheartj/ehs338.
-
27.
Peinnequin A, Mouret C, Birot O, Alonso A, Mathieu J, Clarencon D, et al. Rat pro-inflammatory cytokine and cytokine related mRNA quantification by real-time polymerase chain reaction using SYBR green. BMC Immunol. 2004;5:3. [PubMed ID: 15040812]. [PubMed Central ID: PMC373448]. https://doi.org/10.1186/1471-2172-5-3.
-
28.
Garza MA, Wason EA, Zhang JQ. Cardiac remodeling and physical training post myocardial infarction. World J Cardiol. 2015;7(2):52-64. [PubMed ID: 25717353]. [PubMed Central ID: PMC4325302]. https://doi.org/10.4330/wjc.v7.i2.52.
-
29.
Lawler PR, Filion KB, Eisenberg MJ. Efficacy of exercise-based cardiac rehabilitation post-myocardial infarction: A systematic review and meta-analysis of randomized controlled trials. Am Heart J. 2011;162(4):571-584 e2. [PubMed ID: 21982647]. https://doi.org/10.1016/j.ahj.2011.07.017.
-
30.
Dubach P, Myers J, Dziekan G, Goebbels U, Reinhart W, Vogt P, et al. Effect of exercise training on myocardial remodeling in patients with reduced left ventricular function after myocardial infarction: Application of magnetic resonance imaging. Circulation. 1997;95(8):2060-7. [PubMed ID: 9133516].
-
31.
Bostrom P, Mann N, Wu J, Quintero PA, Plovie ER, Panakova D, et al. C/EBPbeta controls exercise-induced cardiac growth and protects against pathological cardiac remodeling. Cell. 2010;143(7):1072-83. [PubMed ID: 21183071]. [PubMed Central ID: PMC3035164]. https://doi.org/10.1016/j.cell.2010.11.036.
-
32.
Leite CF, Lopes CS, Alves AC, Fuzaro CS, Silva MV, Oliveira LF, et al. Endogenous resident c-Kit cardiac stem cells increase in mice with an exercise-induced, physiologically hypertrophied heart. Stem Cell Res. 2015;15(1):151-64. [PubMed ID: 26070113]. https://doi.org/10.1016/j.scr.2015.05.011.
-
33.
Cai MX, Shi XC, Chen T, Tan ZN, Lin QQ, Du SJ, et al. Exercise training activates neuregulin 1/ErbB signaling and promotes cardiac repair in a rat myocardial infarction model. Life Sci. 2016;149:1-9. [PubMed ID: 26892146]. https://doi.org/10.1016/j.lfs.2016.02.055.