Abstract
Background:
The repeated sprint ability (RSA) has been studied with protocols using distances longer than 20 m per sprint, whereas basketball players cover on average less than 20-meter distance per sprint during match.Objectives:
The aim of the present study was to examine the physiological impact of 10 × 15 m RSA test in straight-line (RSASL) or with change of direction (RSACOD), i.e. 10 × (7.5 + 7.5 m)) in young national level basketball players.Patients and Methods:
Young basketball players (n = 11, age 17.1 (1.0) years, body mass 76 (6) kg, height 184 (4) cm, body mass index 22.6 (1.8) kgm-2, sport experience 6.9 (2.7) years, mean (standard deviation)) and a control group consisting of high-school athletes (n = 7, 16.1 (0.7) years, 67 (6) kg, 177 (6) cm, 21.5 (1.0) kgm-2, 7.7 (1.6) years, respectively) performed RSASL and RSACOD on a counter-balanced order. Sprints started every 30 seconds (active recovery) and there was 30 minutes break between RSA protocols; time variables were total time (TT), best time (BT) and fatigue index (FI). Countermovement jump (CMJ) was tested before and after each RSA protocol. Heart rate (HR) was continuously monitored during testing procedures.Results:
Compared with RSASL, TT and BT were worst in RSACOD (38.13 vs. 27.52 s and 3.67 vs. 2.66 s, P < 0.001, respectively), whereas FI did not differ (3.8 vs. 3.5%, P = 0.388). A 2 × 2 repeated measures ANOVA showed main effect of RSA on CMJ (pre-test vs. post-test, increase + 1.8 cm, P = 0.020, η2 = 0.28); there was neither main effect of RSA protocols (RSASL vs. RSACOD +0.7 cm, P = 0.251, η2 = 0.08) nor an interaction between pre-post measurements and RSA protocols (P = 0.578, η2 = 0.02). Compared with RSASL, RSACOD induced higher mean and peak HR responses (175 vs 172 bpm, P < 0.001, and 185 vs 182 bpm, P = 0.002, respectively). No statistical difference was observed between basketball players and control group neither for TT (27.98 vs. 26.80 seconds, + 4.4%, P = 0.149) and BT (2.71 vs. 2.59 seconds, + 4.5%, P = 0.157) in RSASL nor for TT (38.55 vs. 37.47 seconds, + 2.9%, P = 0.169) and BT (3.70 vs. 3.63 seconds, 1.8%, P = 0.414) in RSACOD.Conclusions:
In conclusion, RSASL and RSACOD differed for time variables (TT and BT) and HR responses. Despite being non-significant, the trend that adding COD reduced the percentage differences in TT and BT between the two groups highlighted the role of sport specialization.Keywords
1. Background
Basketball is a team sport, in which in addition to high intensity short movements, such as jumping and passing, basketball players perform also many repeated sprints during match. The ability to perform repeated sprints with minimal recovery (1) or the ability to produce the best possible average sprint performance over a series of sprints, separated by short recovery periods (2) has been defined as repeated sprint ability (RSA). Because of the importance of this ability for sport performance, several test protocols varying by sprint distance, number of sprints, duration, and mode of recovery among sprints and number and mode of change of direction (COD) have been developed to assess RSA in basketball players (3-8). The above-mentioned RSA test protocols have used sprint distance ranging from 20 (7) to 35 m (8). Such sprint distance, taking into account that the official dimensions of a playing court are 28 m in length and 15 m in width (9) and suggestions that an average sprint in basketball lasts less than 2 seconds (10), seemed too long. To the best of our knowledge, there was not any RSA test protocol using shorter distances than 20 minutes. The development of a test protocol using shorter distances (e.g. 15 m) than the existing protocols would have more face validity and reflect better the actual RSA of basketball players. In addition, less information was available about RSA during adolescence, especially using RSA protocols with COD. Moreover, the effect of sport specialization, i.e. whether athletes such as basketball players scored better in RSA exercise with COD than athletes who use smaller number or different patterns of COD in their sport, has not been studied yet.
2. Objectives
The aim of the present study was to examine the physiological impact of RSA 10 × 15 m straight-line (RSASL) or with change of direction (RSACOD, i.e. 10 × (7.5 + 7.5 m)) in young national level basketball players.
3. Patients and Methods
3.1. Study Design and Participants
Young basketball players (n = 11, age 17.1 (1.0) years, body mass 76 (6) kg, height 184 (4) cm, body mass index 22.6 (1.8) kgm-2, sport experience 6.9 (2.7) years, mean (standard deviation)) and a control group consisting of high-school athletes practicing soccer, tennis and triathlon (n = 7, 16.1 (0.7) years, 67 (6) kg, 177 (6) cm, 21.5 (1.0) kgm-2, 7.7 (1.6) years, respectively) volunteered to participate in the study. The participants were students attending an athletic high school of Piraeus, Greece. The present research was conducted in accordance with the Declaration of Helsinki (1964). All participants provided informed consent. This study was approved by the local institutional review board. Inclusion criteria were the absence of any injury or illness at the time of the study (February 2015). The participants were instructed not to eat for 2 hours before testing. Testing procedures were carried out on a weekday at 12 p.m. (temperature 20°C and humidity 62%)
3.2. Protocols and Equipment
In the beginning of the testing session, the participants performed 20 minutes warm-up consisting of 10 minutes jogging, 5 minutes stretching exercises and 5 minutes running drills (e.g. 15 m sprints with and without COD). After the warm-up, they were tested for countermovement jump (CMJ), performed randomly either RSASL or RSACOD and were re-tested for CMJ. Then, they rested for 30 minutes and performed on a counterbalanced order either RSACOD or RSASL with pretest and posttest measurement of CMJ. RSASL: The RSASL exercise consisted of 10 × 15 m sprints starting every 30 seconds (active recovery). The time for the 30 seconds sprint-recovery cycle was measured by a hand-held stopwatch; Seiko S056 (Seiko Sasaki, Tokyo, Japan). Participants were instructed to assume the ready position 5 seconds before starting the next sprint and were encouraged verbally in order to exert maximal effort in every trial. Each sprint was timed using a photocell system (Brower Timing Systems, Utah, USA) consisting of two pairs of photocells. The photocells were placed at belt height so that the legs do not break the light beam (according to manufacturer’s guidelines. The participants started each trial from a standing position 0.5 m behind the first pair of photocells. The following time variables were calculated: total time (TT), best time (BT) and fatigue index (FI). The FI was estimated using the Fitzsimons’ formula (11).

RSACOD: In the RSACOD exercise, the participants performed 10 × (7.5 + 7.5 m) sprints starting every 30 seconds (active recovery). Similar procedures and equipment as in the case of RSASL were used. The only difference in the set-up of the equipment was that only one pair of photocells was used, because the start line was also the finish line. CMJ was tested on an optical measurement system consisting of a transmitting and receiving bar (Optojump next, Microgate Engineering, Bolzano, Italy). This equipment calculated the height of CMJ based on flight time. In each occasion (pretest or posttest), two trials were performed and the best was recorded. Heart rate (HR) was continuously monitored (Polar team2, Finland) during testing procedures. Mean (HRmean) and peak HR (HRpeak) was recorded for each RSA test.
3.3. Statistical Analysis
The statistical package IBM SPSS v.20.0 (SPSS, Chicago, USA) was used to perform all statistical analyses. The dependent variables were tested for the assumption of normality using the Shapiro-Wilk test and visual inspection of normality plots. All data were presented as mean (standard deviation). Differences between groups were examined by independent t-test and effect size (ES) for statistical difference in this test was determined by the following criteria for Cohen’s d: d ≤ 0.2, trivial ES; 0.2 < d ≤ 0.6, small ES; 0.6 < d ≤ 1.2, moderate ES; 1.2 < d ≤ 2.0, large ES; and d > 2.0, very large ES (12). The relationship between RSASL and RSACOD with regards to TT, BT and FI was examined by Pearson moment correlation coefficient r, and its magnitude was evaluated as trivial (r ≤ 0.1), small (0.1 < r ≤ 0.3), moderate (0.3 < r ≤ 0.5), large (0.5 < r ≤ 0.7), very large (0.7 < r ≤ 0.9) and almost perfect (r > 0.9) (13). Pearson r was used to examine the relationship of the best pretest CMJ value with time variables of the two RSA exercises. A 2 × 2 repeated measures ANOVA was used to examine the effect of RSA format (RSASL vs. RSACOD) and exercise (posttest vs. pretest) on CMJ. To evaluate the ES for differences in ANOVA the following criteria of eta squared were used: η2 = 0.01, small ES; η2 = 0.06, medium ES; η2 = 0.13, large ES (12). Significance level was set at alpha = 0.05.
4. Results
Compared with RSASL, TT and BT were worst in RSACOD (38.13 vs. 27.52 seconds and 3.67 vs. 2.66 seconds, P < 0.001, respectively), whereas FI did not differ (3.8 vs. 3.5%, P = 0.388). Very large correlations were observed between RSASL and RSACOD with regards to TT and BT, whereas the correlation for FI was moderate and non-significant (Figure 1). The best pretest CMJ value correlated inversely with TT and BT of RSA exercises and these correlations were of large to very large magnitude (Figure 2). Pretest CMJ was also correlated with FI of RSASL (r = 0.57, P = 0.014), but not with FI of RSACOD (r = -0.16, P = 0.535). The work-to-rest ratio was lower in RSASL (2.75:27.25 seconds, i.e. ~ 10%) than in RSACOD (3.81:26.19 seconds, i.e. ~ 15%). A 2 × 2 repeated measures ANOVA showed a main effect of RSA on CMJ (pre-test 34.8 cm vs. post-test 36.6 cm, increase +1.8 cm, P = 0.020, η2 = 0.28); there was neither a main effect of RSA protocols (RSASL vs. RSACOD +0.7 cm, P = 0.251, η2 = 0.08) nor an interaction between pre-post measurements and RSA protocols (P = 0.578, η2 = 0.02). Compared with RSASL, RSACOD induced higher mean and peak HR responses (175 vs 172 bpm, P < 0.001, and 185 vs 182 bpm, P = 0.002, respectively). Basketball players were older, taller and heavier than the control group (Table 1). No statistical difference was observed between basketball players and control group neither for TT (27.98 vs. 26.80 seconds, +4.4%, P = 0.149) and BT (2.71 vs. 2.59 seconds, +4.5%, P = 0.157) in RSASL nor for TT (38.55 vs. 37.47 seconds, +2.9%, P = 0.169) and BT (3.70 vs. 3.63 seconds, +1.8%, P = 0.414) in RSACOD (Table 2).
Relationship Between Repeated Sprint Ability Exercise in Straight Line (RSASL) and With Change of Direction (RSACOD) With Regards to Time Variables
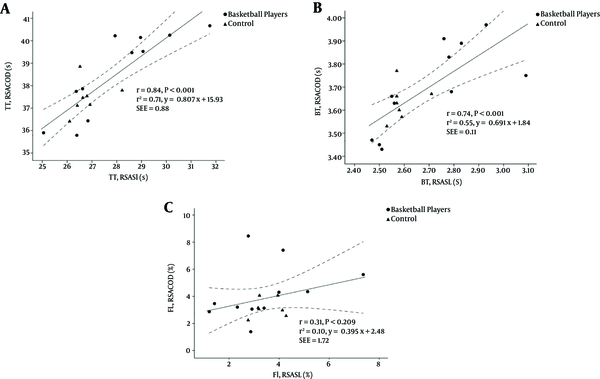
Relationship Between Countermovement Jump and Time Variables
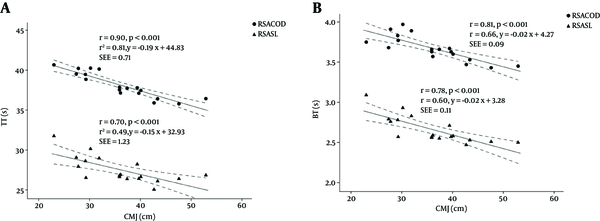
Training Experience and Anthropometric Characteristics of Participants by Sport Specialization
Basketball Players (n = 11) | Control (n = 7) | Mean Difference (90% CI) | |
---|---|---|---|
Age, y | 17.1 (1.0) | 16.1 (0.7)a | 1.1 (0.3;1.8) |
Body mass, kg | 76.5 (6.2) | 67.4 (5.7)b | 9.1 (4.0;14.2) |
Stature, cm | 184 (4) | 177 (6)a | 7 (3;11) |
BMI, kgm-2 | 22.6 (1.8) | 21.5 (1.0) | 1.1 (-0.2;2.4) |
Experience, y | 6.9 (2.7) | 7.7 (1.6) | −0.8 (-2.8;1.2) |
Training days, 1/wk | 3.6 (1.3) | 3.4 (1.1) | 0.2 (-0.8;1.2) |
Training volume, min/wk | 327 (116) | 313 (155) | 14 (-97;126) |
Official match, 1/wk | 0.9 (0.3) | 0.9 (0.4) | 0.1 (-0.2;0.3) |
Time Variables of the Repeated Sprint Ability Exercise Protocols by Sports Specialization
Basketball Players (n = 11) | Control (n = 7) | Mean Difference (90% CI) | |
---|---|---|---|
RSASL | |||
TT, s | 27.98 (1.96) | 26.80 (0.68) | 1.17 (-0.18;2.53) |
BT, s | 2.71 (0.20) | 2.59 (0.06) | 0.12 (-0.02;0.26) |
FI, No. (%) | 3.4 (1.7) | 3.5 (0.6) | -0.1 (-1.3;1.1) |
RSACOD | |||
TT, s | 38.55 (1.86) | 37.47 (0.75) | 1.07 (-0.23;2.38) |
BT, s | 3.70 (0.19) | 3.63 (0.08) | 0.06 (-0.07;0.20) |
FI, % | 4.3 (2.1) | 3.2 (0.7) | 1.1 (-0.3;2.6) |
5. Discussion
The main find of the present study was that A) RSASL and RSACOD differed for time variables (TT and BT) and HR responses with better performance for TT and BT in RSASL, and higher mean and peak HR in RSACOD, and B) the percentage differences in TT and BT between the two groups of adolescent athletes decreased from RSASL to RSACOD, although the latter exercise lasted longer. The worst (slower) performance in time variables (TT +38.6% and BT +38.0%) in RSACOD should be attributed to the fact that this exercise protocol included both acceleration and deceleration in order to change direction, whereas RSASL included only acceleration. When differences were examined between basketball players and controls, a trend of worst performance in TT and BT for both RSA exercise protocols in basketball players than in controls was observed. Basketball players were worst in TT and BT in RSASL than in controls (mean difference +1.18 seconds and +0.12 seconds, respectively) and it would be expected that since RSACOD lasted longer the difference between the two groups would increase. Furthermore, adding a COD, i.e. a trajectory with turn of 180 degrees angle would be expected to benefit the shorter group (in the present study, the shorter group was the control group) (6), since the large anthropometric dimensions of basketball players might not facilitate such turns. Surprisingly, the respective differences in RSACOD were +1.08 seconds and +0.07 seconds), i.e. basketball players reduced their difference from the control group. An explanation for this trend might be the sport specialization, according to which basketball players relatively improved their performance in the tasks with COD, because they use mostly sprints with COD during training and playing. With regards to the average work to rest ratio in the two RSA exercises, it was 1/10 in RSASL and 1/7 in RSACOD. This variation in the work to rest ratio was due to the longer duration of a sprint with COD than of that without COD resulting in shorter rest time in the former than the latter given that in both exercises sprints started every 30 seconds. The different work-to-rest ratio in the two exercises influenced the metabolism, because it has been shown that a shorter rest time between repeated sprints might result in smaller restoration of phosphocreatine stores (2). Despite the different work-to-rest ratio, the findings of the study did not show any difference in the decrease of performance (FI) between the two RSA exercise protocols. FI was ~ 3.5% for both protocols, which was in agreement with a previous study using a longer RSA protocol (10 × 15 + 15 m with 30 seconds passive recovery, exercise to rest ratio 1/5) (4) indicating that the rest duration was sufficient in order not to prevent further decreases in performance. The magnitude of the correlations between RSASL and RSACOD with regards to TT and BT was very large. This indicated that the two exercise protocols shared a large portion of variance for TT and BT (71% and 55%, respectively). This portion was larger for TT than for BT. An explanation for this discrepancy was that TT was the total score of exercise, ranging from ~ 27 s in RSASL to ~ 38 s in RSACOD. Since there was maximal effort in both exercise protocols, the large common portion of variance suggested that both protocols taxed similarly the energy transfer systems (i.e. alactic and lactic anaerobic metabolism) and running ability. On the other hand, in the case of BT, i.e. the best single performance, the effect of COD was more intense, because the duration of single trials was ~2.70 seconds in RSASL and ~3.70 seconds in RSACOD. To examine the effect of the two RSA exercise protocols on muscular fatigue, the participants performed a jumping test (CMJ) before and after each protocol. The findings showed an overall main effect of RSA exercise on CMJ, with posttest value being ~2 cm higher than pretest. This finding was not in agreement with previous studies that had used jumping tests (Abalakov test or CMJ) to examine the effect of an RSA test protocol (14) or small-sided basketball games (15) on muscular fatigue. In the first study, there was ~ 9% decrease in Abalakov test after an RSA test (6 × 35 m with 10 seconds) (14). This discrepancy should be attributed to the use of longer distance and shorter rest period compared to the present study. In the second study, CMJ decreased by ~ 2 cm after 3 × 3 game and increased by ~ 3 cm after a 4 × 4 game, which led the authors to conclude that the former game format was more intense and with greater physiological impact than the latter one (15). In the present study, the pretest CMJ had correlation with TT of larger magnitude in RSACOD than in RSASL, and the same trend was noticed for the correlation of CMJ with BT. This finding showed the additional muscular effort that was needed in the RSACOD. Since the two RSA exercises were novel, there were not any reference data to compare the scores of the participants in the present study. With regards to their jumping performance, CMJ in the basketball players (~ 35 cm) was lower than the values reported for Greek (38.6 cm) (16), Italian (39.9 cm) (4) and Tunisian basketball players of similar age (41.4 cm) (17), and adult basketball players (39.8 cm (3). The basketball players were taller and heavier than the control group. Stature (184 cm) was similar as that previously reported in 17 years (182 cm) (16) and in 16 years basketball players of elite Greek clubs (186 cm) (18), and lower than in 17 years select basketball players from the Dutch basketball academy (Netherlands) (190 cm) (6). Body mass (76.5 kg) was similar to that of 17 year Dutch basketball players (77.6 kg) (6), and lower than Greek basketball players (79.3 kg) (18). The larger body dimensions in basketball players than in the control group confirmed previous studies, which highlighted the importance of stature and body mass for performance in basketball (19, 20). RSA has been shown to be an important component of performance in basketball. Thus, coaches and fitness trainers who work with young basketball players should monitor regularly RSA and include repeated sprints in their training program. The findings of the present study provided reference data on time variables of two RSA exercises, and their impact on HR responses and muscular fatigue, and, consequently they would serve as a valuable tool for coaches and fitness trainers. A limitation of the present study was the small size of the control group. In addition, the control group consisted of athletes competing in different sports. Thus, the findings should be considered with caution taking into account these limitations. The present study focused on repeated sprints. However, considering the pattern of movements in basketball (many changes of directions, not only in forward-backward, but also in lateral directions), future studies should examine the physiological impact of agility protocols and the role of sport specialization.
5.1. Conclusions
In conclusion, RSASL and RSACOD differed for time variables (TT and BT) and HR responses, with better performance in time variables and lower values in HR for RSASL. Despite being non-significant, the trend that adding COD reduced the percentage differences in TT and BT between the two groups highlighted the role of sport specialization.
References
-
1.
Spencer M, Bishop D, Dawson B, Goodman C. Physiological and metabolic responses of repeated-sprint activities:specific to field-based team sports. Sports Med. 2005;35(12):1025-44. [PubMed ID: 16336007].
-
2.
Bishop D, Girard O, Mendez-Villanueva A. Repeated-sprint ability - part II: recommendations for training. Sports Med. 2011;41(9):741-56. [PubMed ID: 21846163]. https://doi.org/10.2165/11590560-000000000-00000.
-
3.
Stojanovic MD, Ostojic SM, Calleja-Gonzalez J, Milosevic Z, Mikic M. Correlation between explosive strength, aerobic power and repeated sprint ability in elite basketball players. J Sports Med Phys Fitness. 2012;52(4):375-81. [PubMed ID: 22828459].
-
4.
Castagna C, Manzi V, D'Ottavio S, Annino G, Padua E, Bishop D. Relation between maximal aerobic power and the ability to repeat sprints in young basketball players. J Strength Cond Res. 2007;21(4):1172-6. [PubMed ID: 18076232]. https://doi.org/10.1519/R-20376.1.
-
5.
Caprino D, Clarke ND, Delextrat A. The effect of an official match on repeated sprint ability in junior basketball players. J Sports Sci. 2012;30(11):1165-73. [PubMed ID: 22697579]. https://doi.org/10.1080/02640414.2012.695081.
-
6.
te Wierike SC, de Jong MC, Tromp EJ, Vuijk PJ, Lemmink KA, Malina RM, et al. Development of repeated sprint ability in talented youth basketball players. J Strength Cond Res. 2014;28(4):928-34. [PubMed ID: 24667248]. https://doi.org/10.1097/JSC.0000000000000223.
-
7.
Meckel Y, Gottlieb R, Eliakim A. Repeated sprint tests in young basketball players at different game stages. Eur J Appl Physiol. 2009;107(3):273-9. [PubMed ID: 19572143]. https://doi.org/10.1007/s00421-009-1120-8.
-
8.
Carvalho HM, Coelho ESMJ, Figueiredo AJ, Goncalves CE, Philippaerts RM, Castagna C, et al. Predictors of maximal short-term power outputs in basketball players 14-16 years. Eur J Appl Physiol. 2011;111(5):789-96. [PubMed ID: 20981436]. https://doi.org/10.1007/s00421-010-1703-4.
-
9.
Fiba. Official basketball rules. Barcelona, Spain. FIBA Central Board; 2014.
-
10.
McInnes SE, Carlson JS, Jones CJ, McKenna MJ. The physiological load imposed on basketball players during competition. J Sports Sci. 1995;13(5):387-97. [PubMed ID: 8558625]. https://doi.org/10.1080/02640419508732254.
-
11.
Glaister M, Howatson G, Pattison JR, McInnes G. The reliability and validity of fatigue measures during multiple-sprint work: an issue revisited. J Strength Cond Res. 2008;22(5):1597-601. [PubMed ID: 18714226]. https://doi.org/10.1519/JSC.0b013e318181ab80.
-
12.
Cohen J. Statistical power analysis for the behavioral sciences. Lawrence Erlbaum Associates, Inc; 1977.
-
13.
Batterham AM, Hopkins WG. Making meaningful inferences about magnitudes. Int J Sports Physiol Perform. 2006;1(1):50-7. [PubMed ID: 19114737].
-
14.
Balsalobre-Fernandez C, Tejero-González C, del Campo-Vecino J, Bachero-Mena B, Sánchez-Martínez J. Relationships among repeated sprint ability, vertical jump performance and upper-body strength in professional basketball players. Archivos de Medicina del Deporte. 2014;31(161):148-53.
-
15.
Sampaio J, Abrantes C, Leite N. Power, heart rate and perceived exertion responses to 3x3 and 4x4 basketball small-sided game. Revista de Psicología del Deporte. 2009;18(3):463-7.
-
16.
Nikolaidis PT, Asadi A, Santos EJ, Calleja-Gonzalez J, Padulo J, Chtourou H, et al. Relationship of body mass status with running and jumping performances in young basketball players. Muscles Ligaments Tendons J. 2015;5(3):187-94. [PubMed ID: 26605193]. https://doi.org/10.11138/mltj/2015.5.3.187.
-
17.
Ben Abdelkrim N, Chaouachi A, Chamari K, Chtara M, Castagna C. Positional role and competitive-level differences in elite-level men's basketball players. J Strength Cond Res. 2010;24(5):1346-55. [PubMed ID: 20393355]. https://doi.org/10.1519/JSC.0b013e3181cf7510.
-
18.
Nikolaidis PT, Calleja-Gonzalez J, Padulo J. The effect of age on positional differences in anthropometry, body composition, physique and anaerobic power of elite basketball players. Sport Sciences for Health. 2014;10(3):225-33. https://doi.org/10.1007/s11332-014-0198-5.
-
19.
Drinkwater EJ, Hopkins WG, McKenna MJ, Hunt PH, Pyne DB. Modelling age and secular differences in fitness between basketball players. J Sports Sci. 2007;25(8):869-78. [PubMed ID: 17474040]. https://doi.org/10.1080/02640410600907870.
-
20.
Torres-Unda J, Zarrazquin I, Gil J, Ruiz F, Irazusta A, Kortajarena M, et al. Anthropometric, physiological and maturational characteristics in selected elite and non-elite male adolescent basketball players. J Sports Sci. 2013;31(2):196-203. [PubMed ID: 23046359]. https://doi.org/10.1080/02640414.2012.725133.