Abstract
Background:
Concussion causes acute, short-term brain dysfunctions. However, the impact of repetitive concussion history on brain function remains unclear.Objectives:
The present study examined the effect of a history of multiple concussions on the cognitive functions and dynamic cerebral blood flow (CBF) regulation in collegiate rugby football players.Methods:
Nine male rugby football players with a history of ≤ 1 concussion and nine players with a history of multiple concussions (≥ 4 concussions) participated in this study. Reaction time and working memory were assessed using a neurocognitive assessment device (CogSport; CogState Ltd., Melbourne, Australia). Arterial blood pressure and middle cerebral artery blood velocity (MCAv) were measured continuously throughout the experiment. Dynamic cerebral autoregulation was examined using a thigh-cuff occlusion and release technique.Results:
We found a significant difference in the short-term working memory between players with a history of ≤ 1 concussion and those with a history of multiple concussions (P < 0.05). However, dynamic cerebral autoregulation was not significantly different between the two groups.Conclusions:
We found a dysfunction in the short-term memory function of collegiate rugby football players with a history of multiple concussions. However, this impairment in brain function was not associated with changes in dynamic CBF regulation.Keywords
Cerebral Blood Flow Cognition Head Impact Collision Sports Neuropsychological Test
1. Background
Rugby is a collision sport placing players at a risk of head injury from a blow to the head or from a fall. Indeed, it has been reported that there is a high incidence of concussion in rugby players (4.73 / 1000PH) (1) with high recurrence rates (11.7 %) (2). A concussion causes acute, short-term dysfunction in multiple aspects of cognitive and mental functions as well as the vestibular system (3-5). These short-term brain dysfunctions are likely to disappear within approximately 10 days (6). However, the results of a recent neuroimaging study indicated that the head impact-induced neurochemical cascade associated with concussion can reduce cognitive function, even after the return to play (7-9). Recent studies have also reported that repetitive concussions are associated with long-term effects on brain function, including cognitive dysfunction (10). However, compared with a single concussion, the impact of repetitive concussion history on brain function remains unclear.
Given this background, it is very important to determine the appropriate timing for the return to play in young rugby players who have received a blow to the head and suffered a concussion. Therefore, compared with other injuries, players must follow the World Rugby start to return to play guidelines more carefully if they suffer a concussion (4, 11). It is plausible that multiple concussions may cause more severe brain dysfunction compared with that caused by a single concussion. Nevertheless, the extent to which the history of multiple concussions affects the brain function as well as the mechanisms of such respective concussion-induced brain dysfunctions remains unknown. An understanding of these concussion aspects should aid the development of an effective rehabilitation device or method as well as safer rugby play guidelines.
The potential mechanisms of concussion-induced cognitive dysfunction include physiological factors, such as decreased cerebral blood flow (CBF) (9, 12-14) neurometabolic changes affecting ionic fluxes, and impaired neurotransmission caused by axonal injury (6, 15, 16). In addition, recent studies have investigated the effect of head impact on dynamic cerebral autoregulation, the regulator of CBF (8, 17, 18). Bailey et al. (8) reported that dynamic cerebral autoregulation is impaired in professional boxers diagnosed with chronic traumatic brain injury and suggested that this impairment is related to brain functions, such as memory and attention. Therefore, we hypothesized that young rugby players with a history of multiple head impact and/or concussion injuries have a lower cognitive function compared with those with a history of ≤ 1 concussion. Moreover, multiple concussion-induced long-term brain dysfunction is associated with an impairment in dynamic CBF regulation.
2. Objectives
We aimed to examine (i) cognitive function, including short-term memory and attention and (ii) dynamic cerebral autoregulation in rugby players with multiple and single or no concussion history.
3. Methods
A total of 82 male rugby football players from a university rugby football team (Waseda University) were recruited. All participants were asked to complete a written questionnaire regarding their concussion history. Concussion was based on consensus statement (3, 4), was defined as (i) caused by a direct blow to the body, (ii) result in the impairment of neurological function. Depending on the questionnaire survey, participants with a history of concussion within the last 6 months were excluded. Nine players with a history of > 4 concussions (multiple concussion group: mean age, 20 ± 1 year; mean height, 173 ± 2 cm; mean weight, 80 ± 4 kg) and nine players matching the height and weight with a history of ≤ 1 concussion (control group: mean age, 20 ± 1 year; mean height, 175 ± 5 cm; mean weight, 85 ± 8 kg) were included in the study. Control group included four players with no history of concussion and five players with a history of one concussion. Further investigations included an assessment of neurocognitive function and cerebral autoregulation pre-season. All study protocols were approved by the ethics committee on human research of the Waseda University (No. 2012 - 264). This study conforms to the declaration of Helsinki. All athletes were fully informed of the procedures and purpose of this study and provided written informed consent. The participants were free to withdraw from participation at any time. In addition, all participants were prohibited from strenuous exercise and alcohol intake for at least 24 hour before testing and were asked to refrain from consuming caffeinated beverages on the day of the test.
3.1. Evaluation of Cognitive Function
Neurocognitive function was assessed using a computer-based neurocognitive evaluation system (CogSport; CogState Ltd., Melbourne, Australia) (19-21) that has been evaluated for reliability with an intraclass correlation coefficient of 0.69 - 0.90 (19). Using this system, Collie et al. (20) reported that concussed athletes exhibited a decline in their reaction times compared with the results of the baseline before the start of the football season. The CogSport consists of four tasks that evaluate cognitive function: simple reaction time (psychomotor function), complex reaction time (decision-making), continuous learning (learning), and one-back (working memory) (19). We measured the reaction speed (time, ms) with respect to these four tasks in a soundproof environment.
3.2. Experimental Protocol to Identify Dynamic Cerebral Autoregulation
Dynamic cerebral autoregulation was investigated using the bilateral thigh-cuff occlusion and release technique as described in previous studies (8, 22). Participants were seated in a semi-recumbent position (45°) in a reclining seat and rested quietly for 20 minutes. Following this resting period, they performed three trials of the thigh-cuff occlusion and release protocol to identify dynamic cerebral autoregulation. This protocol was initiated with a baseline period (5 minutes) followed by an inflation of the thigh cuffs (supra-systolic, > 220 mmHg) for 3 minutes, and then the occluded thigh cuffs were released to acutely decrease the arterial blood pressure (ABP). When the thigh cuffs were deflated, we immediately determined the dynamic cerebral autoregulation according to the resultant rapid and transient drop in arterial blood pressure as well as the adaptive changes in middle cerebral artery blood velocity (MCAv) by recording the relevant data for 1 minutes after release of the cuff.
The baseline control values of MAP, MCAv, and CVCi were calculated from the 4 s average immediately before the cuff was released. Changes in these parameters (i.e., MAP, MCAv, and CVCi) incurred during the cuff release relative to their control values were subsequently determined. The rate of regulation (RoR), as an index of dynamic cerebral autoregulation, is the slope of the regression line between CVCi and time (rate of change in CVCi during the 1.0 - 3.5 seconds interval following the cuff release: RoR = (CVCi/time)/MAP).
Throughout the experiment of measuring dynamic cerebral autoregulation, the beat-to-beat mean arterial blood pressure was measured using finger photoplethysmography (Finometer® PRO; finapres medical systems, Amsterdam, the Netherlands), and heart rate was monitored using a lead II electrocardiogram. The middle cerebral artery blood flow velocity (MCAv) was measured using 2-MHz pulsed transcranial doppler ultrasonography (Multidop T; DWL, Sipplingen, Germany). A doppler probe was fitted to the left temporal window with an adjustable headband, and participants were instructed to maintain their head in one position. The beat-by-beat data were sampled at 1 kHz using an analogue-to-digital converter (PowerLab; ADInstruments, Milford, Massachusetts, USA) connected to a personal computer for analysis. Beat-to-beat MAP and MCAv were determined, and the cerebrovascular conductance index (CVCi) was calculated by dividing MCAv by MAP.
3.3. Statistics
All results are expressed as the means ± standard deviations. Differences in cognitive function and cerebral autoregulation between the multiple concussion and control groups were examined using unpaired t-tests conducted using SPSS version 22.0. In addition, cerebral autoregulation and cognitive function were examined using Pearson’s correlation coefficients. Differences were considered significant at P < 0.05. The effect size was interpreted as small effects = 0.20, moderate effects = 0.50, and large effects = 0.80.
4. Results
The participant baseline characteristics are presented in Table 1. There were no significant differences between the two groups regarding age, height, weight, or competition history. The short-term working memory (one-back task) in the multiple concussion group was significantly impaired compared with that of the control group (control group: 526.22 ± 50.16 ms; multiple concussion group: 625.11 ± 89.48 ms; P = 0.01; effect size = 0.59). However, there were no significant differences in the other cognitive tasks. Unexpectedly, there was no significant difference in dynamic cerebral autoregulation between the control and multiple concussion groups (control group: 0.252 ± 0.102; multiple concussion group: 0.259 ± 0.115; P = 0.89). In addition, the individual neurocognitive function was not associated with dynamic cerebral autoregulation (Table 2).
Participants Characteristic
Control Group | Multiple Group | |||||
---|---|---|---|---|---|---|
Mean | SD | Mean | SD | P | Effect Size | |
Age (yaears) | 19.9 | 0.9 | 20.1 | 1.4 | 0.69 | 0.10 |
Height (cm) | 175.3 | 4.7 | 173.1 | 2.3 | 0.25 | 0.30 |
Weight (kg) | 85.3 | 8.3 | 80.4 | 4.4 | 0.15 | 0.36 |
Competition history (years) | 9.0 | 4.5 | 8.0 | 4.0 | 0.63 | 0.12 |
Condition | ||||||
HR (beat/min) | 60.7 | 5.0 | 62.4 | 6.2 | 0.53 | 0.16 |
SBP (mmHg) | 127.6 | 8.1 | 123.9 | 4.5 | 0.24 | 0.29 |
DBP (mmHg) | 69.8 | 6.9 | 65.0 | 6.0 | 0.13 | 0.37 |
MBP (mmHg) | 89.1 | 7.2 | 84.6 | 4.2 | 0.13 | 0.38 |
MCAv (cm/s) | 52.3 | 20.7 | 54.6 | 8.7 | 0.76 | 0.08 |
PET CO2 (mmHg) | 41.2 | 2.9 | 42.8 | 1.8 | 0.18 | 0.33 |
CogSport (ms) | ||||||
SRT | 268.8 | 32.4 | 281.4 | 39.1 | 0.47 | 0.19 |
CRT | 423.6 | 50.3 | 455.4 | 48.7 | 0.19 | 0.32 |
CL | 743.8 | 146.4 | 763.1 | 49.5 | 0.71 | 0.09 |
OB | 526.2 | 50.2 | 625.1a | 89.5 | 0.01 | 0.59 |
Autoregulation | ||||||
RoR | 0.25 | 0.10 | 0.26 | 0.11 | 0.89 | 0.07 |
Slope | 0.05 | 0.03 | 0.06 | 0.03 | 0.48 | 0.18 |
ΔMAP | 0.21 | 0.04 | 0.22 | 0.03 | 0.73 | 0.09 |
Correlation Coefficients Between Cerebral Autoregulation and Neurocognitive Functiona
SRT | CRT | CL | OB | |
---|---|---|---|---|
RoR | -0.08 | 0.00 | 0.19 | 0.16 |
P value | 0.76 | 1.00 | 0.45 | 0.53 |
Representative traces observed during the thigh-cuff release technique. The thigh cuff was released at time 0. Straight lines were determined by a linear regression analysis 1 - 3.5 seconds CVCi = Cerebrovascular conductance index; MAP = Mean arterial blood pressure; MCAv = Middle cerebral artery blood flow velocity; RoR = Rate of regulation
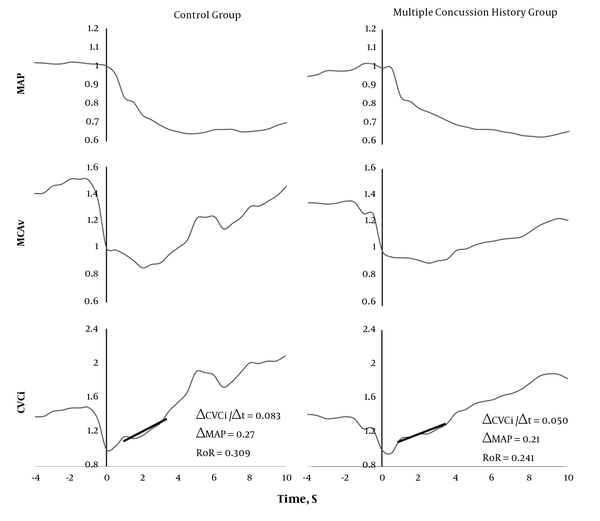
5. Discussion
In the present study, short-term working memory was significantly poorer (P = 0.01) in the players with a history of multiple concussions compared with players with a history of ≤ 1 concussion. However, dynamic cerebral autoregulation did not differ according to the case history of concussions (P = 0.89) and was not associated with an impaired short-term working memory. These findings suggest that concussion history should be considered for the recovery duration, since it may be an important factor in determining brain function following a concussion. In addition, multiple concussion-induced brain dysfunction in rugby football players may be due to an alteration in cerebral neural activity rather than dynamic CBF regulation. This may be important information for an effective rehabilitation program after a concussion.
In the present study, short-term working memory (one-back task) in the multiple concussion group was significantly impaired compared with the players with a history of ≤ 1 concussion. Notably, the reaction time was slower in the multiple concussion group compared with that of the control group (approximately 0.1 second, P = 0.01), and this difference was similar to the values previously reported after a concussion (23, 24). Post-concussion syndrome is characterized by poor short-term memory and headaches (7, 25). In addition, athletes with a history of multiple concussions (23) or a high exposure to head impact are reported to have impaired memory, attention, and processing speed (24). Concussion-related brain dysfunction has been assumed to result from the metabolic and pathophysiological consequences of axonal injury (16). Immediately after a stretching or shearing stress (mechanical stress) is exerted on an axonal fiber, a neuronal depolarization with an alteration in ionic flux occurs, which manifests as a neurological impairment (6, 15, 16). In addition, the abnormal ionic flux enhances glucose metabolism and reduces CBF (16). These metabolic and pathophysiological alterations are likely to have recovered within 10 days after experiencing a concussion (6, 15, 16). However, from the results of the present study, it is possible that a history of multiple concussions may be a limitation in the recovery of these metabolic and pathophysiological alterations to a normal level. Therefore, concussion history should be considered to determine the timing for the return to play for football players in the Rugby safe play guidelines.
Another possible mechanism of concussion-induced brain dysfunction may be related to cerebral circulatory homeostasis. Recent studies have reported that regional blood flow (26) and cerebral perfusion (9) are related to a decrease in working memory in persistent concussion patients. These findings suggest that adequate dynamic CBF regulation may be required to preserve brain function. Indeed, the results of a previous study (8) indicate that an impairment in cognitive function is associated with dynamic cerebral autoregulation in boxers with persistent concussion symptoms. However, contrary to our hypothesis, we observed no significant difference in the dynamic cerebral autoregulation between the multiple concussion and control groups (P = 0.89). Thus, the short-term memory function was not associated with dynamic CBF regulation in rugby players. One explanation for this unexpected result may be the difference in the severity of the concussion or mild traumatic brain injury (mTBI). The professional boxers in the previous study (8) experienced more serious cognitive dysfunction than the participants in the present study. Therefore, it is plausible that the professional boxers may have developed detectable autonomic nervous system problems (27) as a result of the greater head impact exposure compared with that experienced by the rugby player participants in the present study. In addition, the control group of the present study includes rugby players with a history of only a single concussion. Thus, it is possible that our control group also had impairments in dynamic cerebral autoregulation. Indeed, the RoR value (0.255 ± 0.105) is lower than that of the control group (more than 0.3) reported in previous studies (8). Despite these caveats, we found no difference in dynamic cerebral autoregulation but a difference in cognitive function between rugby players with a history of multiple compared to those with a history of ≤ 1 concussion. In contrast to boxers, these findings suggest that repetitive concussions that occur in rugby players may be not associated with dynamic cerebral autoregulation. Moreover, multiple concussion-induced brain dysfunction may be related to metabolic and pathophysiological alterations rather than CBF regulation.
Our study has some considerable limitations. This study was cross-sectional in design, and it is therefore difficult to determine whether there was a causal relationship between the history of multiple concussions and the decreased short-term working memory observed in the multiple concussion group of rugby players. In addition, CogSport has been evaluated for reliability with an intraclass correlation coefficient of 0.69 - 0.90 (19). Although it has moderate reliability, CogSport is one of the appropriate testing methods used for the evaluation of a concussion. Consequently, in a future study, we must consider the mechanism of memory dysfunction caused by repeated concussions and head impacts in young athletes using a longitudinal study design. Finally, in the present study, we could not identify whether the brain function was impaired in players with a single concussion history, because we did not examine any participants without any concussion history. However, our findings clearly suggest that concussion-induced brain dysfunction, particularly that of the working memory, is associated with a case history of repetitive concussions.
The results of this study indicate that collegiate rugby players with a history of multiple concussions have poorer short-term working memory compared with players with ≤ 1 concussion. This finding suggests that brain dysfunction in rugby players may be associated with the concussion case history. However, our findings do not support the hypothesis that the brain dysfunction results from changes in dynamic CBF regulation.
Acknowledgements
References
-
1.
Gardner AJ, Iverson GL, Williams WH, Baker S, Stanwell P. A systematic review and meta-analysis of concussion in rugby union. Sports Med. 2014;44(12):1717-31. [PubMed ID: 25138311]. https://doi.org/10.1007/s40279-014-0233-3.
-
2.
Castile L, Collins CL, McIlvain NM, Comstock RD. The epidemiology of new versus recurrent sports concussions among high school athletes, 2005-2010. Br J Sports Med. 2012;46(8):603-10. [PubMed ID: 22144000]. https://doi.org/10.1136/bjsports-2011-090115.
-
3.
McCrory P, Meeuwisse W, Johnston K, Dvorak J, Aubry M, Molloy M, et al. Consensus Statement on Concussion in Sport: the 3rd International Conference on Concussion in Sport held in Zurich, November 2008. Br J Sports Med. 2009;43 Suppl 1:i76-90. [PubMed ID: 19433429]. https://doi.org/10.1136/bjsm.2009.058248.
-
4.
McCrory P, Meeuwisse WH, Aubry M, Cantu B, Dvorak J, Echemendia RJ, et al. Consensus statement on concussion in sport: the 4th International Conference on Concussion in Sport held in Zurich, November 2012. Br J Sports Med. 2013;47(5):250-8. [PubMed ID: 23479479]. https://doi.org/10.1136/bjsports-2013-092313.
-
5.
McClincy MP, Lovell MR, Pardini J, Collins MW, Spore MK. Recovery from sports concussion in high school and collegiate athletes. Brain Inj. 2006;20(1):33-9. [PubMed ID: 16403698]. https://doi.org/10.1080/02699050500309817.
-
6.
Giza CC, Hovda DA. The Neurometabolic Cascade of Concussion. J Athl Train. 2001;36(3):228-35. [PubMed ID: 12937489].
-
7.
Ahman S, Saveman BI, Styrke J, Bjornstig U, Stalnacke BM. Long-term follow-up of patients with mild traumatic brain injury: a mixed-method study. J Rehabil Med. 2013;45(8):758-64. [PubMed ID: 24002311]. https://doi.org/10.2340/16501977-1182.
-
8.
Bailey DM, Jones DW, Sinnott A, Brugniaux JV, New KJ, Hodson D, et al. Impaired cerebral haemodynamic function associated with chronic traumatic brain injury in professional boxers. Clin Sci (Lond). 2013;124(3):177-89. [PubMed ID: 22913765]. https://doi.org/10.1042/CS20120259.
-
9.
Metting Z, Spikman JM, Rodiger LA, van der Naalt J. Cerebral perfusion and neuropsychological follow up in mild traumatic brain injury: acute versus chronic disturbances? Brain Cogn. 2014;86:24-31. [PubMed ID: 24556319]. https://doi.org/10.1016/j.bandc.2014.01.012.
-
10.
Hart JJ, Kraut MA, Womack KB, Strain J, Didehbani N, Bartz E, et al. Neuroimaging of cognitive dysfunction and depression in aging retired National Football League players: a cross-sectional study. JAMA Neurol. 2013;70(3):326-35. [PubMed ID: 23303193]. https://doi.org/10.1001/2013.jamaneurol.340.
-
11.
Raftery M. Concussion and chronic traumatic encephalopathy: International Rugby Board's response. Br J Sports Med. 2014;48(2):79-80. [PubMed ID: 24096899]. https://doi.org/10.1136/bjsports-2013-093051.
-
12.
Chen JK, Johnston KM, Frey S, Petrides M, Worsley K, Ptito A. Functional abnormalities in symptomatic concussed athletes: an fMRI study. Neuroimage. 2004;22(1):68-82. [PubMed ID: 15109998]. https://doi.org/10.1016/j.neuroimage.2003.12.032.
-
13.
McAllister TW, Sparling MB, Flashman LA, Guerin SJ, Mamourian AC, Saykin AJ. Differential working memory load effects after mild traumatic brain injury. Neuroimage. 2001;14(5):1004-12. [PubMed ID: 11697932]. https://doi.org/10.1006/nimg.2001.0899.
-
14.
Meier TB, Bellgowan PS, Singh R, Kuplicki R, Polanski DW, Mayer AR. Recovery of cerebral blood flow following sports-related concussion. JAMA Neurol. 2015;72(5):530-8. [PubMed ID: 25730545]. https://doi.org/10.1001/jamaneurol.2014.4778.
-
15.
Giza CC, Hovda DA. The new neurometabolic cascade of concussion. Neurosurgery. 2014;75 Suppl 4:S24-33. [PubMed ID: 25232881]. https://doi.org/10.1227/NEU.0000000000000505.
-
16.
MacFarlane MP, Glenn TC. Neurochemical cascade of concussion. Brain Inj. 2015;29(2):139-53. [PubMed ID: 25587743]. https://doi.org/10.3109/02699052.2014.965208.
-
17.
Junger EC, Newell DW, Grant GA, Avellino AM, Ghatan S, Douville CM, et al. Cerebral autoregulation following minor head injury. J Neurosurg. 1997;86(3):425-32. [PubMed ID: 9046298]. https://doi.org/10.3171/jns.1997.86.3.0425.
-
18.
Rangel-Castilla L, Gasco J, Nauta HJ, Okonkwo DO, Robertson CS. Cerebral pressure autoregulation in traumatic brain injury. Neurosurg Focus. 2008;25(4):7. [PubMed ID: 18828705]. https://doi.org/10.3171/FOC.2008.25.10.E7.
-
19.
Collie A, Maruff P, Makdissi M, McCrory P, McStephen M, Darby D. CogSport: reliability and correlation with conventional cognitive tests used in postconcussion medical evaluations. Clin J Sport Med. 2003;13(1):28-32. [PubMed ID: 12544161].
-
20.
Collie A, Makdissi M, Maruff P, Bennell K, McCrory P. Cognition in the days following concussion: comparison of symptomatic versus asymptomatic athletes. J Neurol Neurosurg Psychiatry. 2006;77(2):241-5. [PubMed ID: 16421129]. https://doi.org/10.1136/jnnp.2005.073155.
-
21.
Makdissi M, Darby D, Maruff P, Ugoni A, Brukner P, McCrory PR. Natural history of concussion in sport: markers of severity and implications for management. Am J Sports Med. 2010;38(3):464-71. [PubMed ID: 20194953]. https://doi.org/10.1177/0363546509349491.
-
22.
Ogoh S, Brothers RM, Eubank WL, Raven PB. Autonomic neural control of the cerebral vasculature: acute hypotension. Stroke. 2008;39(7):1979-87. [PubMed ID: 18451346]. https://doi.org/10.1161/STROKEAHA.107.510008.
-
23.
Iverson GL, Gaetz M, Lovell MR, Collins MW. Cumulative effects of concussion in amateur athletes. Brain Inj. 2004;18(5):433-43. [PubMed ID: 15195792]. https://doi.org/10.1080/02699050310001617352.
-
24.
McAllister TW, Flashman LA, Maerlender A, Greenwald RM, Beckwith JG, Tosteson TD, et al. Cognitive effects of one season of head impacts in a cohort of collegiate contact sport athletes. Neurology. 2012;78(22):1777-84. [PubMed ID: 22592370]. https://doi.org/10.1212/WNL.0b013e3182582fe7.
-
25.
Tator CH, Davis H. The postconcussion syndrome in sports and recreation: clinical features and demography in 138 athletes. Neurosurgery. 2014;75 Suppl 4:S106-12. [PubMed ID: 25232874]. https://doi.org/10.1227/NEU.0000000000000484.
-
26.
Dean PJ, Sato JR, Vieira G, McNamara A, Sterr A. Multimodal imaging of mild traumatic brain injury and persistent postconcussion syndrome. Brain Behav. 2015;5(1):45-61. [PubMed ID: 25722949]. https://doi.org/10.1002/brb3.292.
-
27.
Erlanger DM. Exposure to sub-concussive head injury in boxing and other sports. Brain Inj. 2015;29(2):171-4. [PubMed ID: 25313457]. https://doi.org/10.3109/02699052.2014.965211.