Abstract
Background:
The use of deep fried oils (DFOs) to cook foods is associated with numerous diseases. Although the role of physical activity and some supplements on fat lipolysis has been established, the effect of aerobic training (AT) and octopamine (Oct) on octopamine receptor-dependent lipolysis pathway is not well understood.Objectives:
The present study aimed to investigate the effect of AT and Oct supplementation on the expression of Oct receptors in the visceral adipose tissue of DFO-exposed rats.Methods:
In this experimental study, 30 male Wistar rats were divided into (1) healthy control (C), (2) DFO, (3) DFO + Oct, (4) DFO + AT, and (5) DFO + Oct + AT groups. Aerobic training was performed for four weeks, five sessions per week at an intensity of 16 - 26 m/min and equivalent to 50% - 65% VO2max, and Otc supplement was interperitoneally injected at 81 µmol/kg five days a week.Results:
Aerobic training and Oct supplementation increased tyramin-R and hormone-sensitive lipase (HSL) (P ≤ 0.05), while Oct significantly decreased G protein-coupled receptors (GPCR) (P ≤ 0.05). Also, the interaction of AT and Oct on GPCR reduction was significant (P ≤ 0.05).Conclusions:
It seems that AT and Otc alone improve octopamine receptors and lipolysis markers in the visceral adipose tissue of DFO-treated rats, but these two factors have no interactive effects on this pathway.Keywords
Endurance Training Octopamine GPCR TAARs Intra-Abdominal Fat
1. Background
Due to lifestyle changes and business development, the tendency to use fast foods has increased. Fast foods are cooked in oils that are frequently fried at a temperature of 150°C - 200°C (1). Frequent exposure to high temperatures causes changes such as oxidation, hydrolysis, and polymerization in foods cooked in deep fried oils (DFOs), increase oxidative stress in the body and cause high blood pressure, endothelial vascular dysfunction, impaired fat metabolism, obesity, chronic diseases, obesity, atherosclerosis, heart ischemia, and diabetes (2). Some hormones at the cell membrane level and G protein-coupled receptors (GPCRs) with two alpha and beta subunits activate trace-amine associated receptors (TAARs), including β-phenylethylamine, octopamine (Oct), tyramine, and volatile amines (3). Trace amine-associated receptors activate G protein subunits and adenylyl cyclase phosphorylation and increase cyclic adenosine monophosphate (cAMP), which in turn, leads to the phosphorylation of protein kinases A and C and activate element-binding protein (CREB) and nucleus factor of activated T-cells (NFAT) (4, 5).
The prevalence of obesity and the harms of poor diet have led researchers to seek low-risk, non-invasive methods, such as exercise, to prevent or treat their complications (6). Exercise activates adipose triglyceride lipase (ATGL) and hormone-sensitive lipase (HSL) in adipose tissues by activating the stimulating G protein subunit mechanism and cAMP-dependent protein kinase (PKA) phosphorylation (7, 8). However, there are limited studies on the effect of physical activity on the GPCRs-dependent lipolysis pathway. For example, ten weeks of endurance training improved insulin receptor levels, changed the size of fat droplets in the abdominal adipose tissue, and increased HSL, but had no significant effects on changing the nature of fat droplets via the GPCRs-dependent pathway of healthy men (8).
In a review study, it was stated that the activation of the sympathetic system following physical activity activates beta-3 adrenergic receptors and GPCRs and phosphorylates HSL (9). In addition to physical activity, researchers recommend the use of dietary supplements for better results (6). Among them, Oct is an endogenous amine and the active ingredient of some plants, which has lipolysis effects, improves metabolism, and reduces inflammatory factors in adipose tissue by the mechanism of sympathetic nervous system activation and beta-3 adrenergic receptor and HSL phosphorylation (10, 11). In this regard, eight weeks of Oct supplementation at 81 µmol/kg improves the function of M1 and M2 macrophages in adipose tissue (11) and reduces myocyte apoptosis (12) in rats exposed to DFOs. Due to the simultaneous importance of nutrition and physical activity, researchers have shown that Oct supplementation and exercise can interactively reduce apoptosis in the heart tissue (12) and improve the activity of M1 and M2 macrophages in the visceral adipose tissue (11) of rats exposed to DFOs.
2. Objectives
Despite the favorable results regarding the interactive effect of physical activity and Oct supplementation, no study was found to investigate the effect of these two interventions on the GPCRs and TAARs-dependent lipolysis pathway. Therefore, the present study aimed to investigate the effect of eight weeks of AT and Oct supplementation on the expression of Oct receptors in the visceral adipose tissue of rats poisoned with DFOs.
3. Methods
3.1. Subjects
In this experimental trial, 30 male Wistar rats with a mean age of 20 weeks and a weight of 325.00 ± 25.00 gr were prepared from the Histogen Research Center and kept in the laboratory for one week for adaptation. The rats were randomly divided into five groups of six, including (1) healthy control; (2) deep-fried oil (DFO) poisoned control + intraperitoneal injection normal saline (Sham); (3) DFO + octopamine (Oct) supplement; (4) DFO + aerobic training (AT); and (5) DFO + AT + Oct (AT + Oct).
The rats were kept in standard conditions in terms of temperature (22°C to 24°C), 12-hour light and dark cycle, relative humidity 55% to 60%, and free access to water and food in polycarbonate cages with autoclave capability. It is also noteworthy that all the principles of working with laboratory animals in the present study were based on the ethics committee of working with laboratory animals in Histogen.
3.2. Preparation of DFO
In order to prepare fried oil, based on previous studies, 8 L of sunflower oil was used. The oil was fried for 8 h at a temperature of 190°C to 200°C for four consecutive days, and according to sources, chicken nuggets, potatoes, chicken, and protein products (sausages and hotdogs) were immersed in the oil every 30 min. Finally, the oil was stored on the fourth day for use as a poisoning intervention until execution, and 10 mL/kg of the oil was gavaged for four weeks, five days per week (13).
3.3. Octopamine Consumption
Oct supplement was prepared from Sigma Aldrich Company. Oct-receiving groups received 81 µmol/kg by intraperitoneal injection for four weeks and five days per week. To prepare Oct for injection, it was dissolved in 9% normal saline (13).
3.4. Aerobic Training Protocol
The training protocol was performed for four weeks at a moderate intensity. According to studies, the training intensity reached 50% of VO2max in the first week and reached 65% of VO2max in the last week. In order to adapt, the rats performed one week of adaptation training at a speed of 9 m/min and duration of 20 min before the start of the main training program.
According to previous studies, the training duration was 20 min, and the intensity of training was 16 m/min on the first day and reached 26 m/min on the last day. The rats cooled for 5 min at a speed of 7 m/min and after the main training for 5 min at a speed of 5 m/min (11).
3.5. Dissection and Sampling of Visceral Adipose Tissue
The rats were anesthetized with a combination of ketamine (70 mg/kg) and xylazine (3 - 5 mg/kg) 48 hours after the last training session and following 12 hours of fasting. After complete anesthesia, the abdominal cavity was dissected, and visceral fat was completely extracted; then, 3 g of visceral adipose tissue was placed in special tissue storage microtubes for sampling. The samples were immediately immersed in liquid nitrogen and then stored at -80°C for use during measurement.
3.6. GPCR Assay by Western Blot
To measure GPCR levels by the western blot method, equal amounts of protein were boiled for 5 to 10 min and then run on polyacrylamide for 2 h to 3 h at 100 V. Following this step, the electrophoresis gel was prepared for transfer. Then, nitrocellulose paper with device pads and sponges were sandwiched in the transfer buffer and electrophoresed at 350 amps for 1 h. The nitrocellulose paper was then washed using Tris-buffered saline (TBS) buffer for 5 to 10 min. Next, the nitrocellulose paper was again placed at room temperature for one hour, immersed in TBS (or blocking) buffer, and gently shaken, and the nitrocellulose paper was washed again in TBS buffer several times.
In the next step, nitrocellulose paper was diluted with secondary antibody (dilution of 1/3000 antibody) at room temperature for 1 h to 2 h and incubated in TBS buffer. The nitrocellulose paper was then washed again with TBS buffer. In the next step, nitrocellulose paper appeared on the photographic film in the dark room by the exposing solution and stabilizer using ECL, and after the bands appeared, the nitrocellulose paper was washed with distilled water. The paper with the β-Actin antibody was placed on the paper and re-incubated with the secondary antibody; the control β-Actin also appeared on X-ray film, and the obtained bands were analyzed by Image J.
3.7. Assessment of HSL by Immunohistochemistry
To measure HSL, 20 g of para formaldehyde (Germany, Merck) was added to 500 cc of distilled water and placed on a hot plate to reach a temperature of 60°C. NaOH was then added to the solution to reach the pH of the solution to 7.2 to 7.4. Finally, a PBS tablet was placed in the solution. Then, to prepare 2 N hydrochloric acid, 2 cc of 37.5% hydrochloric acid was added to 11 cc of distilled water. Also, to prepare 10% goat serum and 0.3% Triton, 100 Landa goat serum was added to 900 Landa PBS, and 10% goat serum was obtained. Also, 3 Alenda Triton 100x was added to 997 Alenda PBS, and 0.3% Triton was obtained. The samples were then washed with PBS in four steps at 5-minute intervals. To recover the antigen, 2 N hydrochloric acid was poured on the samples for 30 minutes. Borate buffer was added to neutralize the acid for 5 minutes. Cells were washed with PBS, and then 0.3% Triton was used for 30 minutes to make cell membranes permeable. They were washed with PBS, and 10% goat serum was added as an additional background dye for 30 min to block the secondary antibody reaction. The primary diluted antibody (1 in 100) with PBS was added to the sample, and after creating a humid environment to prevent tissue drying, it was placed in the refrigerator overnight at 2°C to 8°C. On the next day, the tissue container was removed from the refrigerator and then washed four times with PBS, each time for 5 min. Secondary antibodies were added to the sample at a dilution of 1 to 150 and then incubated in the dark at 37°C for 1 hour and 30 minutes in the incubator. The samples were then transferred from the incubator to the dark room, and after four washes, DAPI was added to them. Then, immediately after removal, PBS was poured on the samples. In the last step, the samples were observed by an Olympus fluorescent microscope with a 400X lens to confirm the markers (Figure 1).
Visceral adipose tissue sample by Olympus Model fluorescent microscopy and 400X lens
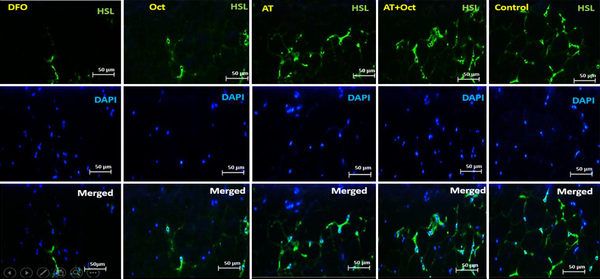
3.8. Assessment of TAARs and Tyramine-R Gene Expression Levels
For molecular studies at the level of gene expression, 50 to 100 mg of visceral adipose tissue was placed in a homogenizer or a small mortar, and the samples were completely crushed. In the following, RNA was first extracted from the adipose tissue according to the manufacturer’s protocol (TRIzol®, manufactured by Qiagen Co., USA with catalog number 79306). Then, using the light absorption property at 260 nm and the following formula, the concentration and purity of the RNA sample was quantitatively obtained.
After extracting RNA with very high purity and concentration from all the studied samples, cDNA synthesis steps were performed according to the protocol of RevertAid First Strand cDNA Synthesis made by Thermo Scientific Co. USA, with catalog number K1622. Then, the synthesized cDNA was used to perform the reverse transcription reaction. First, the designed primers related to genes were examined, and then the expression of the genes was examined using quantitative q-RT PCR. The sequence of primers used in the study is presented in Table 1.
Primer Sequence of the Genes Evaluated in the Research
Genes | Primer Sequences |
---|---|
GAPDH | |
Forward | 5’-AAGTTCAACGGCACAGTCAAGG-3’ |
Reverse | 5’-CATACTCAGCACCAGCATCACC-3’ |
Tyramin-R | |
Forward | 5’-TGCCAAGAGGACAGGGGTTA-3’ |
Reverse | 5’-GATAAGGACCACGACGGCAT-3’ |
TAAR1 | |
Forward | 5’-TCCATTCTCCACCTAGCCTTC-3’ |
Reverse | 5’-TACTGCTCCTCAACTCCTTCT-3’ |
It is also noteworthy that the gene expression levels of the research variables were assessed using the GAPDH reference gene. Then, the following formula was applied for quantification based on determining the expression ratio of the desired gene to the reference gene, which is based on the difference yield in Ct.
3.9. Statistical Model
The Shapiro-Wilk test was used to investigate the normal distribution of the findings. The results of independent samples t-test were used to investigate the effect of DFO on the levels of research variables. Also, two-way analysis of variance (ANOVA) was used to evaluate the main effect of training, the main effect of Oct supplementation, and the interactive effect of training and Oct supplementation. Besides, Bonferroni’s post hoc test was run to evaluate the impact of each intervention. The findings of the present study were analyzed in SPSS, version 22, and the significance level was set at 0.05.
4. Results
The results of independent samples t-test showed that GPCR protein levels in the control group were significantly higher than those in the DFO group (P = 0.004; Figure 2), but the gene expression levels of tyramin-R (P = 0.012; Figure 3) and TAAR1 (P = 0.001; Figure 4) and HSL concentration (P = 0.001; Figure 5) in the DFO group were significantly lower than those in the control group.
Results of two-way analysis of variance (ANOVA) in GPCR Protein levels. **, (P ≥ 0.01) indicates an increase in the deep fried oil group compared to the control group; #, (P ≥ 0.05); and ##, (P ≥ 0.01) indicate a decrease in the AT + Oct and Oct groups compared to the deep fried oil group.
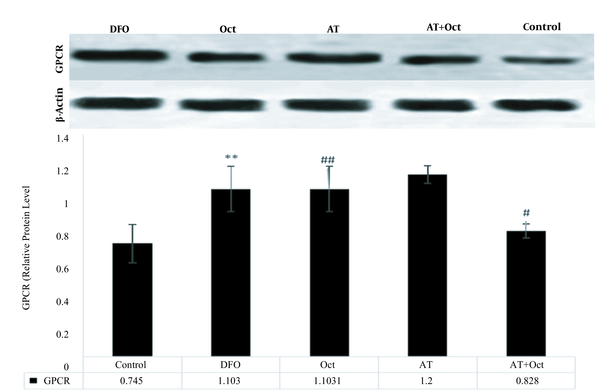
Results of two-way analysis of variance on tyramin-R gene expression levels. *, (P ≥ 0.05) indicates a decrease in the deep fried oil group compared to the control group; ##, (P ≥ 0.01); ###, (P ≥ 0.001), indicates an increase in Oct and AT groups compared to the deep fried oil group.
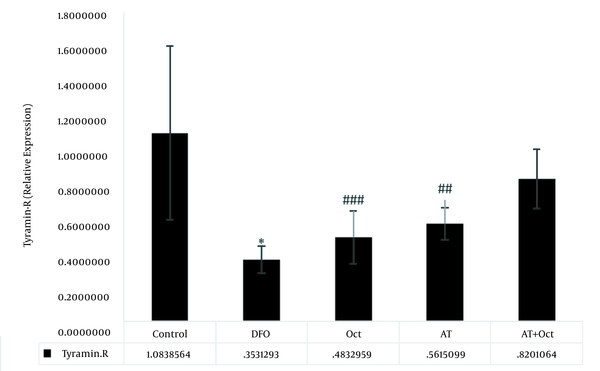
Results of two-way analysis of variance on TAAR1 gene expression levels. ***, (P ≥ 0.001) indicates a decrease in the deep fried oil group compared to the control group; ##, (P ≥ 0.01) indicates an increase in the Oct and AT groups compared to the deep fried oil group.
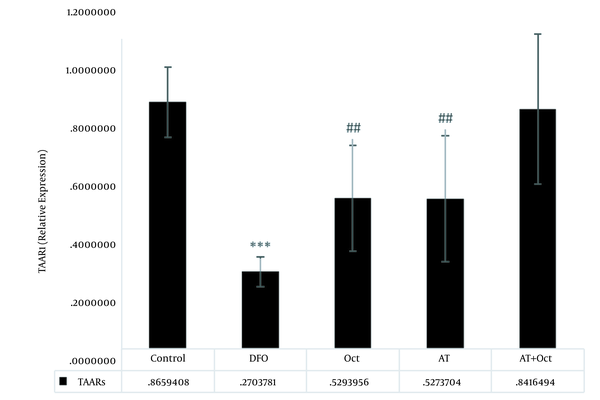
Results of two-way analysis of variance for concentration percentage of HSL protein expression. ***, (P ≥ 0.001) indicates a decrease in the deep fried oil group compared to the control group; ###, (P ≥ 0.001) indicates an increase in the Oct and AT groups compared to the deep fried oil group.
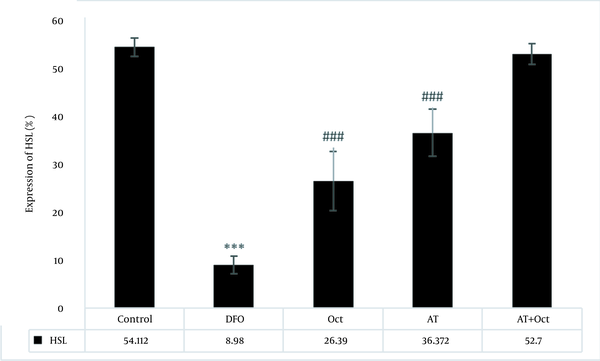
The results of two-way ANOVA showed that training did not have a significant effect on increasing GPCR levels (P = 0.89), while octopamine decreased GPCR protein levels (P = 0.001). Also, the interaction of training and octopamine supplementation on the reduction of GPCR in DFO-exposed rats was significant (P = 0.04; Figure 2). Training (P = 0.001) and octopamine (P = 0.004) increased tyramin-R gene expression level, but the interaction of training and octopamine supplementation was not significant to increase tyramin-R gene expression in the adipose tissue of DFO-exposed rats (P = 0.28; Figure 3).
The effects of training (P = 0.005) and octopamine supplementation (P = 0.005) on increasing TAAR1 gene expression were not significant, also the interaction of training and octopamine supplementation was not significant to increase TAAR1 gene expression in the adipose tissue of DFO-exposed rats (P = 0.75; Figure 4).
The effects of training (P = 0.001) and octopamine supplementation (P = 0.001) on increasing HSL were significant, while the interaction of training and octopamine supplementation was not significant to increase HSL (P = 0.77; Figure 5).
The evaluation of HSL protein concentration in visceral adipose tissue in the research groups. In this figure, the green light indicates the concentration of HSL. As can be seen, the highest concentration is observed in the control group and the lowest in the deep fried oil group. HSL concentration also increases in the AT + Oct and AT groups. In addition, although the HSL concentration is higher in the Oct group than the DFO group, the increase in HSL concentration is more dependent on training.
5. Discussion
The results of the present study showed that aerobic training amplified the gene expression level of tyramin-R and the protein level of HSL in the visceral adipose tissue of DFO-poisoned rats. It seems that the sympathetic nervous system and the increase in catecholamines following physical activity are the main factors of lipolysis of white adipose tissue and thermogenesis by the mechanism of binding to β-adrenergic receptors. Increased adrenaline activates the β-3-adrenergic-receptor and binds them to the receptor activate Gs, which leads to an increase in cAMP as a secondary messenger. Metabolic proteins are then activated by PKA activation; also, physical activity by increasing the expression of atrial natriuretic peptide (ANP) receptor A leads to an increase in guanine cyclase, cGMP, and protein kinase G, which directly leads to the phosphorylation of HSL or can participate in facilitating the function of HSL on fat droplets by deforming perilipin (14). In addition, TAAR1 is a receptor bound to the Gαs protein and is sensitive to the receptors of tyramine, octopamine, most neuropeptides, and ANPs and activates the release and modification of calcium pathway and HSL phosphorylation in adipose tissue during exercise through the cGMP mechanism (15, 16).
Regarding the effect of physical activity on adipose tissue lipolysis, it is shown that eight weeks of endurance training for 40 minutes a day at an intensity of 70% to 90% of VO2max with an ergometer bike improved insulin resistance in adipose tissue and increased insulin receptor, hexokinase II, in obese adipose tissue of young men. However, it had no significant effect on changes in fat droplets and GPCRs in adipose tissue (8). In another study, researchers showed that eight weeks, five sessions per week and each session 60 minutes, of exercise at 60% of maximum speed reduced p-AKT/t-AKT ratio but increased ATGL and uncoupling protein 1 (UCP-1) in the adipose tissue of rats poisoned with cafeteria diet (17); also, eight weeks of endurance training induced intramuscular triglyceride and increased oxidation capacity, ATGL, perilipin 3 and 5, and HSL, while having no significant effect on G protein levels in obese subjects (18). Research has shown that the study of lipolysis-related pathways associated with GPCRs and TAARs is a new topic, but there is a scarcity of studies addressing this mechanism and the effect of exercise on it. However, the intensity of training, duration of the training, type of training, and the method of measuring variables are factors affecting the research results.
The results showed that Oct consumption decreased GPCR protein level and increased tyramine-R and HSL expression in the adipose tissue of DFO-poisoned rats. The adrenergic receptor most sensitive to Oct is the type 3 beta receptor, which has an inhibitory effect on beta 2 and alpha 1 receptors and increases lipolysis by activating cAMP. Oct has a similar function to neurotransmitters such as epinephrine, norepinephrine, and tyramine, which help the body in muscle contraction and carboxylate metabolism. GPCRs, also known as TAARs, have significant impacts on mammalian biogenetics; p-octopamine, tyramine, tryptamine, and b-phenylethylamine by binding to the active G protein, cAMP, which leads to HSL and perilipin phosphorylation and increased lipolysis (10, 19).
While the lipolysis effects of Oct are dose-dependent, regarding the effect of Oct on lipolysis pathways and fat mass reduction, a review study showed that although p-Octopamine has less tendency to bind to α-1, α-2, β-1, and β-2 adrenergic, it strongly tends to bind to β-3, and with its dependent pathways, it can activate lipolysis in adipose tissue (20). Also, 1 mmol of Oct increased β-3 adrenergic expression and improved glucose transporter and monoamine oxidase oxidation in rats (21). In another study, researchers exhibited that Oct increased endogenous levels of epinephrine and norepinephrine and induced lipolysis (20). In one study, Oct and tyramine consumption improved fat metabolism (22). Therefore, it seems that dose-dependent octopamine can improve lipolysis in rats exposed to DFO. Therefore, the lack of effect of Oct on TAAR1 can be attributed to the dose.
The results of the present study revealed that AT and Oct consumption had an interactive effect in reducing GPCR and increasing the expressions of tyramin-R, TAAR1, and HSL in adipose tissue of DFO-poisoned rats. Exercise increases HSL activity by increasing catecholamines, phosphorylation of lipolysis proteins, and promoting active G and cAMP. Physical activity also leads to HSL phosphorylation by increasing the expression of ANP, guanine cyclase, cGMP, and protein kinase G (14). Atrial natriuretic peptide also activates cGMP, releases and modulates calcium, and increases HSL in adipose tissue (15, 16). Oct supplementation also enhances cAMP by rising the expression of β-3 adrenergic. In addition, Oct, like catecholamines and neurotransmitters, helps the body by binding to GPCRs, phosphorylation of HSL, increasing lipolysis, and biological activity (10, 19).
According to former studies, these two interventions seem to have similar pathways, but cannot activate this pathway interactively. In this regard, the simultaneous use of Oct and exercise at an intensity of 55% of maximum power caused oxidation and improved performance of athletes, but it had no significant impact on increasing lipolysis hormones in men (23). Also, aerobic training and Oct supplementation had no interactive effects on reducing inflammatory factors in the heart tissue of rats exposed to DFO (24).
According to the results of previous studies, it seems that the duration of physical activity, dose of Oct, and basic metabolic disorders are the factors affecting the results of aerobic training and Oct.
Due to the role of beta-adrenergic pathways in both interventions, lack of measurement of beta-adrenergic receptors is one of the limitations of the present study. Therefore, future studies are suggested to evaluate these mechanisms. Also, considering the effect of both interventions on some lipolysis markers, it seems that the study of perilipin levels and microscopic image of fat cells in future studies is necessary.
5.1. Conclusions
Aerobic training and Oct supplementation appear to improve Oct receptors and lipolysis markers in the visceral adipose tissue of DFO-treated rats, but these two factors do not have an interactive effect on this pathway.
References
-
1.
Rayhan MA, Islam MK, Khatun MA, Islam D, Rahman MN. Remedial role of exercise training to deep‐fried oil‐induced metabolic and histological changes in Wistar rats. J Food Biochem. 2020;44(11). https://doi.org/10.1111/jfbc.13458.
-
2.
Yuan L, Jiang F, Cao X, Liu Y, Xu YJ. Metabolomics reveals the toxicological effects of polar compounds from frying palm oil. Food Funct. 2020;11(2):1611-23. [PubMed ID: 32020140]. https://doi.org/10.1039/c9fo02728a.
-
3.
Zucchi R, Chiellini G, Scanlan TS, Grandy DK. Trace amine-associated receptors and their ligands. Br J Pharmacol. 2006;149(8):967-78. [PubMed ID: 17088868]. [PubMed Central ID: PMC2014643]. https://doi.org/10.1038/sj.bjp.0706948.
-
4.
Rutigliano G, Bandini L, Sestito S, Chiellini G. 3-Iodothyronamine and Derivatives: New Allies Against Metabolic Syndrome? Int J Mol Sci. 2020;21(6). [PubMed ID: 32183490]. [PubMed Central ID: PMC7139928]. https://doi.org/10.3390/ijms21062005.
-
5.
Nielsen TS, Jessen N, Jorgensen JO, Moller N, Lund S. Dissecting adipose tissue lipolysis: molecular regulation and implications for metabolic disease. J Mol Endocrinol. 2014;52(3):R199-222. [PubMed ID: 24577718]. https://doi.org/10.1530/JME-13-0277.
-
6.
Davari F, Alimanesh Z, Alimanesh Z, Salehi O, Hosseini SA. Effect of training and crocin supplementation on mitochondrial biogenesis and redox-sensitive transcription factors in liver tissue of type 2 diabetic rats. Arch Physiol Biochem. 2020:1-6. [PubMed ID: 32401063]. https://doi.org/10.1080/13813455.2020.1762663.
-
7.
Kato H, Ogasawara J, Takakura H, Shirato K, Sakurai T, Kizaki T, et al. Exercise Training-Enhanced Lipolytic Potency to Catecholamine Depends on the Time of the Day. Int J Mol Sci. 2020;21(18). [PubMed ID: 32967199]. [PubMed Central ID: PMC7554872]. https://doi.org/10.3390/ijms21186920.
-
8.
Riis S, Christensen B, Nellemann B, Moller AB, Husted AS, Pedersen SB, et al. Molecular adaptations in human subcutaneous adipose tissue after ten weeks of endurance exercise training in healthy males. J Appl Physiol (1985). 2019;126(3):569-77. [PubMed ID: 30571288]. https://doi.org/10.1152/japplphysiol.00989.2018.
-
9.
Ferreira R, Nogueira-Ferreira R, Vitorino R, Santos LL, Moreira-Goncalves D. The impact of exercise training on adipose tissue remodelling in cancer cachexia. Porto Biomed J. 2017;2(6):333-9. [PubMed ID: 32258790]. [PubMed Central ID: PMC6806758]. https://doi.org/10.1016/j.pbj.2017.02.006.
-
10.
Carpéné M, Testar X, Carpéné C. High doses of synephrine and octopamine activate lipolysis in human adipocytes, indicating that amines from Citrus might influence adiposity. Mol Phylogeny Antioxid Prop Med Uses. 2014:141-68.
-
11.
Mahmudi R, Azarbayjani MA, Peeri M, Farzanegi P. Effects of Training and Octopamine Supplementation on Expression of M1 and M2 Monocyte/Macrophage Surface Markers in White Adipose Tissue of Rats Poisoned with Deep-Fried Oil. Gene Cell Tissue. 2020;7(1). https://doi.org/10.5812/gct.100036.
-
12.
Kianmehr P, Azarbayjani MA, Peeri M, Farzanegi P. Synergic effects of exercise training and octopamine on peroxisome proliferator-activated receptor-gamma coactivator -1a and uncoupling protein 1 mRNA in heart tissue of rat treated with deep frying oil. Biochem Biophys Rep. 2020;22:100735. [PubMed ID: 32140572]. [PubMed Central ID: PMC7047140]. https://doi.org/10.1016/j.bbrep.2020.100735.
-
13.
Shokri F, Azarbayjani MA, Peeri M, Ghazalian F. The Effect of Octopamine and Aerobic Exerciseand on Genes Affecting Angiogenesis of Visceral Adipose Tissue in Rats Fed with Deep-Fried Oil. J Fasting Health. 2020;8(3):192-8.
-
14.
Rodrigues AC, Prímola-Gomes TN, Peluzio MCG, Hermsdorff HHM, Natali AJ. Aerobic exercise and lipolysis: A review of the β-adrenergic signaling pathways in adipose tissue. Sci Sports. 2020.
-
15.
Sengenes C, Zakaroff-Girard A, Moulin A, Berlan M, Bouloumie A, Lafontan M, et al. Natriuretic peptide-dependent lipolysis in fat cells is a primate specificity. Am J Physiol Regul Integr Comp Physiol. 2002;283(1):R257-65. [PubMed ID: 12069952]. https://doi.org/10.1152/ajpregu.00453.2001.
-
16.
Decker AM, Mathews KM, Blough BE, Gilmour BP. Validation of a High-Throughput Calcium Mobilization Assay for the Human Trace Amine-Associated Receptor 1. SLAS Discov. 2021;26(1):140-50. [PubMed ID: 32734809]. https://doi.org/10.1177/2472555220945279.
-
17.
Americo ALV, Muller CR, Vecchiatto B, Martucci LF, Fonseca-Alaniz MH, Evangelista FS. Aerobic exercise training prevents obesity and insulin resistance independent of the renin angiotensin system modulation in the subcutaneous white adipose tissue. PLoS One. 2019;14(4). e0215896. [PubMed ID: 31022246]. [PubMed Central ID: PMC6483229]. https://doi.org/10.1371/journal.pone.0215896.
-
18.
Louche K, Badin PM, Montastier E, Laurens C, Bourlier V, de Glisezinski I, et al. Endurance exercise training up-regulates lipolytic proteins and reduces triglyceride content in skeletal muscle of obese subjects. J Clin Endocrinol Metab. 2013;98(12):4863-71. [PubMed ID: 24178794]. https://doi.org/10.1210/jc.2013-2058.
-
19.
Stohs SJ. Physiological functions and pharmacological and toxicological effects of p-octopamine. Drug Chem Toxicol. 2015;38(1):106-12. [PubMed ID: 24654910]. https://doi.org/10.3109/01480545.2014.900069.
-
20.
Stohs SJ, Shara M, Ray SD. p-Synephrine, ephedrine, p-octopamine and m-synephrine: Comparative mechanistic, physiological and pharmacological properties. Phytother Res. 2020;34(8):1838-46. [PubMed ID: 32101364]. [PubMed Central ID: PMC7496387]. https://doi.org/10.1002/ptr.6649.
-
21.
Visentin V, Morin N, Fontana E, Prevot D, Boucher J, Castan I, et al. Dual action of octopamine on glucose transport into adipocytes: inhibition via beta3-adrenoceptor activation and stimulation via oxidation by amine oxidases. J Pharmacol Exp Ther. 2001;299(1):96-104. [PubMed ID: 11561068].
-
22.
Roeder T. The control of metabolic traits by octopamine and tyramine in invertebrates. J Exp Biol. 2020;223(Pt 7). [PubMed ID: 32238440]. https://doi.org/10.1242/jeb.194282.
-
23.
Beaumont RE, Cordery P, James LJ, Watson P. Supplementation with a low-dose of octopamine does not influence endurance cycling performance in recreationally active men. J Sci Med Sport. 2017;20(10):952-6. [PubMed ID: 28389217]. https://doi.org/10.1016/j.jsams.2017.03.007.
-
24.
Jafari Amoli F, Hosseini SA, Azarbayjani MA, Farzanegi Arkhazloo P. The Anti-inflammatory Effects of Aerobic Training and Octopamine Consumption in the Heart Tissue of Rats Fed Deep-Fried Oil. Jorjani Biomed J. 2019;7(4):11-9. https://doi.org/10.29252/jorjanibiomedj.7.4.11.