Abstract
Background:
Cholestasis is a pathophysiological condition, significantly reducing spermatozoa production. MiR-34c is highly expressed in adult male testicles and controls different stages of spermatogenesis.Objectives:
Here, we aimed to investigate miR-34c expression in the testes of rat models of cholestasis. The expressions of THY-1, FGF-2, and CASP-3 genes, that are targeted by mirR-34c were also investigated.Methods:
Cholestasis was induced in six adult rats via bile duct ligation. Four weeks after cholestasis induction, sera and testicular tissues were collected for further examinations. The levels of liver enzymes were measured using the ELISA. The structure of the testes was evaluated by histological examination. Total RNA was extracted from testes using a special kit and converted to cDNA. The expressions of miR-34c-5p, THY-1, FGF-2, and CASP-3 genes were determined by Real-Time PCR.Results:
The serum levels of ALP, AST, and ALT were significantly elevated in the rat models of cholestasis (P < 0.001). Real-Time PCR revealed that the expressions of miR-34c-5p, THY-1, and FGF-2 genes decreased while CASP-3 gene was upregulated in the testes of cholestatic animals (all differences were significant at P < 0.05).Conclusions:
Our study indicated that cholestasis was associated with reduced expression of miR-34c and altered expression of its target genes in the testis. Our results highlight the potential effects of cholestasis, a hepatobiliary disease, on testicular tissue function and male fertility.Keywords
1. Background
Bile acid (BA) secretion is a critical biological process regulating cholesterol and lecithin excretion in the human body, and its disruption can result in cholestasis (1). Cholestasis affects the function of different organs due to the aggregation of bile in the body, including the male reproductive system (2). Previous studies have shown significant changes in reproductive organs’ weights, suppression of testosterone production, reduction of sperm count and motility, histopathological changes in testes, and increased testicular oxidative stress biomarkers in the testes of the rat models of cholestasis (3). The inflammatory reactions induced by cholestasis and the subsequent macrophages’ entry increase autophagy in spermatocytes and disrupt spermatogenesis (1).
Micro RNAs (miRNAs) account for 1-5% of the human genome and regulate at least 30% of protein-encoding genes (4). MiRNAs have important roles in regulating liver function and modulating its pathogenic conditions; for example, miR-let7a-5p affects the expression of adenosine triphosphate–binding cassette subfamily C member 2 (ABCC2/Abcc2) gene that is up-regulated in obstructive cholestasis (5). In humans, hepatic multidrug resistance protein 3 (MDR3), which is down-regulated in the cholestatic liver, is directly regulated by miR-378a-5p (6). Several lines of evidence have suggested significant roles for miRNAs in spermatogenesis and male fertility (7). They also regulate cellular responses to the hormones secreted by Sertoli cells (8). Because of their important regulatory roles, they can be considered as biomarkers for screening and identifying male infertility (9). MiR-34c is one of the significant miRNAs contributing to mammalian spermatogenesis (10, 11) and is highly expressed in adult male testicles where it controls the proliferation and differentiation of spermatogonia cells (12) and is involved in the four stages of spermatogenesis (13). Altered expression of miR-34c has been shown in various infertility disorders such as oligoasthenozoospermia, asthenozoospermia, and azoospermia (10, 14). Investigating the expression profile of miRNAs in azoospermic patients suggested miR34c to be essential for spermatocyte meiosis (15) and the development and formation of spermatocytes and sperms in adult primates (16). It was shown that increased miR-34c expression in mature mouse testes contributed to sperm production and the regulation of mouse embryonic stem cells’ differentiation to male germ cells (11). In one study, the over-expression of miR-34c led to the up-regulation of the genes associated with meiosis, including Nanos3, Scp3, and Stra8, during mouse spermatogenesis (17). The computational predictions illustrated on http://mirgate.bioinfo.cnio.es/miRGate/ revealed that mir-34c-5p targets THY-1, Fibroblast Growth Factor 2 (FGF-2), and Caspase-3 (CASP-3) genes (Table 1). Fibroblast Growth Factor 2 is secreted from various cells in the testis, and it has been revealed that FGF-2-deficient mice were unable to produce normal spermatozoa (18). Besides, THY-1 or CD90 is a spermatogonia stem cell factor that in rodents indicates the presence of SSCs in testes (19). Finally, CASP-3 encodes a protease involved in cell death, and its activation under cholestasis-induced oxidative stress promotes cell apoptosis in the testis (20).
The Computational Predictions of miR-34c-5p on Thy-1, Casp-3, and Fgf2 Genes
Input Genes | Transcripts | miRNA | Start | Stop | Computational Predictions |
---|---|---|---|---|---|
Thy-1 | ENSRNOT00000008685 | rno-mir-34c-5p | 662 | 684 | 3 |
Casp-3 | ENSRNOT00000014095 | rno-mir-34c-5p | 159 | 184 | 1 |
Fgf-2 | ENSRNOT00000023388 | rno-mir-34c-5p | 10 | 18 | 2 |
2. Objectives
Regarding the importance of mir-34c for proper spermatogenesis and the relationship between cholestasis and testicular dysfunction, in the current study, we aimed to study the expression of miR-34c-5p and its target genes (i.e., THY-1, FGF-2, and CASP-3) in an animal model of cholestasis.
3. Methods
3.1. Cholestasis Induction in Animals and Tissue Collection
In this study, male Rattus norvegicus Wistar rats of 2 - 2.5-month-old, weighing between 200 and 300 g were transferred to our laboratory and kept in a controlled environment. Rats were divided into two groups; the control and cholestasis model (n = 6 per group). In order to create cholestasis models, rats were anesthetized by the intraperitoneal injection of ketamine and xylazine. Then by making an incision in the abdomen’s midline, the common bile duct was exposed, ligated close to the liver hilum just beneath the bifurcation, and then dissected (21). The rats were placed in cages and housed for four weeks in controlled conditions. After four weeks, cholestasis rats were sacrificed by CO2 inhalation, and their testicles were collected for further analyses.
3.2. Histological Assay
Paraffin-embedded specimens of testes were prepared following ethanol dehydration and xylene clearance. The tissues were sectioned at the thickness of 7 µm, and the sections were deparaffinized in xylene and rehydrated by ethanol. Then tissue slides were embedded into hematoxylin for two minutes. For eosin staining, the sections were dehydrated using ethanol and then kept in eosin for two minutes. Finally, the sections were cleared using xyleneand the slides were examined under a light microscope
3.3. Evaluation of ALP, AST, and ALT Liver Enzymes
In order to confirm the creation of cholestasis, serum levels of alkaline phosphatase (ALP), aspartate aminotransferase (AST), and alanine aminotransferase (ALT) enzymes were assessed in the experimental rat models. Briefly, four weeks after cholestasis induction, blood was collected from the heart, and after 30 minutes of staying at room temperature and blood clot formation, the serum was separated and assayed for the mentioned enzymes using special kits (Pars Azmoon, Iran).
3.4. Real-Time PCR
The gene expressions of THY-1, FGF-2, CASP-3, and miR-34c-5p were analyzed in the testicular tissues of the control and cholestasis groups using Real-Time PCR. In summary, RNA was extracted from testicular tissues according to the manufacturer’s protocols (QIAGEN, Germany). The purity and concentration of RNA were assessed using the Agilent 2100 bio-analyzer (Agilent Technologies, USA). The synthesis of cDNA was carried out by the Revert Aid First-Strand cDNA Synthesis Kit (Fermentas, USA) according to the manufacturer's recommendations. Real-Time PCR was conducted using the ABI 7500 (7500 Real-Time PCR Software) instrument and SYBR Green Master Mix (QIAGEN, Germany) in a final volume of 20 µL. The thermal cycling was performed based on the following program; initial denaturation at 95°C for 15 minutes, followed by 40 cycles of annealing at 58°C for 10 seconds and amplification at 72°C for 15 seconds with a final extension at 72°C for 10 min. The Gapdh gene was utilized as an internal control, and the fold-change of the genes was calculated by the 2-ΔΔCT method. Primers have been listed in Table 2.
Sequences of the Primers Designed for This Study
Genes | Forward (5′-3′) | Reverse (5′-3′) |
---|---|---|
Gapdh | AAGTTCAACGGCACAGTCAAGG | CATACTCAGCACCAGCATCACC |
Fgf-2 | AGAAGAGAGAGGAGTTGTG | GGTAAGTGTTGTAGTTATTGGA |
Casp-3 | AAGTGATGGAGATGAAGGAGT | CAGGCGTGAATGATGAAGAGT |
Thy-1 | GATATGGATGAGGGAAGTTGG | ATAGAAGGGGGCTGAGAATGA |
U6 | TGCTTCGGCAGCACATATAC | AGGGGCCATGCTAATCTTCT |
miR-34c-5p | AGGCAGTGTAGTTAGC | GTGCAGGGTCCGAGGT |
3.5. Statistical Analysis
All experiments were conducted at least three times. The difference between the groups was evaluated by one-way ANOVA and Tukey post hoc test. The level of statistical significance was considered at a p-value of less than 0.05.
3.6. Ethical Statement
All experimental procedures in this study were approved by the ethical committee of Kharazmi University under the code of 20288.
4. Results
4.1. Tissue Structure of Testes in Cholestasis Rats
A comparison of seminiferous tubular structure between healthy and cholestasis mice showed that cholestasis induction damaged seminiferous tubular structure, characterized by displaced cells, the presence of intracellular vacuoles, and disrupted tubular basement membranes in some parts (Figure 1).
The histological examination of the testicular tissue in the control (A) and cholestasis (B) groups. Irregularity in the structure of seminiferous tubules and cellular vacuolation appeared in the testes of cholestasis rats. H & E staining; × 100.
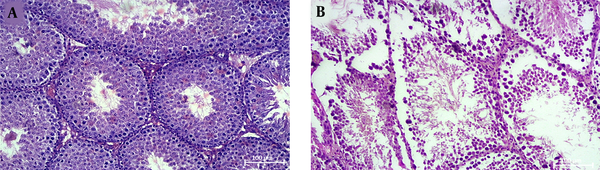
4.2. Liver Enzymes
The results showed that ALP, ALT, and AST liver enzymes significantly increased in the cholestasis group compared to the control group (P < 0.001), revealing a rise up to 17 folds four weeks after the surgery. These results indicated that cholestasis was successfully induced in animals (Figure 2).
Effects of cholestasis on liver enzymes. The results indicated significant elevations in the levels of AST (A), ALP (B), and ALT (C) liver enzymes in the cholestasis group compared with the control group (Mean ± SD; ***: P value < 0.001).
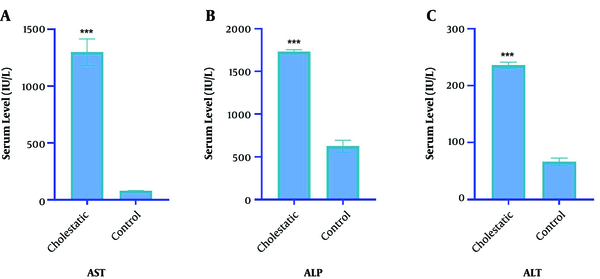
4.3. MiR-34c-5p Expression
The results of Real-Time PCR showed that the expression of miR-34c-5p significantly decreased in the cholestasis group compared with the control group (2-1/1125, P = 0.05) (Figure 3).
Effects of cholestasis on testicular miR-34-5p gene expression. Real-Time PCR showed a reduction in the expression of miR-34-5p in the cholestasis group compared to the control group (Mean ± SD; *: P value < 0.05).
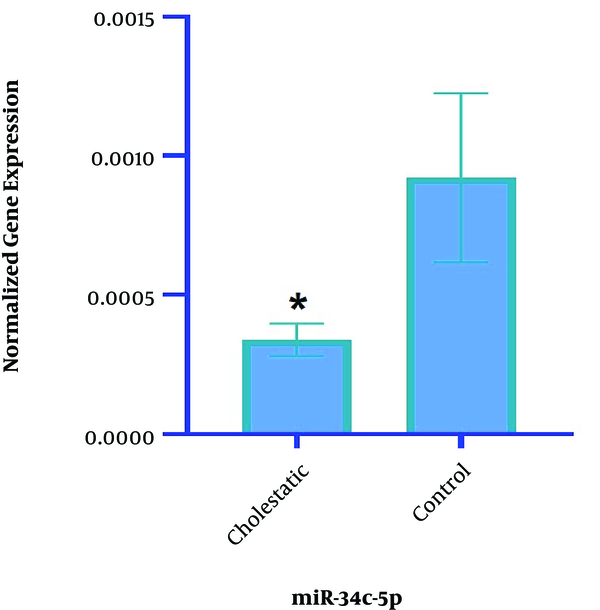
4.4. The Expression of MiR-34c-5p Target Genes
The impact of altered miR-34c-5p expression was assessed on the expressions of THY-1, FGF-2, and CASP-3, as its target genes, in both the control and cholestasis groups (Figure 4). The results showed that the expressions of THY-1 (2-2/0525, P = 0.01) and FGF-2 (2-1/7915, P = 0.02) markedly reduced in the cholestasis group compared with the control group. Inversely, the expression of the CASP-3 gene considerably increased in the cholestasis group compared with the control group (2+2/43, P = 0.00). Respective melting curves have been shown in Figure 5.
Real-Time PCR showed that Thy-1 (A) and Fgf-2 (B) genes’ expressions decreased while the expression of Casp3 (C) increased in the cholestasis group compared to the control group (Mean ± SD; *: P value < 0.05, **: P value < 0.01).
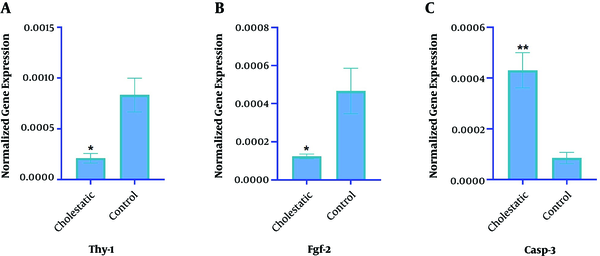
Melting curves of the genes assessed by Real-time PCR (miR-34c-5p (A), Fgf-2 (B), Casp-3 (C), Thy-1 (D), U6 (E), Gapdh (F)).
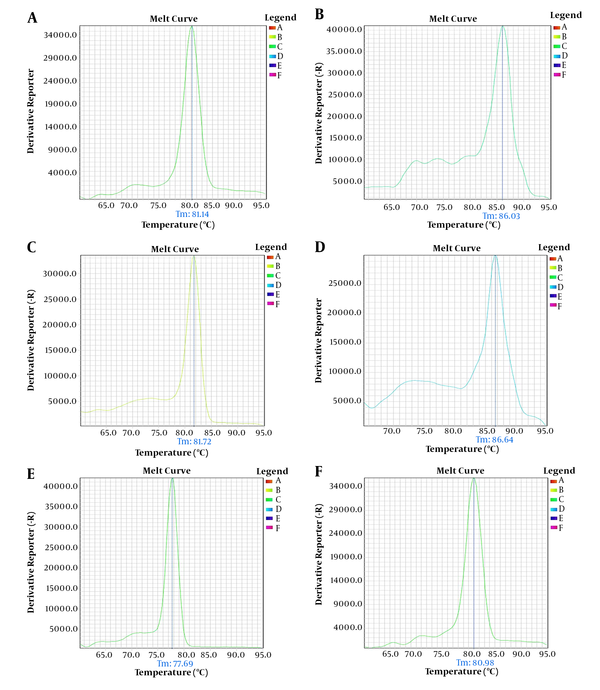
5. Discussion
Cholestasis causes the accumulation of BAs in the serum, and excessive amounts of BAs induce apoptosis in spermatids and germ cells through activating the Farnesoid X receptor alpha (FXRα) (22). In this context, similar to young mice, fxrα−/− male mice demonstrated a greater number of undifferentiated germ cells and fertility capacity (23). In the present study, to better understand the effects of cholestasis on testes, we examined the expression of miR-34c as one of the critical miRNAs involved in spermatogenesis. Our results indicated that the expression of miR-34c in the testicles of cholestasis rats decreased compared to controls. Several pieces of evidence, especially the role of inflammation, may explain this finding. One of the adverse effects of cholestasis on testes is infiltration by macrophages, subsequently leading to inflammation. Shi et al. demonstrated that after bile duct ligation, the number of cells expressing proliferating cells nuclear antigen (PCNA+) significantly reduced while the expressions of apoptosis-related genes such as CASP-3 and Bcl-2-associated X protein (BAX) elevated (24). Our finding also showed a higher expression of CASP-3, which could boost cellular death in testes of cholestasis rats. Two studies have shown that inflammation changes miR-34c expression in testes; the first study showed that six hours after receiving an intraperitoneal injection of an inflammation-inducing agent, bacterial lipopolysaccharide (LPS), the expressions of miRNAs, including miR-34c, gradually reduced in the testes of mice (25). The other study showed that miR34c-5p was significantly diminished in the testicular tissues of patients with cryptorchidism, as well as in murine models of cryptorchidism (26). In this regard, a previous study highlighted the presence of an inflammatory state in cryptorchid testes (27).
Our findings also demonstrated that cholestasis induction was accompanied by decreased THY-1 and FGF-2 expressions, supporting the hypothesis that miR-34c-5p had a regulatory site on the mentioned genes and the fact that altered miR-34c level might change THY-1 and FGF-2 expressions. The downregulation of THY-1 and FGF-2 genes could disrupt spermatogenesis in cholestasis animals. THY-1 is a marker for undifferentiated spermatogonia cells (28). A study performed by Ryu et al. showed that all spermatogonia stem cells in rats’ testicles expressed the THY-1 gene (29), and another study conducted by Yao et al. revealed that 90% of human spermatogonia were positive for this marker (30). A comparison between THY-1+ spermatogonia cells and THY-1− somatic cells revealed that miR-34c was highly expressed in the THY-1+ cell population (31). Likewise, FGF-2 encodes a growth factor secreted from various cell types in the testis (32) and is essential for normal spermatogenesis and sperm motility. It was noted that FGF-2-deficient mice were unable to produce normal spermatozoa (33). This factor is also necessary for the proliferation and differentiation of spermatogonia cells (34).
5.1. Conclusions
In conclusion, the current study revealed that cholestasis might affect the function of testes and spermatogenesis via dysregulating the expression of miR-34c. Considering the importance of miR-34c in several steps of sperm production, its dysregulation can be associated with the impaired expression of the genes needed for the development and viability of spermatocytes, such as THY-1 and FGF-2.
Acknowledgements
References
-
1.
Shi SH, Jiang L, Xie HY, Zhu YF, Zhang WJ, Zheng SS. Secondary biliary cholestasis promotes testicular macrophage infiltration and autophagy in rats. Am J Reprod Immunol. 2015;73(4):301-12. [PubMed ID: 25041469]. https://doi.org/10.1111/aji.12292.
-
2.
Kiani S, Valizadeh B, Hormazdi B, Samadi H, Najafi T, Samini M, et al. Alteration in male reproductive system in experimental cholestasis: roles for opioids and nitric oxide overproduction. Eur J Pharmacol. 2009;615(1-3):246-51. [PubMed ID: 19445924]. https://doi.org/10.1016/j.ejphar.2009.04.049.
-
3.
Ommati MM, Farshad O, Niknahad H, Arabnezhad MR, Azarpira N, Mohammadi HR, et al. Cholestasis-associated reproductive toxicity in male and female rats: The fundamental role of mitochondrial impairment and oxidative stress. Toxicol Lett. 2019;316:60-72. [PubMed ID: 31520699]. https://doi.org/10.1016/j.toxlet.2019.09.009.
-
4.
Macfarlane LA, Murphy PR. MicroRNA: Biogenesis, function and role in cancer. Curr Genomics. 2010;11(7):537-61. [PubMed ID: 21532838]. [PubMed Central ID: PMC3048316]. https://doi.org/10.2174/138920210793175895.
-
5.
Balasubramaniyan N, Devereaux MW, Orlicky DJ, Sokol RJ, Suchy FJ. Up-regulation of miR-let7a-5p leads to decreased expression of ABCC2 in obstructive cholestasis. Hepatol Commun. 2019;3(12):1674-86. [PubMed ID: 31832574]. [PubMed Central ID: PMC6887930]. https://doi.org/10.1002/hep4.1433.
-
6.
Song CW, Qiu W, Zhou XQ, Feng XC, Chen WS. Elevated hepatic MDR3/ABCB4 is directly mediated by MiR-378a-5p in human obstructive cholestasis. Eur Rev Med Pharmacol Sci. 2019;23(6):2539-47. [PubMed ID: 30964181]. https://doi.org/10.26355/eurrev_201903_17402.
-
7.
Salas-Huetos A, James ER, Aston KI, Carrell DT, Jenkins TG, Yeste M. The role of miRNAs in male human reproduction: A systematic review. Andrology. 2020;8(1):7-26. [PubMed ID: 31578810]. https://doi.org/10.1111/andr.12714.
-
8.
Procopio MS, de Avelar GF, Costa GMJ, Lacerda S, Resende RR, de Franca LR. MicroRNAs in Sertoli cells: implications for spermatogenesis and fertility. Cell Tissue Res. 2017;370(3):335-46. [PubMed ID: 28779347]. https://doi.org/10.1007/s00441-017-2667-z.
-
9.
Abu-Halima M, Hammadeh M, Backes C, Fischer U, Leidinger P, Lubbad AM, et al. Panel of five microRNAs as potential biomarkers for the diagnosis and assessment of male infertility. Fertil Steril. 2014;102(4):989-997 e1. [PubMed ID: 25108464]. https://doi.org/10.1016/j.fertnstert.2014.07.001.
-
10.
Abu-Halima M, Hammadeh M, Schmitt J, Leidinger P, Keller A, Meese E, et al. Altered microRNA expression profiles of human spermatozoa in patients with different spermatogenic impairments. Fertil Steril. 2013;99(5):1249-1255 e16. [PubMed ID: 23312218]. https://doi.org/10.1016/j.fertnstert.2012.11.054.
-
11.
Rokavec M, Li H, Jiang L, Hermeking H. The p53/miR-34 axis in development and disease. J Mol Cell Biol. 2014;6(3):214-30. [PubMed ID: 24815299]. https://doi.org/10.1093/jmcb/mju003.
-
12.
Yu M, Mu H, Niu Z, Chu Z, Zhu H, Hua J. miR-34c enhances mouse spermatogonial stem cells differentiation by targeting Nanos2. J Cell Biochem. 2014;115(2):232-42. [PubMed ID: 24038201]. https://doi.org/10.1002/jcb.24655.
-
13.
Wang X, Zhang P, Li L, Che D, Li T, Li H, et al. miRNA editing landscape reveals miR-34c regulated spermatogenesis through structure and target change in pig and mouse. Biochem Biophys Res Commun. 2018;502(4):486-92. [PubMed ID: 29864426]. https://doi.org/10.1016/j.bbrc.2018.05.197.
-
14.
Wang C, Yang C, Chen X, Yao B, Yang C, Zhu C, et al. Altered profile of seminal plasma microRNAs in the molecular diagnosis of male infertility. Clin Chem. 2011;57(12):1722-31. [PubMed ID: 21933900]. https://doi.org/10.1373/clinchem.2011.169714.
-
15.
Shah MA, Xu C, Kifayatullah SW, Yi C. Review: Role of microRNAs during spermatogenesis in mammals. Pure appl biol. 2018;7(2). https://doi.org/10.19045/bspab.2018.700101.
-
16.
Yang P, Chen D, Wang YX, Zhang L, Huang LL, Lu WQ, et al. Mediation of association between polycyclic aromatic hydrocarbon exposure and semen quality by spermatogenesis-related microRNAs: A pilot study in an infertility clinic. J Hazard Mater. 2020;384:121431. [PubMed ID: 31672436]. https://doi.org/10.1016/j.jhazmat.2019.121431.
-
17.
Xu C, Shah MA, Mipam T, Wu S, Yi C, Luo H, et al. Bovid microRNAs involved in the process of spermatogonia differentiation into spermatocytes. Int J Biol Sci. 2020;16(2):239-50. [PubMed ID: 31929752]. [PubMed Central ID: PMC6949159]. https://doi.org/10.7150/ijbs.38232.
-
18.
Saucedo L, Sobarzo C, Brukman NG, Guidobaldi HA, Lustig L, Giojalas LC, et al. Involvement of fibroblast growth factor 2 (FGF2) and its receptors in the regulation of mouse sperm physiology. Reproduction. 2018;156(2):163-72. [PubMed ID: 29866768]. https://doi.org/10.1530/REP-18-0133.
-
19.
Kubota H, Avarbock MR, Brinster RL. Spermatogonial stem cells share some, but not all, phenotypic and functional characteristics with other stem cells. Proc Natl Acad Sci U S A. 2003;100(11):6487-92. [PubMed ID: 12738887]. [PubMed Central ID: PMC164473]. https://doi.org/10.1073/pnas.0631767100.
-
20.
Metukuri MR, Reddy CM, Reddy PR, Reddanna P. Bacterial LPS-mediated acute inflammation-induced spermatogenic failure in rats: role of stress response proteins and mitochondrial dysfunction. Inflammation. 2010;33(4):235-43. [PubMed ID: 20087639]. https://doi.org/10.1007/s10753-009-9177-4.
-
21.
Heidari R, Mandegani L, Ghanbarinejad V, Siavashpour A, Ommati MM, Azarpira N, et al. Mitochondrial dysfunction as a mechanism involved in the pathogenesis of cirrhosis-associated cholemic nephropathy. Biomed Pharmacother. 2019;109:271-80. [PubMed ID: 30396085]. https://doi.org/10.1016/j.biopha.2018.10.104.
-
22.
Baptissart M, Vega A, Martinot E, Pommier AJ, Houten SM, Marceau G, et al. Bile acids alter male fertility through G-protein-coupled bile acid receptor 1 signaling pathways in mice. Hepatology. 2014;60(3):1054-65. [PubMed ID: 24798773]. https://doi.org/10.1002/hep.27204.
-
23.
Martinot E, Sedes L, Baptissart M, Holota H, Rouaisnel B, Damon-Soubeyrand C, et al. The bile acid nuclear receptor FXRalpha is a critical regulator of mouse germ cell fate. Stem Cell Reports. 2017;9(1):315-28. [PubMed ID: 28669602]. [PubMed Central ID: PMC5511114]. https://doi.org/10.1016/j.stemcr.2017.05.036.
-
24.
Shi SH, Jiang L, Xie HY, Xu J, Zhu YF, Zheng SS. The effect of secondary cholestasis on the CD68-positive and CD163-positive macrophage population, cellular proliferation, and apoptosis in rat testis. J Reprod Immunol. 2015;110:36-47. [PubMed ID: 25966619]. https://doi.org/10.1016/j.jri.2015.03.008.
-
25.
Parker MI, Palladino MA. MicroRNAs downregulated following immune activation of rat testis. Am J Reprod Immunol. 2017;77(6). e12673. [PubMed ID: 28328045]. https://doi.org/10.1111/aji.12673.
-
26.
Huang Z, Tang D, Gao J, Dou X, Cheng P, Peng D, et al. miR-34c disrupts spermatogonial stem cell homeostasis in cryptorchid testes by targeting Nanos2. Reprod Biol Endocrinol. 2018;16(1):97. [PubMed ID: 30322389]. [PubMed Central ID: PMC6190564]. https://doi.org/10.1186/s12958-018-0417-z.
-
27.
Duan Z, Huang H, Sun F. The functional and predictive roles of miR-210 in cryptorchidism. Sci Rep. 2016;6:32265. [PubMed ID: 27562222]. [PubMed Central ID: PMC5000482]. https://doi.org/10.1038/srep32265.
-
28.
Reding SC, Stepnoski AL, Cloninger EW, Oatley JM. THY1 is a conserved marker of undifferentiated spermatogonia in the pre-pubertal bull testis. Reproduction. 2010;139(5):893-903. [PubMed ID: 20154176]. https://doi.org/10.1530/REP-09-0513.
-
29.
Ryu BY, Orwig KE, Kubota H, Avarbock MR, Brinster RL. Phenotypic and functional characteristics of spermatogonial stem cells in rats. Dev Biol. 2004;274(1):158-70. [PubMed ID: 15355795]. https://doi.org/10.1016/j.ydbio.2004.07.004.
-
30.
Yao C, Yuan Q, Niu M, Fu H, Zhou F, Zhang W, et al. Distinct expression profiles and novel targets of MicroRNAs in human spermatogonia, pachytene spermatocytes, and round spermatids between OA patients and NOA patients. Mol Ther Nucleic Acids. 2017;9:182-94. [PubMed ID: 29246297]. [PubMed Central ID: PMC5645173]. https://doi.org/10.1016/j.omtn.2017.09.007.
-
31.
Niu Z, Goodyear SM, Rao S, Wu X, Tobias JW, Avarbock MR, et al. MicroRNA-21 regulates the self-renewal of mouse spermatogonial stem cells. Proc Natl Acad Sci U S A. 2011;108(31):12740-5. [PubMed ID: 21768389]. [PubMed Central ID: PMC3150879]. https://doi.org/10.1073/pnas.1109987108.
-
32.
Ishii K, Kanatsu-Shinohara M, Toyokuni S, Shinohara T. FGF2 mediates mouse spermatogonial stem cell self-renewal via upregulation of Etv5 and Bcl6b through MAP2K1 activation. Development. 2012;139(10):1734-43. [PubMed ID: 22491947]. https://doi.org/10.1242/dev.076539.
-
33.
Sedes L, Martinot E, Baptissart M, Baron S, Caira F, Beaudoin C, et al. Bile acids and male fertility: From mouse to human? Mol Aspects Med. 2017;56:101-9. [PubMed ID: 28511935]. https://doi.org/10.1016/j.mam.2017.05.004.
-
34.
Masaki K, Sakai M, Kuroki S, Jo JI, Hoshina K, Fujimori Y, et al. FGF2 has distinct molecular functions from GDNF in the mouse germline niche. Stem Cell Reports. 2018;10(6):1782-92. [PubMed ID: 29681540]. [PubMed Central ID: PMC5989648]. https://doi.org/10.1016/j.stemcr.2018.03.016.