Abstract
Background:
Novel nanoparticle drug therapy could be an effective method to enhance peripheral nerve regeneration.Objectives:
The current study aimed to determine the effect of Fe3O4 nanoparticles (NP) coated with omega-3 on sciatic nerve regeneration in Wistar rats.Methods:
The rats' left sciatic nerve was crushed with a non-serrated clamp. Animals in experimental groups were given Fe3O4@ω3 NPs (10 or 30 mg/kg/d) for one week. The recovery of functions was then assessed over eight weeks using a walking track analysis and a hot plate test. In addition, histomorphometry was used to assess the histomorphometric factors.Results:
Results showed that Fe3O4@ω3 NPs may have enhanced sciatic nerve regeneration and improved sensorimotor rehabilitation in comparison to the negative controls. These therapeutic effects were significantly greater in Fe3O4@ω3 NPs at a dose of 30 mg/kg. Furthermore, Fe3O4@ω3 NPs treatment, compared to negative controls, significantly reduced gastrocnemius muscle atrophy and improved histomorphometric parameters.Conclusions:
It was determined that Fe3O4@ω3 NPs had great potential for nerve regeneration due to the ease of their manufacturing and their desired efficacy.Keywords
1. Background
Since full functional recovery following severe nerve injury is uncommon, nerve injuries are regarded as major clinical issues. Following nerve injuries, particularly severe injuries, a cascade of secondary processes such as oxidative stress, inflammation, and excitotoxicity occurs, resulting in expanded injury and creating an inhibitory environment for the sprouting and elongation of injured axons (1). In this regard, inflammatory responses have been shown to play an important role in Wallerian degeneration, axonal regeneration, and functional rehabilitation (2). The immune response triggers a cascade of biological processes that remove growth-inhibiting components from the lesion site and create a favorable environment for axonal regeneration (3). As a result, modulating the inflammatory response may be an effective strategy for increasing the efficacy of nerve regeneration (4).
In this regard, animal research and clinical trials have determined that omega-3, a polyunsaturated fatty acid (PUFA), has anti-inflammatory effects, implying that they may be effective in the management of inflammatory responses (5). Furthermore, omega-3 fatty acids have been reported to display neuroprotective properties when dealing with a variety of neurological diseases (6). In our study, therefore, it was attempted to employ nanotechnology in order for developing a combined therapy method for healing peripheral nerve injury through application of iron oxide (Fe3O4) and Magnetite NPs coated with omega-3. As a carrier, the drug is attached to polymeric NPs, and the NPs facilitate therapeutic agent delivery and absorption into the target tissue (7). Iron oxide NPs have received considerable attention compared to other magnetic NPs due to their unique magnetic properties, biocompatibility, and low cytotoxicity (8).
2. Objectives
This study aimed to investigate how Fe3O4 NPs coated with omega-3 affected the sciatic nerve crush model in Wistar rats.
3. Methods
3.1. Synthesis of NPs
The Fe3O4@ω3 NPs were synthesized using the co-precipitation method as follows: Fe2+ (0.2 M, 30 mL) and Fe3+ (0.4 M, 30 mL) ions were stirred for 10 minutes at 80°C. Then, NaOH solution (4.5 M, 30 mL) was added to the iron ions abruptly and stirred for 10 minutes. Omega-3 oil (2mL) was added to the reaction medium drop-by-drop and stirred for 60 minutes. The dark precipitation was washed and dried at 50°C.
3.2. Characterization of NPs
The synthesized NPs were analyzed by X-Ray diffraction, FT-IR spectrophotometer, VSM, and TEM.
3.3. Animals and Groups
A total of 40 adult male Wistar rats (240 ± 20 g) were purchased and kept in plexiglass with standard condition. They were randomly allocated into four groups. Rats in two experimental groups were given Fe3O4@ω3 NPs intraperitoneally at doses of 10 or 30 mg/kg daily for one week (8). Also, animals in the negative control group underwent crushing and received deionized water as a vehicle. In the sham group, only the sciatic nerve was exposed. All animal experiments were approved by the Ethics Committee of Mohaghegh Ardabili University of Ardabil (Iran) under code number: IR.ARUMS.REC.1400.084.
3.4. Surgical Procedures
The rats' right sciatic nerves were operated under deep anesthesia. Then, sciatic nerves of the rats’ right leg were operated and compressed for 30 seconds. Then, the rats’ muscles and skin were sutured.
3.5. Walking Tract Analysis
The sciatic functional index (SFI) was measured at two, four, six, and eight weeks after surgery according to the previous method (9, 10).
3.6. Hot Plate Test
A hot plate latency test was performed to evaluate the sensory recovery. Tests were performed at 2, 4, 6, and 8 weeks after surgery. The temperature was set at 54°C. Then, the rats' operated legs were located on a hot plate, and their reaction was recorded as response latency.
3.7. Histomorphometric Analysis
Histomorphometric analysis of regenerated nerves was carried out eight weeks after surgery according to the previous method (10).
3.8. Histological Evaluation of Gastrocnemius Muscle
Gastrocnemius muscles from all groups were harvested, and then Masson's trichrome staining was used to assess the severity of muscle atrophy.
3.9. Gastrocnemius Muscle Mass Ratio
Gastrocnemius muscle samples of all groups were dissected and harvested. Then, their wet weights were calculated using a Pohl Schmitt digital scale (Switzerland).
3.10. Statistical Analysis
SPSS Statistics 16.0 software was used to analyze the statistical data, and all data were presented as a mean ± SEM. The P-values lower than 0.05 were considered significant. Statistical significance between groups was determined by using ANOVA test and Tukey post hoc test.
4. Results
4.1. Characterization of NPs
4.1.1. XRD Patterns
XRD patterns confirmed the cubic spinel structure of the bare and ω3 coated magnetite NPs (Figure 1). The appeared peaks at 2θ ~ 30.48, 35.78, 43.53, 53.83, 57.38, and 63.03 relating to the crystal planes of (220), (311), (400), (422), (511), and (440), respectively, were consistent with JCPD card no. 19-0629. The average crystallite sizes of the Fe3O4 and Fe3O4@ω3 were measured by Debye-Scherrer equation:
And were found to be about 13 and 7 nm, respectively. In addition, the lattice constant of the coated particles was nearly 8.324 Å, which was decreased proportional to the bare one (8.376 Å).
XRD Patterns of the Fe3O4 and Fe3O4@ω3 NPs
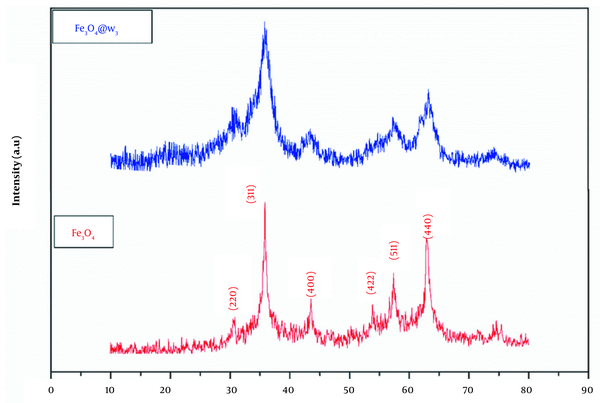
4.2. TEM and SEM Images
Figure 2A and B displays the TEM and SEM images of the Fe3O4@ω3 NPs. Obviously, NPs had an approximately uniform size distribution, and their size was smaller than 10 nm, which was in close agreement with XRD data (Figure 2A). According to both the TEM and SEM images, particles appeared to be quasi-spherical, and some aggregation was observed due to their magnetic nature.
A, TEM; and B, SEM Images of the Fe3O4@ω3 NPs.
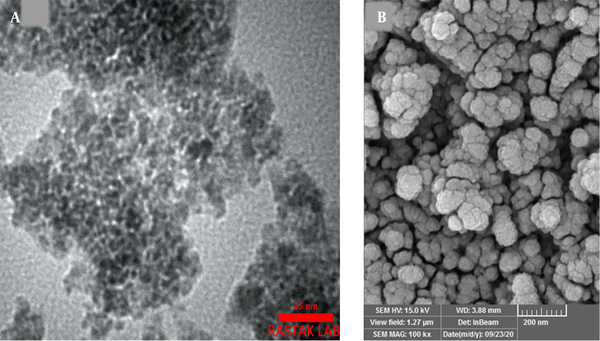
4.3. FT-IR Characterization
FT-IR spectra of the ω3 and Fe3O4@ω3 samples are presented in Appendix 1. The spectrum of ω3 exhibited absorption bands between 1000 - 1500 cm-1 that were related to C-O and C-H vibrations. An absorption band occurred at 1745 cm-1 which corresponded to the C=O vibration. In addition, the stretching vibrations of the -C-H (CH2) bands were observed at 2855 and 2924 cm-1. The spectrum of Fe3O4@ω3 presented two absorption deeps at 445 and 625 cm-1 that were in accordance with Fe-O vibrations in the spinel structure. Furthermore, the absorption band of C=O became very weak and the characteristic band of the C-O vibration shifted to the lower frequencies, which confirmed the interaction between ω3 and the surface of magnetite NPs.
4.4. VSM Characterization
The magnetic properties of the bare and coated magnetite NPs were measured using VSM at room temperature. According to Appendix 2, both samples are superparamagnetic with nearly zero coercivity. Due to the very small size of the particles and the biocompatible shell on their surface, moreover, the saturation magnetization of the omega-3 loaded NPs was decreased proportional to the bare one.
4.5. Motor Functional Recovery Evaluation
Results showed that the SFI values in all groups were reduced after surgery (Figure 3). Then, SFI values in all groups were increased progressively, with a significantly higher improvement detected in Fe3O4@ω3 NP groups (P < 0.05; Figure 3) in increased progressively with the negative control group. Also, the best nerve recovery in comparison with the negative control group was seen in Fe3O4@ω3 NP (30 mg/kg) group six weeks after surgery (P < 0.05; Figure 3).
The motor functional recovery evaluation using SFI value [SFI was evaluated in all experimental groups after crush. Values are presented as mean ± standard error of the mean (n = 10); *P < 0.05, **P < 0.01 and ***P < 0.001, respectively].
![The motor functional recovery evaluation using SFI value [SFI was evaluated in all experimental groups after crush. Values are presented as mean ± standard error of the mean (n = 10); *P < 0.05, **P < 0.01 and ***P < 0.001, respectively]. The motor functional recovery evaluation using SFI value [SFI was evaluated in all experimental groups after crush. Values are presented as mean ± standard error of the mean (n = 10); *P < 0.05, **P < 0.01 and ***P < 0.001, respectively].](https://services.brieflands.com/cdn/serve/31645/0bd78cefa262fab36ee61fe3a98f12a32a1c659d/gct-10-2-124110-i003-preview.png)
4.6. Sensory Neuron Recovery Evaluation
Rats in all groups showed no reaction to the hot plate two weeks after surgery, except for the sham group which reacted after 0.97 seconds. Sensory recovery after nerve surgery (4, 6, and 8 weeks) improved gradually in all groups, with a noticeably faster recovery detected in the Fe3O4@ω3 NPs groups (P < 0.05; Figure 4).
Sensory recovery test. The hot plate test was performed in each experimental group after crush. All data are presented as mean ± standard error of the mean (n = 10) (*P < 0.05, **P < 0.01 and ***P < 0.001, respectively).
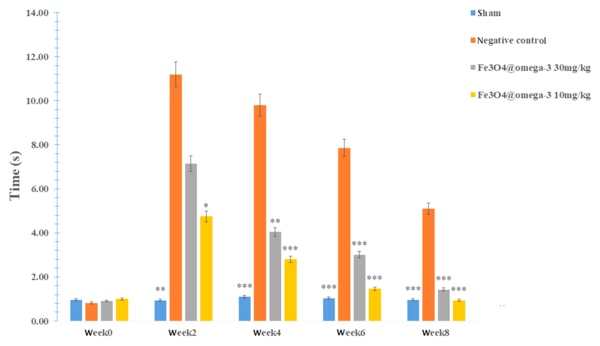
4.7. Histomorphometric Analysis
Table 1 shows the histomorphometric parameters. The results showed that the Fe3O4@ω3 NPs treatment groups had significantly higher mean fiber numbers than the negative control and sham surgery groups. This increase, however, was significantly lower in the sham group. On the other hand, Fe3O4@ω3 NPs (30 mg/kg/day) treated rats had considerably higher fiber number, fiber diameter, and axon diameters than Fe3O4@ω3 NPs (10 mg/kg/day) treated animals (Appendix 3).
Groups | Fiber Count | Fiber Diameter | Axon Diameter | Myelin Thickness |
---|---|---|---|---|
Sham surgery | 5241 ± 24 | 5.41 ± 0.31 | 4.16 ± 0.12 | 1.25 ± 0.11 |
Negative control | 6321 ± 26** | 2.61 ± 0.41** | 1.88 ± 0.41** | 0.73 ± 0.62** |
Fe3O4@ω3 NPs (10 mg/kg) | 7121 ± 38** | 4.23 ± 0.31* | 3.22 ± 0.22* | 1.01 ± 0.06** |
Fe3O4@ω3 NPs (30 mg/kg) | 8951 ± 44*** | 4.91 ± 0.11* | 3.82 ± 0.13 | 1.09 ± 0.21** |
4.8. Histological Evaluation of Gastrocnemius Muscle
As shown in appendix 4, the muscle fibers were stained in red, whereas the collagen fibers showed the blue staining. Denervated muscles in nerve crush groups displayed varying degrees of atrophy compared to those in the sham surgery group. However, the atrophy in the Fe3O4@ω3 NPs-treated groups was significantly lower in the negative control group.
4.9. Gastrocnemius Muscle Mass Ratio
The results showed that the muscle mass ratios in Fe3O4@ω3 NPs (30 mg/kg/day) treated rats were substantially greater in comparison to those in the negative group (P < 0.05). However, no discernible change was observed between the Fe3O4@ω3 NPs (10 mg/kg/day) treated with the negative control and the Fe3O4@ω3 NPs (30 mg/kg/day) groups (Appendix 5).
5. Discussion
The administration of neuroprotective medications after nerve injuries is an effective method for limiting damage and enhancing nerve regeneration. Utilizing nanotechnology to synthesize neuroprotective agents can improve pharmacotherapy efficacy (7, 11). In this regard, magnetic NPs such as Fe3O4 are effective nano systems for managing drug delivery (12, 13). In addition, magnetic NPs have a synergistic impact on the target site, improve therapeutic outcomes, and prevent drug degradation (14, 15). Our findings revealed that one-week treatment with Fe3O4@ω3 NPs (10 or 30 mg/kg) after a sciatic nerve injury had the potential to increase the nerve regeneration. Walking track analysis demonstrated that Fe3O4@ω3 NPs at 10 or 30 mg/kg doses were effective in enhancing motor recovery. However, only Fe3O4@ω3 NPs at a dose of 30 mg/kg were capable of increasing SFI values significantly compared to the negative control group. Moreover, muscle mass ratio and histomorphometric parameters were also considerably improved in Fe3O4@ω3 NPs at 30 mg/kg compared to negative control, confirming the results from the walking track analysis. Furthermore, the hot plate test results suggested that Fe3O4@ω3 NPs-treated (10 or 30 mg/kg) therapy reduced the sensory threshold.
According to our findings, increased tissue levels of omega-3 using Fe3O4 as a carrier improved sciatic nerve regeneration in a rat crush model. Omega-3 fatty acids cannot be produced in the body and must therefore be obtained from diets or supplements (16). Omega-3 has been proven to have many biological effects, including boosting the synthesis of anti-inflammatory and neuroprotective metabolites via prostaglandin metabolism (17). On the other hand, animal research have indicated that omega-3 fatty acids may contribute to neuronal survival and axonal healing following peripheral nerve injury (18). In an in vitro study, Robson et al. reported that omega-3 PUFA had a significant neurite-promoting capacity in neurons from adult and aged animals (19). In a study by Baazm et al. to evaluate the protective effects of parenteral administration of omega-3 on spinal cord injury in rats, it was discovered that omega-3 treatment increased motor function recovery and reduced inflammation (20). Pu et al investigated the neuroprotective effects of a 2-month omega-3 polyunsaturated fatty acid (PUFA)-enriched diet against controlled cortical impact (CCI) in mice, and found that an omega-3-rich diet reduced behavioral deficits (21). Furthermore, several clinical trials on diabetic patients have shown that long-term omega-3 diets can improve diabetic neuropathy, promote nerve regeneration, and restore sensory function (22). Omega-3 appears to prevent oxidative stress-induced apoptosis by decreasing pro-apoptotic signaling and suppressing pro-inflammatory responses (23).
On the other hand, some researchers have found that NPs can effectively promote the growth of injured axons (24, 25). Liu et al., for instance, investigated the role of nano-hydroxyapatite in regeneration in cultured brain cells of rats. According to their findings, nano-hydroxyapatite induced axonal guidance growth by regulating netrin-1 expression as an axonal guidance cue during nerve regeneration. Furthermore, some studies have indicated that a single application of Fe3O4 can cause significant axonal guidance, but it has a cytotoxic effect on cell viability. When nanohydroxyapatite-coated magnetic Fe3O4 (Fe3O4n-HA) particles were added to primary cultured DRG neurons in our study, however, the cytotoxicity of single magnetic NPs Fe3O4 was reduced. Moreover, Fe3O4n-HA was able to efficiently direct axonal guidance and greatly promote axonal elongation (26). Chen et al. also investigated electrospinning multilayered scaffolds loaded with melatonin and Fe3O4 magnetic NPs in order for repairing sciatic nerve defects in rats, and found that scaffolds had the adequate biocompatibility and potential to improve morphological, functional, and electrophysiological parameters. Other studies have determined that NPs are capable of improving the mechanical properties of neural conduits (27). In some experiments, Fe3O4 NPs have also been shown to increase neurite outgrowth by stimulating the mitogen-activated protein kinase signaling pathway (28).
5.1. Conclusion
In sum, it was found that a one-week treatment of Fe3O4@ω3 NPs rats with sciatic nerve defects may have improved the regeneration process and rehabilitation. Our study results confirmed the neuroprotective effects of Fe3O4@ω3 NPs as new drug. It was also demonstrated that Fe3O4@ω3 NPs had great potential for nerve regeneration due to the ease of their manufacturing and their desired efficacy. However, it was recommended that further studies should be conducted in order to confirm our findings.
5.2. Limitation
This study faced some limitations, one of which was the lack of molecular data about the expression of neurotrophic factors in drug-treated groups.
References
-
1.
Mohammad-Bagher G, Arash A, Morteza BR, Naser MS, Ali M. Synergistic Effects of Acetyl-l-Carnitine and Adipose-Derived Stromal Cells on Improving Regenerative Capacity of Acellular Nerve Allograft in Sciatic Nerve Defect. J Pharmacol Exp Ther. 2019;368(3):490-502. [PubMed ID: 30591528]. https://doi.org/10.1124/jpet.118.254540.
-
2.
Fregnan F, Muratori L, Simoes AR, Giacobini-Robecchi MG, Raimondo S. Role of inflammatory cytokines in peripheral nerve injury. Neural Regen Res. 2012;7(29):2259-66. [PubMed ID: 25538747]. [PubMed Central ID: PMC4268726]. https://doi.org/10.3969/j.issn.1673-5374.2012.29.003.
-
3.
Gaudet AD, Popovich PG, Ramer MS. Wallerian degeneration: gaining perspective on inflammatory events after peripheral nerve injury. J Neuroinflammation. 2011;8:110. [PubMed ID: 21878126]. [PubMed Central ID: PMC3180276]. https://doi.org/10.1186/1742-2094-8-110.
-
4.
Trias E, Kovacs M, King PH, Si Y, Kwon Y, Varela V, et al. Schwann cells orchestrate peripheral nerve inflammation through the expression of CSF1, IL-34, and SCF in amyotrophic lateral sclerosis. Glia. 2020;68(6):1165-81. [PubMed ID: 31859421]. [PubMed Central ID: PMC7269115]. https://doi.org/10.1002/glia.23768.
-
5.
Balic A, Vlasic D, Zuzul K, Marinovic B, Bukvic Mokos Z. Omega-3 Versus Omega-6 Polyunsaturated Fatty Acids in the Prevention and Treatment of Inflammatory Skin Diseases. Int J Mol Sci. 2020;21(3). [PubMed ID: 31979308]. [PubMed Central ID: PMC7037798]. https://doi.org/10.3390/ijms21030741.
-
6.
Bi J, Chen C, Sun P, Tan H, Feng F, Shen J. Neuroprotective effect of omega-3 fatty acids on spinal cord injury induced rats. Brain Behav. 2019;9(8). e01339. [PubMed ID: 31225705]. [PubMed Central ID: PMC6710194]. https://doi.org/10.1002/brb3.1339.
-
7.
Soluki M, Mahmoudi F, Abdolmaleki A, Asadi A. [The Protective Effects of Nanoparticles in the Treatment of Nervous System Injuries: A Narrative Review]. J Rafsanjan Univ Med Sci. 2021;20(9):1027-48. Persian. https://doi.org/10.52547/jrums.20.9.1027.
-
8.
Naserzadeh P, Hafez AA, Abdorahim M, Abdollahifar MA, Shabani R, Peirovi H, et al. Curcumin loading potentiates the neuroprotective efficacy of Fe3O4 magnetic nanoparticles in cerebellum cells of schizophrenic rats. Biomed Pharmacother. 2018;108:1244-52. [PubMed ID: 30453447]. https://doi.org/10.1016/j.biopha.2018.09.106.
-
9.
Abdolmaleki A, Zahri S, Bayrami A. Rosuvastatin enhanced functional recovery after sciatic nerve injury in the rat. Eur J Pharmacol. 2020;882:173260. [PubMed ID: 32534070]. https://doi.org/10.1016/j.ejphar.2020.173260.
-
10.
Soluki M, Mahmoudi F, Abdolmaleki A, Asadi A, Sabahi Namini A. Cerium oxide nanoparticles as a new neuroprotective agent to promote functional recovery in a rat model of sciatic nerve crush injury. Br J Neurosurg. 2020:1-6. [PubMed ID: 33356586]. https://doi.org/10.1080/02688697.2020.1864292.
-
11.
Naqvi S, Panghal A, Flora SJS. Nanotechnology: A Promising Approach for Delivery of Neuroprotective Drugs. Front Neurosci. 2020;14:494. [PubMed ID: 32581676]. [PubMed Central ID: PMC7297271]. https://doi.org/10.3389/fnins.2020.00494.
-
12.
Shen L, Li B, Qiao Y. Fe(3)O(4) Nanoparticles in Targeted Drug/Gene Delivery Systems. Materials (Basel). 2018;11(2). [PubMed ID: 29473914]. [PubMed Central ID: PMC5849021]. https://doi.org/10.3390/ma11020324.
-
13.
Chen Y, Chen H, Shi J. Inorganic nanoparticle-based drug codelivery nanosystems to overcome the multidrug resistance of cancer cells. Mol Pharm. 2014;11(8):2495-510. [PubMed ID: 24224544]. https://doi.org/10.1021/mp400596v.
-
14.
Pankhurst QA, Connolly J, Jones SK, Dobson J. Applications of magnetic nanoparticles in biomedicine. J Phys D Appl Phys. 2003;36(13):R167.
-
15.
Gholami A, Asadi A, Abdolmaleki A, Zahri S. [Evaluating the Efficiency of Selenium Nanoparticles in the Production of Decellularized Neural Scaffold and the Ability to Preserve Stem Cells Cultured on Them: A Laboratory Study]. J Rafsanjan Univ Med Sci. 2021;20(7):733-46. Persian.
-
16.
Simopoulos AP. Omega-3 fatty acids in health and disease and in growth and development. Am J Clin Nutr. 1991;54(3):438-63. [PubMed ID: 1908631]. https://doi.org/10.1093/ajcn/54.3.438.
-
17.
Giacobbe J, Benoiton B, Zunszain P, Pariante CM, Borsini A. The Anti-Inflammatory Role of Omega-3 Polyunsaturated Fatty Acids Metabolites in Pre-Clinical Models of Psychiatric, Neurodegenerative, and Neurological Disorders. Front Psychiatry. 2020;11:122. [PubMed ID: 32180741]. [PubMed Central ID: PMC7059745]. https://doi.org/10.3389/fpsyt.2020.00122.
-
18.
Unda SR, Villegas EA, Toledo ME, Asis Onell G, Laino CH. Beneficial effects of fish oil enriched in omega-3 fatty acids on the development and maintenance of neuropathic pain. J Pharm Pharmacol. 2020;72(3):437-47. [PubMed ID: 31876957]. https://doi.org/10.1111/jphp.13213.
-
19.
Robson LG, Dyall S, Sidloff D, Michael-Titus AT. Omega-3 polyunsaturated fatty acids increase the neurite outgrowth of rat sensory neurones throughout development and in aged animals. Neurobiol Aging. 2010;31(4):678-87. [PubMed ID: 18620782]. https://doi.org/10.1016/j.neurobiolaging.2008.05.027.
-
20.
Baazm M, Behrens V, Beyer C, Nikoubashman O, Zendedel A. Regulation of Inflammasomes by Application of Omega-3 Polyunsaturated Fatty Acids in a Spinal Cord Injury Model. Cells. 2021;10(11). [PubMed ID: 34831370]. [PubMed Central ID: PMC8618254]. https://doi.org/10.3390/cells10113147.
-
21.
Pu H, Guo Y, Zhang W, Huang L, Wang G, Liou AK, et al. Omega-3 polyunsaturated fatty acid supplementation improves neurologic recovery and attenuates white matter injury after experimental traumatic brain injury. J Cereb Blood Flow Metab. 2013;33(9):1474-84. [PubMed ID: 23801244]. [PubMed Central ID: PMC3764381]. https://doi.org/10.1038/jcbfm.2013.108.
-
22.
Lewis EJH, Lovblom LE, Cisbani G, Chen DK, Bazinet RP, Wolever TMS, et al. Baseline omega-3 level is associated with nerve regeneration following 12-months of omega-3 nutrition therapy in patients with type 1 diabetes. J Diabetes Complications. 2021;35(3):107798. [PubMed ID: 33309385]. https://doi.org/10.1016/j.jdiacomp.2020.107798.
-
23.
Gordon WC, Bazan NG. Mediator lipidomics in ophthalmology: targets for modulation in inflammation, neuroprotection and nerve regeneration. Curr Eye Res. 2013;38(10):995-1005. [PubMed ID: 23981028]. https://doi.org/10.3109/02713683.2013.827211.
-
24.
Hong Q, Song H, Chi NTL, Brindhadevi K. Numerous nanoparticles as drug delivery system to control secondary immune response and promote spinal cord injury regeneration. Process Biochem. 2022;112:145-53. https://doi.org/10.1016/j.procbio.2021.11.025.
-
25.
Escobar A, Reis RL, Oliveira JM. Nanoparticles for neurotrophic factor delivery in nerve guidance conduits for peripheral nerve repair. Nanomedicine (Lond). 2022;17(7):477-94. [PubMed ID: 35220756]. https://doi.org/10.2217/nnm-2021-0413.
-
26.
Liu M, Zhou G, Hou Y, Kuang G, Jia Z, Li P, et al. Effect of nano-hydroxyapatite-coated magnetic nanoparticles on axonal guidance growth of rat dorsal root ganglion neurons. J Biomed Mater Res A. 2015;103(9):3066-71. [PubMed ID: 25690555]. https://doi.org/10.1002/jbm.a.35426.
-
27.
Chen X, Ge X, Qian Y, Tang H, Song J, Qu X, et al. Electrospinning Multilayered Scaffolds Loaded with Melatonin and Fe3O4 Magnetic Nanoparticles for Peripheral Nerve Regeneration. Adv Funct Mate. 2020;30(38):200453. https://doi.org/10.1002/adfm.202004537.
-
28.
Kim JA, Lee N, Kim BH, Rhee WJ, Yoon S, Hyeon T, et al. Enhancement of neurite outgrowth in PC12 cells by iron oxide nanoparticles. Biomaterials. 2011;32(11):2871-7. [PubMed ID: 21288566]. https://doi.org/10.1016/j.biomaterials.2011.01.019.