Abstract
Background:
Regarding the increasing resistance of bacteria against antibiotics and the existence of antibacterial compounds in plants, we here investigated the antimicrobial effects of the methanolic extracts of several plants on pathogenic bacteria.Methods:
Ten grams of the dry ingredients of Eryngium caucasicum trautv (leaf), Saponaria officinalis (flower), Froriepia subpinnata (leaf), Malva sylvestris (leaf), and Urtica dioica (leaf) was poured in 100 mL of methanol and shaken for 24 hours. After 24 hours, the extracts were strained and dried in the oven, and their antimicrobial activity at the 100 mg/mL concentration was evaluated against the mentioned bacteria using the sinkhole method.Results:
The plant extracts inhibited the growth of all bacteria in various degrees. Among all the plant extracts used, that of F. subpinnata delivered the largest diameter of growth inhibition zone (6 mm) against S. dysenteriae, and the extracts of E. caucasicum and F. subpinnata showed the largest diameter of inhibition zone against Xanthomonas translucens. Also, the extract of M. sylvestris delivered the largest inhibition zone diameter (8 mm) against E. coli.Conclusions:
Our results showed that the methanolic extract of E. caucasicum was the most effective plant extract against S. dysenteriae, Rathayibacter tritici, and L. monocytogenes, but M. sylvestris extract revealed the greatest impact on E. coli. It is recommended to further investigate the antibacterial compounds of these plants as potential treatments for bacterial infections.Keywords
Medicinal Plants Antimicrobial Activity Bacterial Strains Non-growth Inhibition Zone Diameter
1. Background
Infectious diseases cause premature deaths in thousands of people worldwide every day (1-4). Numerous commercial antibiotics are used worldwide to control human infections and infectious diseases. The long-term use of these antibiotics has caused the incidence of bacterial resistance to several drugs, leading to serious problems in the treatment of infectious diseases (5-7). Therefore, it is necessary to conduct extensive research to discover new antimicrobial substances from various herbal and animal sources. Medicinal plants have grabbed special interest as a potential source of new antimicrobials due to their substances with low toxicity. During their secondary metabolism, plants make many compounds with complex molecular structures, some of which show potent antimicrobial properties (8-10).
Saponaria officinalis, known as Coven, is an herbaceous perennial plant with a height of 30 - 100 cm. This plant grows in wet ditches, on roadsides, in areas of waste disposal, near old houses, and in pastures. This plant is also grown as an ornamental plant. Saponaria officinalis contains large amounts of saponins, which foams during extraction with water. This plant has been used as an alternative medicine since the time of Dioscorides. This plant can also be used as an anti-scrofula, calagogue, expectorant, diaphoretic, weak diuretic, expectorant, cleanser, and tonic agent. The decoction derived from this plant is locally applied to treat skin itching (11, 12).
Malva sylvestris, with the scientific name M. sylvestris L, belongs to the Malvaceae family and is a medicinal plant endemic to Iran. This plant has high nutritional and medicinal value. All parts of this plant have medicinal uses, but its flowers and leaves are more commonly applied in Iranian traditional medicine to treat diseases. This plant possesses numerous biological properties, including antiseptic, oral wound healing, analgesic, anti-inflammatory, antioxidant, and antibacterial properties, inhibiting the growth of staphylococci and streptococci. Also, mouthwashes containing the leaf extract of this plant have been used as a treatment for oral lesions and inflammation due to the presence of mucilage, flavonoids, and tannins (13-15).
Pomegranate, with the scientific name of Froriepia subpinnata, belongs to the Punicaceae family. Other common names for this plant include grenade, geranat, and Punica apple. Froriepia subpinnata has been widely used as a traditional medicine in many countries to treat dysentery, diarrhea, worm infections, acidosis, bleeding, and respiratory diseases. In addition, F. subpinnata has antioxidant, anti-atherostatic, antimicrobial, and antiviral properties. The constituents of P. granatum include gallocatechin, delphinidin, cyanidin, gallic acid, ellagic acid, pelargonidin, and sitosterol, which are well-known due to their therapeutic properties (16-18).
Urtica dioica, with the scientific name of U. dioca L, is an herbaceous perennial flowering plant that grows all over the world. This plant belongs to the Urticaceae family and is considered an important medicinal plant because of its high amounts of minerals (iron, manganese, potassium, and calcium), chlorophyll, amino acids, carotenoids, vitamins, flavonoids, tannins, sterols, polysaccharides, and cholestin (19, 20).
Regarding the increasing rate of bacterial resistance against antibiotics, these plants, due to their antibacterial compounds, can be valuable sources for developing novel antimicrobial agents.
2. Objectives
We here investigated the effects of the methanol extracts of these plants on the growth of a number of pathogenic bacteria.
3. Methods
The methanolic extracts of E. caucasicum trautv (leaf), S. officinalis (flower), F. subpinnata (leaf), M. sylvestris (leaf), and U. dioica (leaf) were prepared. The plants were collected from the northern regions of Iran in spring (2022). Then the samples were dried and milled under shade at room temperature. In order to prepare the plant extracts using the cold maceration method, 20 grams of powdered plant leaves were soaked separately in methanol and transferred to a shaker for 24 hours, followed by filtering through Whatman No. 2 filter paper (21, 22). Then the solvent was removed from the filtered material using a rotary vacuum device. The concentrated extract was kept for 48 hours in an oven at 40 degrees Celsius until obtaining pure extracts and the complete removal of the solvent. Finally, the obtained extracts were dried, weighed, and kept in the refrigerator at 4 degrees Celsius until further testing (23, 24).
3.1. Bacterial Strains and Culture Condition
Bacterial strains, including S. dysenteriae, R. tritici, E. coli, and L. monocytogenes, were obtained from the Laboratory of the Veterinary Medicine Department of the University of Zabol, propagated on nutrient agar culture medium, and kept in a refrigerator at 4 degrees Celsius until use. Bacterial suspensions were prepared from fresh and young bacterial cultures by transferring several colonies to the Mueller Hinton Broth culture medium (25).
The turbidity of the prepared microbial suspensions was adjusted to 0.5 McFarland standard (corresponding to 1.5 × 108 bacteria per mL) by setting light absorption at 630 nm in the range of 0.08 to 0.1. A concentration of 1.5× 107 bacteria per milliliter was obtained by diluting the 0.5 McFarland bacterial suspension by one-tenth (26, 27). The antimicrobial effects of the plant extracts at a concentration of 100 mg/mL were investigated using the agar diffusion method. A sterile swab was soaked in the bacterial suspension with turbidity equal to 1.5 × 107 bacteria per mL and was used to uniformly culture bacteria on Mueller Hinton agar. Then several sinkholes with a diameter of 6 mm and a depth of 5 mm were created at suitable intervals, and 100 microliters of the extracts were poured into the sinkholes. Ciprofloxacin antibiotic was used as a positive control. After 24 hours of incubation at 37°C, the diameter of the growth inhibition zone was measured in mm. All the experiments were repeated three times to obtain accurate results (28).
4. Results
The results of this study showed that the extracts of E. caucasicum trautv, S. officinalis, M. sylvestris, U. dioica, and F. subpinnata had variable growth inhibitory effects on S. dysenteriae, R. tritici, E. coli, and L. monocytogenes (P < 0.01, Table 1). Among all the plants used, F. subpinnata had the largest diameter of inhibition zone (6 mm) against S. dysenteriae (Figure 1). Also, F. subpinnata extract showed the largest inhibition zone diameter (4 mm) against R. tritici (Figure 2). The extracts of E. caucasicum and F. subpinnata revealed the largest diameter of the inhibition zone (3 mm) against Xanthomonas translucens (Figure 3). The largest inhibition zone diameter (8 mm) of M. sylvestris was observed against E. coli (Figure 4), and the extracts of E. caucasicum and U. dioica caused the largest diameter of inhibition zone (5 mm) against L. monocytogenes (Figure 5).
Variance Analysis of Bacterial Growth Inhibition Zone at a Concentration of 100 mg/mL of the Methanolic Extracts of the Plant Studied
The inhibition zone diameter observed after treatment with plant methanol extracts at a concentration of 100 mg/mL against S. dysenteriae. ** Significant at 0.01 level of probability.
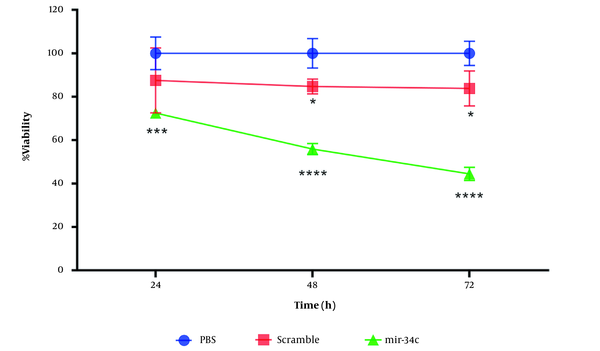
The inhibition zone diameter observed after treatment with plant methanol extracts at a concentration of 100 mg/mL against R. tritici. ** Significant at 0.01 level of probability.
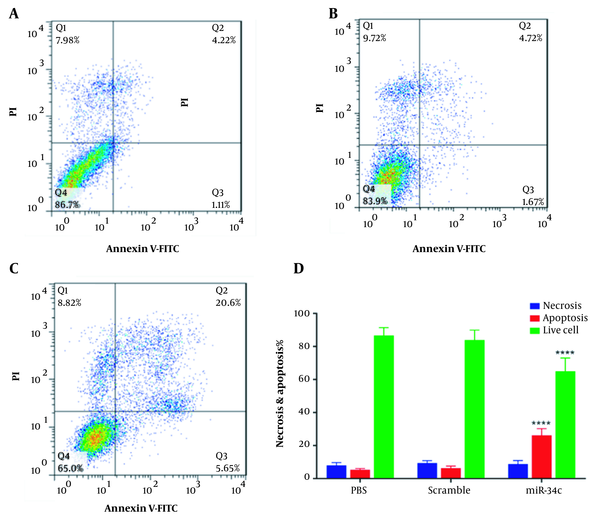
The inhibition zone diameter was observed after treatment with plant methanol extracts at a concentration of 100 mg/mL against Xanthomonas translucens. ** Significant at 0.01 level of probability.
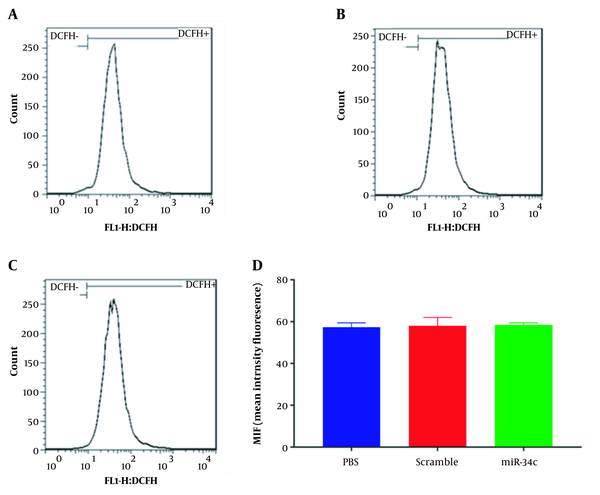
The inhibition zone diameter after treatment with plant methanol extracts at a concentration of 100 mg/mL against E. coli. ** Significant at 0.01 level of probability.
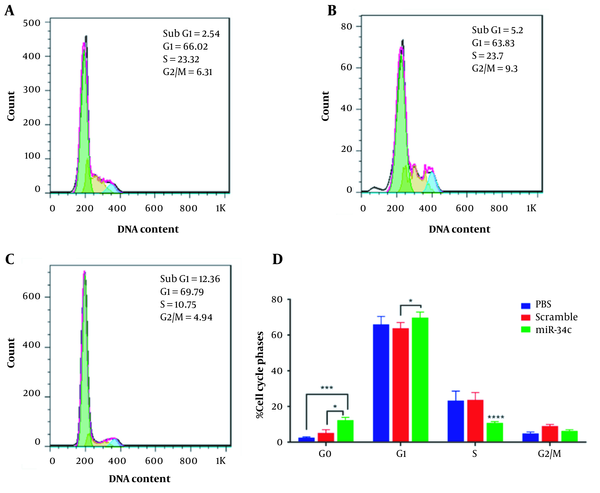
The inhibition zone diameter was observed after treatment with plant methanol extracts at a concentration of 100 mg/mL against L. monocytogenes. ** Significant at 0.01 level of probability.
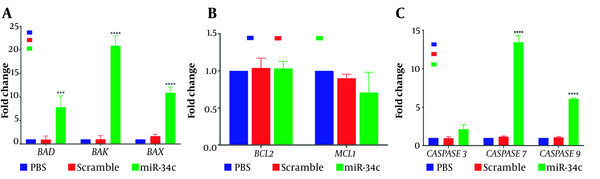
5. Discussion
In this research, F. subpinnata plant extract had the largest diameter of inhibition zone against S. dysenteriae. The extracts of E. caucasicum and F. subpinnata delivered the largest diameters of inhibition zone against Xanthomonas translucens, and M. sylvestris plant extract revealed the largest inhibition zone diameter against E. coli. Finally, E. caucasicum and U. dioica plant extracts showed the largest diameter of inhibition zone against L. monocytogenes.
In the study of Sengul et al., the inhibition zone diameters observed for the methanolic extract of S. officinalis against Bacillus subtilis, Staphylococcus aureus, Streptococcus pyogenes, and Salmonella typhimurium were equal to 10, 18, 0, and 12 mm, respectively (29). In a study by Veda et al., ethyl acetate extract of S. officinalis delivered the inhibition zone diameters of 12.67 ± 0.33, 14, 12.3 ± 0.33, 16, and 13.67 ± 0.33 mm against L. monocytogenes, Staphylococcus aureus, Streptococcus pneumoniae, E. coli, and Pseudomonas aeruginosa, while the inhibition zone diameters of the methanolic extract of this plant against these bacteria were obtained as 13.33 ± 0.33, 14.23 ± 0.33, 16, 19.67 ± 0.33, and 17.67 ± 0.33 mm, respectively (30). In another study, Nabinejad, who investigated the antimicrobial activity of S. officinalis extract against E. coli, reported that the inhibition zone diameters in the dilutions of 10, 15, and 20 microliters were equal to 16, 18, and 19 mm, respectively (31).
In the study of Choi et al., who investigated the in vitro and in vivo antimicrobial activity of F. subpinnata extract against 16 Salmonella samples, the results showed that the inhibitory concentration range was between 62.5 and 1000x03 BCg mL-1. In addition, F. subpinnata extract inhibited Salmonella Typhimurium infection in mice (32). In the study of Jam et al., the antimicrobial and anti-biofilm activities of F. subpinnata L. and Areca nut were investigated against several Gram-positive and Gram-negative resistant bacteria, including Staphylococcus aureus, E. coli, Salmonella, and Enterobacter aeruginosa. The results showed that the antimicrobial and anti-biofilm activities of the plant extracts were dose-dependent, showing strong growth inhibitory effects against S. aureus (98.98%), E. coli (94.98%), and E. aeruginosa (88.55%) (33). Niknejad and Asgharian, who assessed the antibacterial and antioxidant effects of U. dioica leaf essential oil harvested from Tonekabon, Iran, reported that U. dioica leaf essential oil had acceptable antibacterial effects on Gram-positive and Gram-negative bacteria except for Bacillus subtilis, where IC50 and AAI were 21.53 and 2.116 μg/mL, respectively. The main chemical compounds of the extract were found to be phytol (35%), nonanal (3.15%), b-Ionone (0.9%), Phtaleic acid (4.7%), and Carvacrol (5.3%) (34).
In the study of Hashemi et al., the antimicrobial and healing effects of different extracts of U. dioica, as well as burdock and Nasturtium with Silver Sulfadiazine, were investigated on burn infections caused by S. aureus in mice (35). The results showed that in this animal model, the aqueous and ethanolic extracts of U. dioica and oleander and burdock root extracts had antimicrobial activity against the growth of S. aureus, suggesting potent bactericidal effects for the aqueous and ethanolic extracts of U. dioica, Nasturtium, and burdock (36). In the study of Roshani et al., they investigated the antibacterial effects of the acetonic and methanolic extracts of U. dioica and Shirazi thyme against P. aeruginosa expressing metallo-beta-lactamase genes (bla IMP and bla VIM), 83 strains of which were imipenem-resistant while 48 strains showed the CDDT (imipenem-imipenem + EDTA) metallo-beta-lactamase positive phenotype. Among these strains, six expressed the bla IMP gene, but none of them were positive for the bla VIM gene. The results of the recent study showed that the methanolic and acetonic extracts of U. dioica and Shirazi thyme were effective on IMP-producing P. aeruginosa strains (37).
Oxidation in the human body damages cell membranes and other structures, including cellular proteins, lipids, and DNA. When oxygen is metabolized, it creates unstable molecules called free radicals, which steal electrons from other molecules. This causes damage to the structure of DNA and other cellular components. The body can neutralize some free radicals and even needs them to function effectively, but the damage caused by the excess of free radicals over time may be irreparable, leading to certain diseases, including heart and liver diseases and some cancers such as mouth, esophagus, stomach, and intestinal cancers (38).
Sources of antioxidants can be natural or synthetic. Some plants are thought to be rich in antioxidants, which comprise a type of plant nutrients. The body obtains antioxidants from either endogenous or exogenous sources. The antioxidants that enter the body from the outside are called exogenous (39). Antioxidants, sometimes called "free radical scavengers," are essential for preventing the damage caused by free radicals or unstable molecules produced in response to environmental stress and other insults (40).
5.1. Conclusions
The results of this study showed that the methanolic extract of E. caucasicum was the most potent plant extract in inhibiting the growth of some bacteria, including S. dysenteriae, R. tritici, and L. monocytogenes. The growth of E. coli, however, was most effectively inhibited by M. sylvestris extract, suggesting this plant extract is a potential treatment for infections caused by this organism.
Acknowledgements
References
-
1.
Rudan I. A cascade of causes that led to the COVID-19 tragedy in Italy and in other European Union countries. J Glob Health. 2020;10(1):10335. [PubMed ID: 32257150]. [PubMed Central ID: PMC7125421]. https://doi.org/10.7189/jogh.10.010335.
-
2.
Zerbo A, Delgado RC, González PA. Vulnerability and everyday health risks of urban informal settlements in Sub-Saharan Africa. Global Health J. 2020;4(2):46-50. https://doi.org/10.1016/j.glohj.2020.04.003.
-
3.
Mohammadi M, Omidi A, Sabati H. Current trends and new methods of detection of SARS-CoV-2 infection. Cell Mol Biomed Rep. 2022;2(3):138-50. https://doi.org/10.55705/cmbr.2022.345025.1047.
-
4.
Alhashimi RA, Mirzaei A, Alsaedy H. Molecular and clinical analysis of genes involved in gastric cancer. Cell Mol Biomed Rep. 2021;1(3):138-46. https://doi.org/10.55705/cmbr.2021.355860.1056.
-
5.
Kumar M, Sarma DK, Shubham S, Kumawat M, Verma V, Nina PB, et al. Futuristic non-antibiotic therapies to combat antibiotic resistance: A review. Front Microbiol. 2021;12:609459. [PubMed ID: 33574807]. [PubMed Central ID: PMC7870489]. https://doi.org/10.3389/fmicb.2021.609459.
-
6.
Zachariah OH, Lizzy MA, Rose K, Angela MM. Multiple drug resistance of campylobacter jejuni and shigella isolated from diarrhoeic children at kapsabet county referral hospital, kenya. BMC Infect Dis. 2021;21(1):109. [PubMed ID: 33485326]. [PubMed Central ID: PMC7825205]. https://doi.org/10.1186/s12879-021-05788-3.
-
7.
Ahmadi S. Antibacterial and antifungal activities of medicinal plant species and endophytes. Cell Mol Biomed Rep. 2022;2(2):109-15. https://doi.org/10.55705/cmbr.2022.340532.1042.
-
8.
Dey N, Kamatchi C, Vickram AS, Anbarasu K, Thanigaivel S, Palanivelu J, et al. Role of nanomaterials in deactivating multiple drug resistance efflux pumps - A review. Environ Res. 2022;204(Pt A):111968. [PubMed ID: 34453898]. https://doi.org/10.1016/j.envres.2021.111968.
-
9.
Mohammadi M, Sabati H. When successive viral mutations prevent definitive treatment of COVID-19. Cell Mol Biomed Rep. 2022;2(2):98-108. https://doi.org/10.55705/cmbr.2022.339012.1040.
-
10.
Muhammad I, Abubakar B, Muhammad T. Genetic resistance to human malaria. Cell Mol Biomed Rep. 2022;2(2):116-28. https://doi.org/10.55705/cmbr.2022.342533.1043.
-
11.
Slobodianiuk L, Budniak L, Marchyshyn S, Kostyshyn L, Zakharchuk O. Analysis of carbohydrates in Saponaria officinalis L. using GC/MS method. Pharmacia. 2021;68(2):339-45. https://doi.org/10.3897/pharmacia.68.e62691.
-
12.
Tanase MA, Marinescu M, Oancea P, Raducan A, Mihaescu CI, Alexandrescu E, et al. Antibacterial and photocatalytic properties of zno nanoparticles obtained from chemical versus saponaria officinalis extract-mediated synthesis. Molecules. 2021;26(7). [PubMed ID: 33916520]. [PubMed Central ID: PMC8038507]. https://doi.org/10.3390/molecules26072072.
-
13.
Mousavi SM, Hashemi SA, Behbudi G, Mazraedoost S, Omidifar N, Gholami A, et al. A review on health benefits of malva sylvestris l. Nutritional compounds for metabolites, antioxidants, and anti-inflammatory, anticancer, and antimicrobial applications. Evid Based Complement Alternat Med. 2021;2021:5548404. [PubMed ID: 34434245]. [PubMed Central ID: PMC8382527]. https://doi.org/10.1155/2021/5548404.
-
14.
Naghi Tehrani MEH, Ghahremani P, Ramezanzadeh M, Bahlakeh G, Ramezanzadeh B. Theoretical and experimental assessment of a green corrosion inhibitor extracted from Malva sylvestris. J Environ Chem Eng. 2021;9(3). https://doi.org/10.1016/j.jece.2021.105256.
-
15.
Irfan A, Imran M, Khalid M, Sami Ullah M, Khalid N, Assiri MA, et al. Phenolic and flavonoid contents in Malva sylvestris and exploration of active drugs as antioxidant and anti-COVID19 by quantum chemical and molecular docking studies. J Saudi Chem Soc. 2021;25(8). https://doi.org/10.1016/j.jscs.2021.101277.
-
16.
Farhadi N, Meshkini S, Mehdizadeh T. Effect of edible chitosan coating containing Froriepia subpinnata extract on shelf life of Nile tilapia (Oreochromis niloticus) fillet at refrigerated temperature. Res Innov Food Sci Technol. 2022;11(1):95-108.
-
17.
Bahrami A, Jamzad M, Sedaghat S. Phytochemicals and biological activities of froriepia subpinnata (ledeb.) baill. Extracts. J medicinal plants by- products. 2021;10(1):109-15.
-
18.
Azizkhani M, Sodanlo A. Antioxidant activity of Eryngium campestre L., Froriepia subpinnata, and Mentha spicata L. polyphenolic extracts nanocapsulated in chitosan and maltodextrin. J Food Process Preserv. 2020;45(2). https://doi.org/10.1111/jfpp.15120.
-
19.
Dhouibi R, Affes H, Ben Salem M, Hammami S, Sahnoun Z, Zeghal KM, et al. Screening of pharmacological uses of Urtica dioica and others benefits. Prog Biophys Mol Biol. 2020;150:67-77. [PubMed ID: 31163183]. https://doi.org/10.1016/j.pbiomolbio.2019.05.008.
-
20.
Taheri Y, Quispe C, Herrera-Bravo J, Sharifi-Rad J, Ezzat SM, Merghany RM, et al. Urtica dioica-derived phytochemicals for pharmacological and therapeutic applications. Evid Based Complement Alternat Med. 2022;2022:4024331. [PubMed ID: 35251206]. [PubMed Central ID: PMC8894011]. https://doi.org/10.1155/2022/4024331.
-
21.
Fazeli Nasab B, Sirousmehr A, Mirzaei N, Solimani M. [Evaluation of total phenolic, flavenoeid content and antioxidant activity of Leaf and Fruit in 14 different genotypes of Ziziphus mauritiana L. in south of Iran]. Eco-Phytochemical J Med Plants. 2017;4(4):1-14. Persian.
-
22.
abdolmaleki A, feredoni M, Farhadi B, Askari A. [Analgesic and Anti-Inflammatory Effects of Hydroalcoholic Extract of Salvia multicaulis on Male Rats]. Q Horizon of Med Sci. 2015;21(2):121-8. Persian. https://doi.org/10.18869/acadpub.hms.21.2.121.
-
23.
Valizadeh M, Beigomi M, Fazeli-Nasab B. Antibacterial and Anti biofilm effects of ethanol and aceton leaf extract of Momordica charantia and Tecomella undulata against Acinetobacter baumannii. Int J Adv Biol Biomed Res. 2020;8(4):418-3. https://doi.org/10.33945/sami/ijabbr.2020.4.6.
-
24.
Ochieng Nyalo P, Isanda Omwenga G, Piero Ngugi M. GC-MS analysis, antibacterial and antioxidant potential of ethyl acetate leaf extract of senna singueana (delile) grown in Kenya. Evid Based Complement Alternat Med. 2022;2022:5436476. [PubMed ID: 36034966]. [PubMed Central ID: PMC9410794]. https://doi.org/10.1155/2022/5436476.
-
25.
Aubais aljelehawy QH, Hadi Alshaibah LH, Abbas Al- Khafaji ZK. Evaluation of virulence factors among Staphylococcus aureus strains isolated from patients with urinary tract infection in Al-Najaf Al-Ashraf teaching hospital. Cell Mol Biomed. 2021;1(2):78-87. https://doi.org/10.55705/cmbr.2021.144995.1017.
-
26.
Assali M, Almasri M, Kittana N, Alsouqi D. Covalent functionalization of graphene sheets with different moieties and their effects on biological activities. ACS Biomater Sci Eng. 2020;6(1):112-21. [PubMed ID: 33463246]. https://doi.org/10.1021/acsbiomaterials.9b01143.
-
27.
Nwosu LC, Edo GI, Özgör E. The phytochemical, proximate, pharmacological, gc-ms analysis of cyperus esculentus (tiger nut): A fully validated approach in health, food and nutrition. Food Bioscience. 2022;46. https://doi.org/10.1016/j.fbio.2022.101551.
-
28.
Dalli M, Azizi SE, Benouda H, Azghar A, Tahri M, Bouammali B, et al. Molecular composition and antibacterial effect of five essential oils extracted from nigella sativa l. Seeds against multidrug-resistant bacteria: A comparative study. Evid Based Complement Alternat Med. 2021;2021:6643765. [PubMed ID: 33790979]. [PubMed Central ID: PMC7984878]. https://doi.org/10.1155/2021/6643765.
-
29.
Sengul M, Ercisli S, Yildiz H, Gungor N, Kavaz A, Cetin B. Antioxidant, antimicrobial activity and total phenolic content within the aerial parts of artemisia absinthum, artemisia santonicum and saponaria officinalis. Iran J Pharm Res. 2011;10(1):49-56. [PubMed ID: 24363680]. [PubMed Central ID: PMC3869595].
-
30.
Veda G, Talluri MR, Ganga B. Antibacterial activity of Saponaria officinalis and Zanthophyllum aramatum. J Pharmacol Toxicol. 2016;5(1). https://doi.org/10.14419/ijpt.v5i1.6898.
-
31.
Nabinejad A. Antibacterial effects of Saponaria officinalis extracts against avian pathogenic Escherichia coli (APEC). Afr J Agric Res. 2013;8(18):2068-71. https://doi.org/10.5897/ajar11.1390.
-
32.
Choi JG, Kang OH, Lee YS, Chae HS, Oh YC, Brice OO, et al. In Vitro and In Vivo antibacterial activity of Punica granatum peel ethanol extract against Salmonella. Evid Based Complement Alternat Med. 2011;2011:690518. [PubMed ID: 19687188]. [PubMed Central ID: PMC3137154]. https://doi.org/10.1093/ecam/nep105.
-
33.
Jam N, Hajimohammadi R, Gharbani P, Mehrizad A. Antibacterial activity of Punica granatum L. and Areca nut (P.A) combined extracts against some food born pathogenic bacteria. Saudi J Biol Sci. 2022;29(3):1730-6. [PubMed ID: 35280537]. [PubMed Central ID: PMC8913389]. https://doi.org/10.1016/j.sjbs.2021.10.057.
-
34.
Niknejad H, Asgharian A. [Determination of antibacterial and antioxidant activity and identifying chemical compounds of Urtica dioica L. leaf essence as a potent antibacterial agent]. Pajoohandeh J. 2015;20(4):234-9. Persian.
-
35.
Hashemi AH, Shapouri R, Esrafili MR. [Antimicrobial and healing effectburdock and Nasturtium of nettle, extracts with silver sulfadiazine on burn infections of Staphylococcus aurous]. Quarterly J Animal Physiol Develope. 2019;12(2). Persian.
-
36.
Lawrence RM, Lawrence RA. Medications, Herbal preparations, and natural products in breast milk. Breastfeeding. 2022. p. 326-92. https://doi.org/10.1016/b978-0-323-68013-4.00011-0.
-
37.
Roshani M, Heidary M, Goudarzi H, Hashemi A, Eslami G, Yousefi N. [Investigating the Antibacterial Effect of Methanoland Acetone Extracts of Urtica Dioica and Zataria Multifloraagainst Metallo Beta-lactamase Producing Pseudomonas aeruDecember 5, 2015 ginosa]. J Ilam Univ Med Sci. 2016;24(3):70-8. Persian. https://doi.org/10.18869/acadpub.sjimu.24.3.70.
-
38.
Ribeiro JS, Santos M, Silva LKR, Pereira LCL, Santos IA, da Silva Lannes SC, et al. Natural antioxidants used in meat products: A brief review. Meat Sci. 2019;148:181-8. [PubMed ID: 30389412]. https://doi.org/10.1016/j.meatsci.2018.10.016.
-
39.
Atta EM, Mohamed NH, Abdelgawad AA. Antioxidants: An overview on the natural and synthetic types. European Chemical Bulletin. 2017;6(8). https://doi.org/10.17628/ecb.2017.6.365-375.
-
40.
McClements DJ, Decker E. Interfacial antioxidants: A review of natural and synthetic emulsifiers and coemulsifiers that can inhibit lipid oxidation. J Agric Food Chem. 2018;66(1):20-35. [PubMed ID: 29227097]. https://doi.org/10.1021/acs.jafc.7b05066.