Abstract
Background:
Chrysin is a natural flavonoid with several demonstrated neuro-pharmacological effects in brain areas related to anxiety. However, the intra-hypothalamic molecular mechanisms underlying the anxiolytic effects of chrysin remain unclear.Objectives:
The current study revealed the effects of chrysin on hypothalamic corticotrophin-releasing hormone (CRH) and calcitonin gene-related peptide (CGRP) gene expression levels in a rat model of stress.Methods:
Thirty male Wistar rats weighing 200 ± 10 g were divided into six groups for this investigation. Acute restraint stress was induced in the animals for 2 hours. Intact or stress-induced rats received 20 or 40 µg of chrysin via the third cerebral ventricle, respectively. Open-field and forced swimming tests were performed to evaluate stress-related behaviors. Hypothalamic samples were then removed, and real-time polymerase chain reaction (PCR) was used to measure relative gene expression.Results:
The results showed that in the rats receiving chrysin, CRH and CGRP gene expression levels were significantly decreased compared to the stress group. Additionally, chrysin injection reduced anxiogenic behaviors.Conclusions:
Chrysin decreased the expression of hypothalamic CRH and CGRP genes in stressed rats.Keywords
1. Background
Stress is a complex condition that contributes to the pathogenesis of several neuropsychiatric, neuroendocrine, neurological, and metabolic disorders. Anxiety is one of the most prevalent factors involved in the disturbance of various hormonal and peptidergic signaling pathways in the brain and peripheral organs (1).
Corticotrophin-releasing hormone (CRH) is synthesized in the paraventricular nucleus (PVN) of the hypothalamus and consists of 42 amino acids. It is associated with pain, reproduction, energy balance, anxiety, and almost all other diseases. Corticotrophin-releasing hormone is the most crucial intra-hypothalamic regulator of the hypothalamic–pituitary–adrenal (HPA) axis in response to stress. Acute or chronic stress significantly activates the HPA axis, and disturbance of the CRH signaling pathway results in dysfunction of neuroendocrine, neurological, and behavioral responses (2).
Calcitonin gene-related peptide (CGRP) is a neuropeptide consisting of 37 amino acids. Calcitonin gene-related peptide is synthesized throughout the central nervous system. In addition to the spinal cord, CGRP is expressed in various hypothalamic nuclei in both males and females, including the arcuate nucleus, lateral hypothalamus, and medial basal hypothalamus (3). It has been found that the central injection of CGRP activates brain areas related to stress, while antagonism of CGRP receptors suppresses anxiogenic behaviors (4, 5).
Chrysin, a natural polyphenol from the flavonoid group, is a bioactive constituent of honey, propolis, and several herbal medicinal herbs such as Passiflora incarnata, Passiflora coerulea, and Oroxylum indicum (6). Previous studies have demonstrated that chrysin has anticancer, neuroprotective, anti-inflammatory, anxiolytic, and pain-relieving effects (6, 7). Chrysin suppresses anxiety-like behaviors in rats by affecting the action of GABAA receptors (8). The contribution of neurotransmitter systems, such as dopamine (DP), serotonin (5HT), gamma-aminobutyric acid (GABA), and norepinephrine, has been established for the anti-anxiety effects of chrysin. The neuro-pharmacological influences of chrysin have been demonstrated in brain areas including the amygdala, hippocampus, and prefrontal cortex, which are associated with anxiety (8). However, there is no information about the intra-hypothalamic molecular mechanisms related to the anxiolytic effects of chrysin on CRH neural circuits.
2. Objectives
The present study investigated the changes in hypothalamic CRH and CGRP gene expression in a rat model of stress treated with chrysin.
3. Methods
3.1. Animals
Adult male rats used in this study weighed 200 ± 10 g. The animals were housed in the laboratory for two weeks with easy access to water and food. The temperature was maintained at 23 ± 2ºC, with a 12-hour light/dark cycle.
3.2. Experimental Protocol
Thirty male Wistar rats were classified into six groups (n = 5 each). Group I was the control group, which received saline. Groups II and III consisted of intact rats receiving 20 or 40 µg chrysin (9). Group IV was the stress control group, which received saline. Groups V and VI included stress-induced rats receiving 20 or 40 µg chrysin (Cas No. 480-40-0, Co, USA). All injections were administered 30 minutes before stress induction via intracerebroventricular (ICV) injection in a volume of 3 µL. Behavioral tests were performed 2 hours after the rats were exposed to stress.
3.3. Hypothalamic Sample Dissection
First, the animals were euthanized. The skull was then opened to remove the brain. The brain was positioned with the ventral surface facing up, and a 4 mm thick slice containing the hypothalamus was dissected (anteriorly from the optic chiasma, posteriorly to the vicinity of the mammillothalamic system, and laterally to the hypothalamic sulcus).
3.4. Surgical Procedure
For the ICV injection, the animals were first placed under deep anesthesia using ketamine (80 mg/kg) and xylazine (10 mg/kg). The animal's head was then fixed in a stereotactic apparatus. A cannula was implanted in the skull based on the coordinates from the Paxinos and Watson Atlas (AP = 0.84 mm, ML = 0, DV = 6.5 mm) (10). The animals were kept in the laboratory for a one-week recovery period. The injection was performed with a Hamilton syringe attached to a polyethylene tube (PE20).
3.5. Acute Restraint Stress and Drug Administration
After the one-week recovery period, the rats were placed in a well-ventilated plastic tube (18 cm long and 5 cm wide) for acute restraint stress. They were kept in this tube for 2 hours in a quiet room. Chrysin was injected into the rats 30 minutes before the induction of stress (11).
3.6. Behavioral Tests
3.6.1. Open Field Test
For stress assessment, the rats were placed in a square plastic canister (60 cm L × 60 cm W × 40 cm H) with a central zone (30 cm × 30 cm) (12). At the start of the experiment, each rat was gently placed in the center of the canister. The animal was allowed to explore the box freely for 5 minutes. During this time, the animal's behavior was recorded with a camera. The time spent in the center and the number of entries into the center were assessed.
3.6.2. Forced Swimming Test
The rats were placed in a transparent plastic barrel (35 cm in diameter, 50 cm in height) filled with water up to 30 cm, with their tails slowly submerged in the water (13). All the animals were allowed to swim freely. The water temperature was maintained at 24 ± 1°C. During the experiment, the animals' behavior was recorded with a camera. The duration of immobility was assessed over 6 minutes (14). Immobility was defined as the animal remaining still in the water, while swimming was considered an active movement of the limbs.
3.7. Real-time Polymerase Chain Reaction (RT-PCR)
Total RNA was extracted from the hypothalamic samples using the TRIzol reagent according to the kit instructions. RNA concentration was determined using a NanoDrop device. According to the kit instructions, 1 μL of total RNA was reverse transcribed to cDNA in a total volume of 20 μL (Biotech Rabbit, Germany). Gene amplification (final volume 25 μL) was performed using a PCR device and SYBR Green I according to the manufacturer's kit (Takara Bio Inc., Japan).
The RT-PCR cycle was defined as follows: One cycle at 95°C for 15 minutes, followed by 40 cycles including denaturation at 95°C for 20 seconds, annealing at 60°C for 15 seconds, and extension at 72°C for 10 seconds. The sequences used for forward and reverse primers are listed in Table 1.
Sequence of Sense and Antisense Primers
Variables | Primers Sequences |
---|---|
Corticotrophin-releasing hormone(CRH): Sense antisense | 5'- TGGATCTCACCTTCCACCTTCTG -3' |
5'- CCGATAATCTCCATCAGTTTCCTG -3' | |
Calcitonin gene-related peptide (CGRP): Sense antisense | 5'- TCTAAGCGGTGTGGGAATCT -3' |
5'- TAGGGGTGGTGGTTTGTCTC -3' | |
GAPDH: Sense antisense | 5′- AAGTTCAACGGCACAGTCAAG -3′ |
5′- CATACTCAGCACCAGCATCAC -3′. |
The CRH, CGRP, and GAPDH amplified products were 103, 155, and 120 base pairs respectively. The 2-ΔΔCT equation was used to calculate gene expression fold change.
3.8. Statistical Analysis
SPSS software, version 16, was used to conduct the statistical analysis. The values were expressed as mean ± SEM. Tukey's post hoc test was used after one-way ANOVA analysis to compare the values between the experimental groups. The level of significance was set at P ≤ 0.05.
4. Results
The results of the forced swimming test showed that rats exposed to stress were immobile for a significantly longer time than the control group. Both dosages of chrysin (20 and 40 µg) significantly decreased the immobility time compared to the stress group (Figure 1 P ≤ 0.05). Injection of chrysin at dosages of 20 or 40 µg into intact rats also significantly reduced immobility time compared to the control (P ≤ 0.05).
Effects of chrysin on immobility time in the forced swim test. The values are expressed as mean ± SEM and P-value < 0.05 gives a significant difference. *compared to control; &compared to stress group.
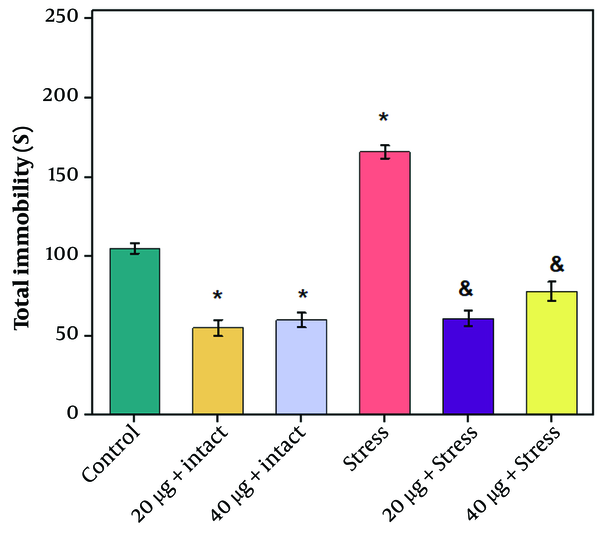
The results of the open field test showed a significant decrease in the number of central crossings and the time spent in the center in the stress group compared to the control group (P ≤ 0.05). Chrysin at dosages of 20 or 40 µg increased these factors compared to the stress group; however, this increase was statistically significant only in rats receiving the 20 µg dosage of chrysin (Figure 2 A and B, P ≤ 0.05). Additionally, there was a significant decrease in the number of central crossings and the time spent in the center in rats receiving 40 µg chrysin compared to those receiving 20 µg. The number of entries into the center and the time spent in the center in the intact group receiving chrysin (20 and 40 µg) decreased compared to the control group, which was significant only at the 40 µg dose (P ≤ 0.05).
A, Effects of chrysin on the time spent in center; and B, the number of entries into the center in open field test. The values are expressed as mean ± SEM and P-value < 0.05 gives a significant difference. *compared to control; &compared to stress group, #compared to 20µg chrysin group.
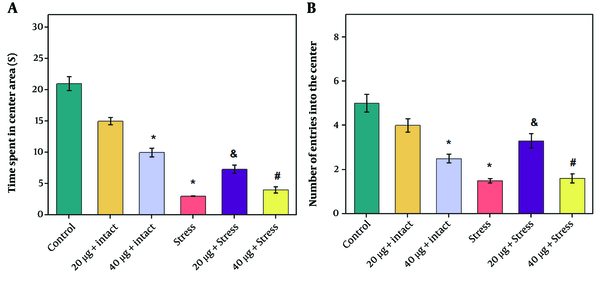
Hypothalamic CRH and CGRP gene expression levels in the rats receiving 20 or 40 µg of chrysin significantly declined compared to the control group (Figure 3 P ≤ 0.05). Induction of stress caused a significant increase in hypothalamic CRH and CGRP gene expression levels compared to the control group (Figure 4 P ≤ 0.05). The third cerebral ventricular injection of chrysin at both doses (20 and 40 µg) significantly decreased hypothalamic CRH and CGRP gene expression levels compared to the stress group (Figure 4 P ≤ 0.05). However, no significant difference was observed between the effects of 40 µg chrysin on CRH and CGRP gene expression levels compared to rats receiving 20 µg (Figure 4).
Effects of chrysin on the hypothalamic corticotrophin-releasing hormone (CRH) and calcitonin gene-related peptide (CGRP) mRNA levels in intact rats. The values are expressed as mean ± SEM and P-value < 0.05 gives a significant difference. *compared to control.
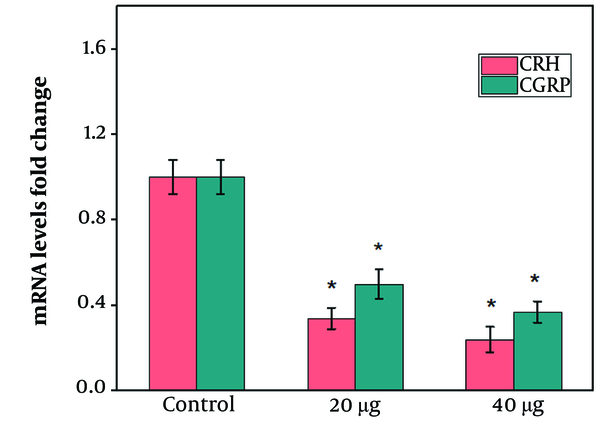
Effects of chrysin on the hypothalamic corticotrophin-releasing hormone (CRH) and calcitonin gene-related peptide (CGRP) mRNA levels in a rat model of stress. The values are expressed as mean ± SEM and P-value < 0.05 gives a significant difference. *compared to control; &compared to stress group.
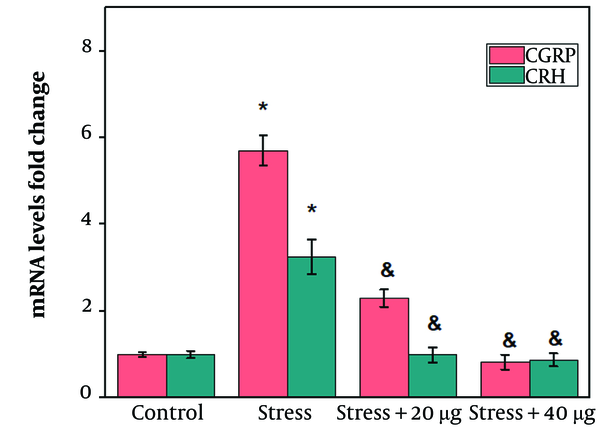
5. Discussion
The results revealed the stimulatory effects of stress on hypothalamic CRH gene expression levels. These findings are consistent with those of previous studies, which indicated that exposure to psychological or physical stressors activates the HPA axis to manage the anxiogenic-induced need (2, 15, 16). Intracerebroventricular injections of chrysin significantly inhibited CRH gene expression levels compared to the stress group. Based on previous anxiolytic behavioral studies, some neurotransmitter systems may be involved in mediating the inhibitory effects of chrysin on CRH gene expression levels (17).
Behavioral studies have established that the anxiolytic effect of chrysin is similar to that of diazepam, but chrysin does not present any motor effects related to sedation. Therefore, these studies suggest that chrysin may be more suitable than benzodiazepines for the treatment of anxiety disorders. As previously reported, the central GABAergic system is crucial for managing stress by controlling the HPA axis. GABAergic neurons innervate nearly half of the CRH neurons in the PVN of the hypothalamus, and GABA exerts inhibitory effects on CRH neuron function, especially via GABAA receptors (18, 19).
Chrysin can bind to the central and peripheral benzodiazepine-binding sites of the GABAA receptor and stimulate the activity of the GABAergic system. The antagonists of the benzodiazepine binding site of the GABAA receptor completely suppress chrysin's anxiolytic effect (8). Therefore, activating GABAergic systems upstream of CRH neurons may be a possible mechanism for chrysin to reduce hypothalamic CRH gene expression levels in rat stress models.
In addition to monoamine systems, several hypothalamic neuropeptides regulate the HPA axis in response to stress by either inhibiting or stimulating CRH neurons. However, there is no study on the hypothalamic molecular mechanisms involved in the downregulation of CRH by chrysin. To explore potential mechanisms upstream of the CRH neurons, the current research was conducted to evaluate the effects of chrysin on hypothalamic CGRP gene expression levels in a rat stress model. The results indicated that stress induction significantly increased CGRP gene expression levels. As previously demonstrated, the inhibition of the CGRP receptor may be a therapeutic target in the management of anxiety disorders (19).
Calcitonin gene-related peptide neurons are highly stress-responsive and stimulate the HPA axis, norepinephrine, and glutamate release, which are directly related to the induction of stress responses (4, 18). On the other hand, GABAergic neurons suppress the activity of CGRP neurons (20). The ICV injection of chrysin significantly decreased hypothalamic CGRP gene expression levels in the rat stress model. The CGRP projection from brain areas related to anxiety neural circuitry increases CRH release (4). Therefore, the downregulation of hypothalamic CRH by chrysin may be due to its GABAergic activity, which may contribute to the decrease in CGRP gene expression levels.
The only limitation of this manuscript was insufficient funding for further experiments.
5.1. Conclusions
In conclusion, based on the results of the forced swimming and open field tests, the ICV injection of chrysin improved acute restraint stress-induced anxiety-like behaviors. The intra-hypothalamic molecular mechanisms underlying the anxiolytic effects of chrysin may partly involve decreasing CRH and CGRP gene expression levels. Chrysin may be a potential therapeutic target for anxiety-related disorders.
Acknowledgements
References
-
1.
Kupcova I, Danisovic L, Grgac I, Harsanyi S. Anxiety and Depression: What Do We Know of Neuropeptides? Behav Sci (Basel). 2022;12(8). [PubMed ID: 36004833]. [PubMed Central ID: PMC9405013]. https://doi.org/10.3390/bs12080262.
-
2.
Mamalaki E, Kvetnansky R, Brady LS, Gold PW, Herkenham M. Repeated immobilization stress alters tyrosine hydroxylase, corticotropin-releasing hormone and corticosteroid receptor messenger ribonucleic Acid levels in rat brain. J Neuroendocrinol. 1992;4(6):689-99. [PubMed ID: 21554656]. https://doi.org/10.1111/j.1365-2826.1992.tb00220.x.
-
3.
Iyengar S, Ossipov MH, Johnson KW. The role of calcitonin gene-related peptide in peripheral and central pain mechanisms including migraine. Pain. 2017;158(4):543-59. [PubMed ID: 28301400]. [PubMed Central ID: PMC5359791]. https://doi.org/10.1097/j.pain.0000000000000831.
-
4.
Sink KS, Walker DL, Yang Y, Davis M. Calcitonin gene-related peptide in the bed nucleus of the stria terminalis produces an anxiety-like pattern of behavior and increases neural activation in anxiety-related structures. J Neurosci. 2011;31(5):1802-10. [PubMed ID: 21289190]. [PubMed Central ID: PMC3088995]. https://doi.org/10.1523/JNEUROSCI.5274-10.2011.
-
5.
Rahman SM, Hauser C, Faucher S, Fine E, Luebke AE. Both systemic calcitonin gene related peptide (CGRP) and a vestibular challenge promote anxiety-related behaviours and dynamic imbalance in mice. bioRxiv. 2023;Preprint. https://doi.org/10.1101/2023.06.30.547257.
-
6.
Talebi M, Talebi M, Farkhondeh T, Kopustinskiene DM, Simal-Gandara J, Bernatoniene J, et al. An updated review on the versatile role of chrysin in neurological diseases: Chemistry, pharmacology, and drug delivery approaches. Biomed Pharmacother. 2021;141:111906. [PubMed ID: 34328092]. https://doi.org/10.1016/j.biopha.2021.111906.
-
7.
Stompor-Goracy M, Bajek-Bil A, Machaczka M. Chrysin: Perspectives on Contemporary Status and Future Possibilities as Pro-Health Agent. Nutrients. 2021;13(6). [PubMed ID: 34198618]. [PubMed Central ID: PMC8232110]. https://doi.org/10.3390/nu13062038.
-
8.
Rodriguez-Landa JF, Guillen-Ruiz G, Hernandez-Lopez F, Cueto-Escobedo J, Rivadeneyra-Dominguez E, Bernal-Morales B, et al. Chrysin reduces anxiety-like behavior through actions on GABA(A) receptors during metestrus-diestrus in the rat. Behav Brain Res. 2021;397:112952. [PubMed ID: 33017640]. https://doi.org/10.1016/j.bbr.2020.112952.
-
9.
Medina JH, Paladini AC, Wolfman C, Levi de Stein M, Calvo D, Diaz LE, et al. Chrysin (5,7-di-OH-flavone), a naturally-occurring ligand for benzodiazepine receptors, with anticonvulsant properties. Biochem Pharmacol. 1990;40(10):2227-31. [PubMed ID: 2173925]. https://doi.org/10.1016/0006-2952(90)90716-x.
-
10.
Paxinos G, Watson C. The Rat Brain in Stereotaxic Coordinates. Amsterdam, Netherlands: Elsevier; 2006.
-
11.
Bali A, Jaggi AS. Preclinical experimental stress studies: protocols, assessment and comparison. Eur J Pharmacol. 2015;746:282-92. [PubMed ID: 25446911]. https://doi.org/10.1016/j.ejphar.2014.10.017.
-
12.
Pawlak CR, Schwarting RK. Object preference and nicotine consumption in rats with high vs. low rearing activity in a novel open field. Pharmacol Biochem Behav. 2002;73(3):679-87. [PubMed ID: 12151044]. https://doi.org/10.1016/s0091-3057(02)00852-3.
-
13.
Roque A, Ruiz-Gonzalez R, Pineda-Lopez E, Torner L, Lajud N. Prenatal immobilization stress and postnatal maternal separation cause differential neuroendocrine responses to fasting stress in adult male rats. Dev Psychobiol. 2020;62(6):737-48. [PubMed ID: 31886525]. https://doi.org/10.1002/dev.21947.
-
14.
Fitzgerald PJ, Hale PJ, Ghimire A, Watson BO. The cholinesterase inhibitor donepezil has antidepressant-like properties in the mouse forced swim test. Transl Psychiatry. 2020;10(1):255. [PubMed ID: 32712627]. [PubMed Central ID: PMC7382650]. https://doi.org/10.1038/s41398-020-00928-w.
-
15.
Rajamanickam S, Justice NJ. Hypothalamic corticotropin-releasing factor neurons modulate behavior, endocrine, and autonomic stress responses via direct synaptic projections. Curr Opinion Endocrine Metabolic Res. 2022;26:100400. https://doi.org/10.1016/j.coemr.2022.100400.
-
16.
Kageyama K, Iwasaki Y, Daimon M. Hypothalamic Regulation of Corticotropin-Releasing Factor under Stress and Stress Resilience. Int J Mol Sci. 2021;22(22). [PubMed ID: 34830130]. [PubMed Central ID: PMC8621508]. https://doi.org/10.3390/ijms222212242.
-
17.
Rodriguez-Landa JF, German-Ponciano LJ, Puga-Olguin A, Olmos-Vazquez OJ. Pharmacological, Neurochemical, and Behavioral Mechanisms Underlying the Anxiolytic- and Antidepressant-like Effects of Flavonoid Chrysin. Mol. 2022;27(11). [PubMed ID: 35684488]. [PubMed Central ID: PMC9182416]. https://doi.org/10.3390/molecules27113551.
-
18.
Maguire J. The relationship between GABA and stress: 'it's complicated'. J Physiol. 2018;596(10):1781-2. [PubMed ID: 29524241]. [PubMed Central ID: PMC5978385]. https://doi.org/10.1113/JP275937.
-
19.
Tafet GE, Nemeroff CB. Pharmacological Treatment of Anxiety Disorders: The Role of the HPA Axis. Front Psychiatry. 2020;11:443. [PubMed ID: 32499732]. [PubMed Central ID: PMC7243209]. https://doi.org/10.3389/fpsyt.2020.00443.
-
20.
Bourgoin S, Pohl M, Benoliel JJ, Mauborgne A, Collin E, Hamon M, et al. gamma-Aminobutyric acid, through GABAA receptors, inhibits the potassium-stimulated release of calcitonin gene-related peptide- but not that of substance P-like material from rat spinal cord slices. Brain Res. 1992;583(1-2):344-8. [PubMed ID: 1380400]. https://doi.org/10.1016/s0006-8993(10)80048-4.